Chapter 1 Overview of the Organization of the Nervous System
The human nervous system is the most complex product of biological evolution. The constantly changing patterns of activity of its billions of interactive units represent the fundamental physical basis of every aspect of human behaviour and experience. Many thousands of scientists and clinicians around the world, whether driven by intellectual curiosity or the quest for better methods of disease prevention and treatment, have studied the nervous system over many years. However, our understanding of complex neural organization and function is still quite rudimentary, as is our ability to deal with its many pathologies. Multidisciplinary research into the nervous system is one of the most active areas of contemporary biology and medicine, and rapid advances across a range of fronts bring the realistic prospect of better prevention and treatment of many neurological disorders in the future.
The functional capabilities of the nervous system are a product of its vast population of intercommunicating nerve cells, or neurones, estimated to number on the order of 1010. Neurones encode information, conduct it, sometimes over considerable distances, and then transmit it to other neurones or to non-neural tissues (muscles or glandular cells). Most neurones consist of a central mass of cytoplasm within a limiting cell membrane (the cell body or soma) from which a number of branched processes, termed neurites, extend (Fig. 1.1). One of these, the axon, is usually much longer than the others and normally conducts information away from the cell body. The other processes are termed dendrites, and these typically conduct information toward the soma. The nerve cell membrane is polarized, the inside of the cell being around 70 mV negative with respect to the outside. Information is coded in the form of patterns of transient depolarizations and repolarizations of this membrane potential, known as nerve impulses or action potentials. These are conducted along the axon, which may have collateral branches that permit information to be distributed simultaneously to several targets (Fig. 1.2). Axons possess specialized endings, or axon terminals, that come into close apposition with the membrane of the target cell at synapses, where information passes from one cell to another. Axon terminals may form synaptic contacts with dendrites (axodendritic), cell bodies (axosomatic), other axons (axoaxonic) or non-neural tissue such as muscle cells (neuromuscular junction). Transmission of information to other cells is brought about when action potentials cause the release of specific neurotransmitter substances stored in synaptic vesicles within the presynaptic nerve terminal. Specialized receptors are located on the postsynaptic target cell membrane. The neurotransmitter binds to these and, depending on the nature of the chemical and the receptor, either elicits an excitatory (depolarizing) or inhibitory (hyperpolarizing) response or modulates intracellular second messenger systems.
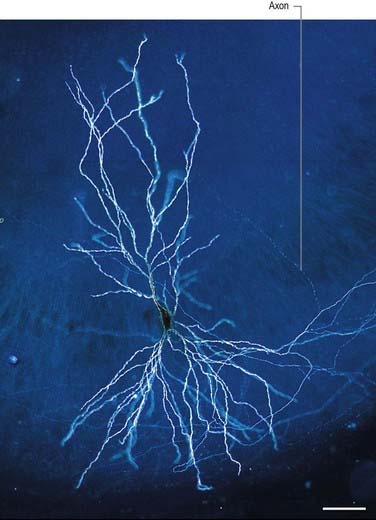
Fig. 1.1 Dark-field illuminated micrograph of a CA3 pyramidal cell in a hippocampal slice culture, intracellularly injected with the dye biocytin. Scale bar 50 µm.
(Courtesy of R. Anne McKinney, McGill University, and Mathias Abegg, Brain Research Institute, University of Zurich.)
The nervous system is customarily divided into two major parts: the central nervous system (CNS) and the peripheral nervous system (PNS). The CNS consists of the brain and spinal cord. The PNS is composed of cranial nerves and spinal nerves together with their ramifications and certain groupings of cell bodies that constitute the peripheral ganglia. Another convention divides the nervous system into somatic and autonomic components. Anatomically, both of these have elements in the CNS and PNS. The autonomic nervous system, which consists of sympathetic and parasympathetic divisions, is made up of neurones concerned primarily with control of the internal environment through innervation of secretory glands and cardiac and smooth muscle. It is considered in detail in Chapter 21. The wall of the gastrointestinal tract contains neurones capable of sustaining local reflex activity independent of the CNS, which are known as the enteric nervous system.
Central Nervous System
The brain and spinal cord (Fig. 1.3) contain the great majority of neuronal cell bodies in the nervous system. In many parts of the CNS the cell bodies of neurones are grouped together and are more or less segregated from axons. The generic term for such collections of cell bodies is grey matter. Smaller aggregations of neuronal cell bodies, which usually share a common functional role, are termed nuclei. It follows that neuronal dendrites and synaptic interactions are mostly confined to grey matter. Axons tend to be grouped together to form white matter, so called because axons are often ensheathed in myelin, which confers a paler colouration. Axons that pass between similar sources or destinations within the CNS tend to run together in defined pathways or tracts. These often cross the midline (decussate), which means that half of the body is, in many respects, controlled by and sends information to the opposite side of the brain.
Some groups of neurones in the spinal cord and brain stem that subserve similar functions are organized into longitudinal columns. The neurones in these columns may be concentrated into discrete, discontinuous nuclei in some areas, such as the cranial nerve nuclei of the brain stem, or they may form more or less continuous longitudinal bands, as in much of the spinal cord (Fig. 1.4). Efferent neurones constitute three such columns. The somatic motor column contains motor neurones, the axons of which serve muscles derived from head somites. The two other columns are related to specialized features of head morphology. Of these, the branchial motor column innervates muscles derived from the wall of the embryonic pharynx (branchial muscles), and the visceral motor column supplies preganglionic parasympathetic fibres to glands and visceral smooth muscle. There are four longitudinal cell columns related to sensory functions. The general somatic sensory column essentially deals with information from the head. Special somatic sensory neurones are related to the special senses and receive vestibular and auditory input. General visceral sensory neurones deal with information from widespread and varied visceral sensory endings, and special visceral sensory neurones are related to the special sense of taste.
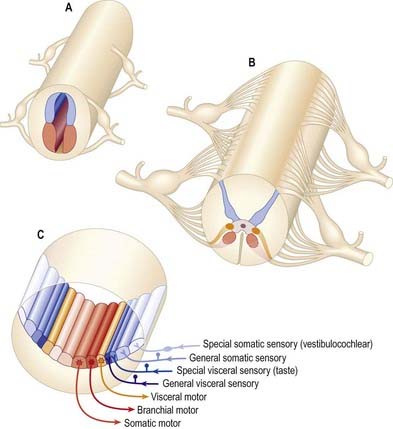
Fig. 1.4 Arrangement of sensory and motor cell columns in the spinal cord and brain stem. A, Organization of the primitive spinal cord with a dorsal sensory column (blue), a ventral column (red) and segmentally arranged dorsal and ventral nerve roots. B, Arrangement of adult spinal cord serving the thorax, with sensory and somatic motor columns colour-coded in the same way as in A, with an additional intermediate (lateral) visceral motor column (orange). C, Arrangement of multiple longitudinal columns in the brain stem, where the motor column is now subdivided into three parts and the sensory column into four. For further information about the embryological aspects of the early nervous system, consult Chapter 3. See also Fig. 10.1.
To sustain the energy required by constant neuronal activity, the CNS has a high metabolic rate and a rich blood supply. The blood–brain barrier controls the neuronal environment and imposes severe restrictions on the types of substances that can pass from the blood stream into nervous tissue.
Spinal Cord
The spinal cord is located within the vertebral column, lying in the upper two-thirds of the vertebral canal (Ch. 8). It is continuous rostrally with the medulla oblongata. For the most part, the spinal cord controls the functions of, and receives afferent input from, the trunk and limbs. Afferent and efferent connections travel in 31 pairs of segmentally arranged spinal nerves. These attach to the cord as dorsal and ventral rootlets that unite to form the spinal nerves proper (Fig. 1.5). The dorsal and ventral roots are functionally distinct. Dorsal roots carry primary afferent nerve fibres from cell bodies located in dorsal root ganglia. Ventral roots carry efferent fibres from cell bodies located in the spinal grey matter.