CHAPTER 15 Overview of the nervous system
The human nervous system is the most complex product of biological evolution. The constantly changing patterns of activity of its billions of interactive units represent the fundamental physical basis of every aspect of human behaviour and experience. Many thousands of scientists and clinicians around the world, whether driven by intellectual curiosity or the quest for better methods of disease prevention and treatment, have studied the nervous system over many years. Despite the enormous amount of data available, our understanding of complex neural organization and function is far from complete, as is our ability to deal with its many pathologies. Nevertheless, research on the nervous system is one of the most active areas of contemporary biology and medicine and rapid advances on numerous fronts bring with them the realistic prospect of better prevention and treatment of many neurological disorders in the future.
The functional capabilities of the nervous system are a product of its vast population of intercommunicating nerve cells or neurones, estimated to number in the order of 1010 (Ch. 3). In brief, neurones encode information, conduct it, sometimes over considerable distances, and then transmit it to other neurones or to non-neural tissues such as muscles or glandular cells. Most neurones consist of a central mass of cytoplasm within a limiting cell membrane, the cell body or soma, from which extend a number of branched processes, or neurites (Fig. 15.1). One of these, the axon, or nerve fibre, is usually much longer than the others and conducts information away from the cell body. The other processes are termed dendrites and these typically conduct information towards the soma (cell body). The nerve cell membrane is polarized, the inside of the cell being around −70 mV negative with respect to the outside. Information is coded in the form of patterns of transient depolarizations and repolarizations of this membrane potential, known as action potentials or nerve impulses. These are conducted along the axon, which may have collateral branches that permit information to be distributed simultaneously to several targets. Axons possess specialized endings, or axon terminals, which come into close apposition with the membrane of the target cell at synapses, where information passes from one cell to another (Fig. 15.2). Axon terminals may form synaptic contacts with dendrites (axodendritic), cell bodies (axosomatic), other axons (axoaxonic) or non-neural tissue such as muscle cells (neuromuscular junction). Transmission of information to other cells occurs when action potentials cause the release of specific neurotransmitter substances, stored in synaptic vesicles within the presynaptic nerve terminal. The neurotransmitter binds to specialized receptors that are located on the postsynaptic target cell membrane and, depending upon the nature of the chemical and the receptor, either elicits an excitatory (depolarizing) or inhibitory (hyperpolarizing) response, or may modulate intracellular second messenger systems.
The huge complexity of the nervous system reflects the fact that individual neurones may make synaptic contact with hundreds, or even thousands, of other neurones via profuse axonal collateralization and dendritic branching (arborization). The latter is exemplified by the extensive dendritic field of the cerebellar Purkinje cell, which is traversed by thousands of axons, making synaptic contact as they pass. At the level of the individual neurone, competing incoming excitatory and inhibitory synaptic potentials are summated in time (temporal summation) and between synapses (spatial summation). If the postsynaptic neurone is depolarized above a certain threshold it fires action potentials which are conducted along the axon to the next target cells.
The nervous system is conventionally divided into two major parts, the central nervous system (CNS) and the peripheral nervous system (PNS). The CNS consists of the brain and spinal cord. The PNS consists of the cranial and spinal nerves and their ramifications, and certain groupings of cell bodies that constitute the peripheral ganglia. Another convention divides the nervous system into somatic and autonomic components, with elements in both the CNS and PNS. The somatic nervous system controls the contraction of skeletal muscles. The autonomic nervous system, which may be subdivided into sympathetic and parasympathetic components, consists of neurones that innervate secretory glands, cardiac and smooth muscle and are therefore concerned primarily with control of the internal environment. Neurones in the wall of the gastrointestinal tract form the enteric nervous system, and are capable of sustaining local reflex activity that is independent of the CNS (Ch. 73).
CENTRAL NERVOUS SYSTEM
The brain and spinal cord (see Fig. 43.1A) contain the great majority of neuronal cell bodies in the nervous system. In many parts of the CNS, neuronal cell bodies are grouped together and are, more or less, segregated from axons. The generic term for such collections of cell bodies is grey matter. Small aggregations of neuronal cell bodies, which usually share a common functional role, are termed nuclei. Neuronal dendrites and synaptic interactions are mostly confined to grey matter. Axons tend to be grouped together to form white matter, so called because axons are often ensheathed in myelin which confers a paler colouration. Axons that pass between similar origins or destinations within the CNS tend to run together in defined pathways, or tracts. These often cross the midline (decussate), which means that one half of the body is, in many respects, controlled by, and sends information to, the opposite side of the brain.
Some groups of neurones in the spinal cord and brain stem that subserve similar functions are organized into longitudinal columns. The neurones in these columns may be concentrated into discrete, discontinuous nuclei in some areas, e.g. the cranial nerve nuclei of the brain stem, or they may form more or less continuous longitudinal bands, as in much of the spinal cord (Fig. 15.3). Efferent neurones constitute three such columns. The axons of motor neurones in the somatic motor column innervate muscles derived from head somites. The two other columns are related to specialized features of head morphology: neurones in the branchial motor column innervate muscles derived from the wall of the embryonic pharynx (branchial muscles) and the visceral motor column supplies preganglionic parasympathetic fibres to glands and visceral smooth muscle. Four longitudinal cell columns are related to sensory functions. The general somatic sensory column essentially deals with general sensory information from the head; special somatic sensory neurones are related to the special senses and receive vestibular and auditory input; general visceral sensory neurones deal with information from widespread and varied visceral sensory endings; special visceral sensory neurones are related to the special sense of taste.
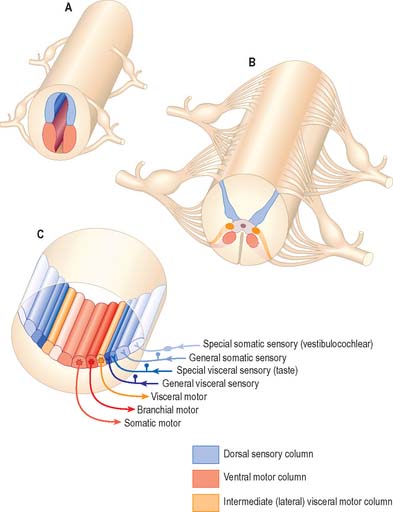
Fig. 15.3 Arrangement of sensory and motor cell columns in the spinal cord and brain stem. In each of parts A, B and C, dorsal is towards the top of the diagram. A shows the organization of the primitive spinal cord with a dorsal sensory column, a ventral motor column, and segmentally arranged dorsal and ventral nerve roots. B depicts the adult thoracic spinal cord with sensory and somatic motor columns and an additional intermediate (lateral) visceral motor column. C indicates the arrangement of multiple longitudinal columns in the brain stem, where the motor column is now subdivided into three, and the sensory column into four. For further information about the embryological aspects of the early nervous system consult Ch. 24. Consult also Fig 19.1.
To provide the energy required by constant neuronal activity, the CNS has a high metabolic rate and a rich blood supply (Ch. 17). A blood–brain barrier controls the neuronal environment and imposes severe restrictions on the types of substances that can pass from the bloodstream into nervous tissue (Ch. 3).
SPINAL CORD
The spinal cord lies within the vertebral column, in the upper two-thirds of the vertebral canal, and is continuous rostrally with the medulla oblongata of the brain stem (Ch. 43). For the most part, the spinal cord receives afferent input from, and controls the functions of, the trunk and limbs. Afferent and efferent connections between the periphery and the spinal cord travel in 31 pairs of segmentally arranged spinal nerves that attach to the cord as a linear series of dorsal and ventral rootlets. Adjacent groups of rootlets unite to form dorsal and ventral roots that combine to form the spinal nerves proper (Fig. 15.4). The dorsal and ventral roots are functionally distinct. Dorsal roots carry primary afferent nerve fibres from neuronal cell bodies located in dorsal root ganglia, whereas ventral roots carry efferent fibres from neuronal cell bodies located in the spinal grey matter.
Internally, the spinal cord consists of a central core of grey matter surrounded by white matter. The grey matter is configured in a characteristic H, or butterfly, shape that has projections known as dorsal and ventral horns (Fig. 15.5). In general, neurones situated in the dorsal horn are primarily concerned with sensory functions whilst those in the ventral horn are mostly associated with motor activities. At certain levels of the spinal cord a small lateral horn is additionally present, marking the location of the cell bodies of preganglionic sympathetic neurones. The central canal, a vestigial component of the ventricular system, lies at the centre of the spinal grey matter and runs the length of the cord. The white matter of the spinal cord consists of ascending and descending tracts that link spinal cord segments to one another and the spinal cord to the brain.
BRAIN
The brain (encephalon) lies within the cranium (Ch. 26). The brain receives information from, and controls the activities of, the trunk and limbs mainly through connections with the spinal cord. It also possesses 12 pairs of cranial nerves through which it communicates mostly with structures of the head and neck. The brain is divided into major regions on the basis of ontogenetic growth and phylogenetic principles (Fig. 15.6, Fig. 15.7, Fig. 15.8). Ascending in sequence from the spinal cord, the principal divisions are the rhombencephalon or hindbrain, the mesencephalon or midbrain, and the prosencephalon or forebrain.
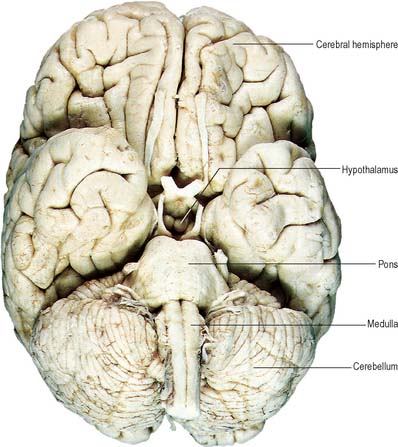
Fig. 15.7 Base of the brain, showing major divisions and cranial nerves.
(Figure enhanced by B Crossman, from a photograph by Kevin Fitzpatrick on behalf of GKT School of Medicine, London.)
The prosencephalon is subdivided into the diencephalon and the telencephalon. The diencephalon equates mostly to the thalamus and hypothalamus, but also includes the smaller epithalamus and subthalamus. The telencephalon is mainly composed of the two cerebral hemispheres or cerebrum. The diencephalon is almost completely embedded in the cerebrum and is therefore largely hidden from the exterior. The human cerebrum constitutes the major part of the brain. It occupies the anterior and middle cranial fossae and is directly related to the cranial vault. It consists of two cerebral hemispheres. The surface of each hemisphere is convoluted into a complex pattern of ridges (gyri) and furrows (sulci). Internally, each hemisphere has an outer layer of grey matter, the cerebral cortex, beneath which lies a thick mass of white matter (Fig. 15.9). One of the most important components of the cerebral white matter, the internal capsule (see Fig. 15.11), contains nerve fibres which pass to and from the cerebral cortex and lower levels of the neuraxis. Several large nuclei of grey matter, usually referred to as the basal ganglia, are partly embedded in the subcortical white matter. Connections between corresponding areas of the two sides of the brain cross the midline within commissures. By far the largest commissure is the corpus callosum, which links corresponding regions of the two cerebral hemispheres.
During prenatal development, the walls of the neural tube thicken greatly, but never completely obliterate the central lumen. Although the latter remains in the spinal cord as the narrow central canal, it becomes greatly expanded in the brain to form a series of interconnected cavities called the ventricular system (Ch. 16). In two regions, the fore- and hindbrains, parts of the roof of the neural tube do not generate nerve cells but become thin, folded sheets of highly vascular secretory tissue, the choroid plexuses. These secrete cerebrospinal fluid which fills the ventricles. The cavity of the rhombencephalon becomes expanded to form the fourth ventricle, which lies dorsal to the pons and upper half of the medulla. Caudally, the fourth ventricle is continuous with a canal in the caudal medulla and, through this, with the central canal of the spinal cord. The fourth ventricle is continuous with the subarachnoid space through three openings in its roof (the foramina of Luschka and of Magendie). At its rostral extent, the fourth ventricle is continuous with a narrow channel, the cerebral aqueduct, which passes through the midbrain. The rostral end of the cerebral aqueduct opens out into the third ventricle, a narrow, slit-like, midline cavity which is bounded laterally by the diencephalon. At the rostral end of the third ventricle, a small aperture on each side leads into the large lateral ventricle which is located within each cerebral hemisphere (see Fig. 15.6C).
OVERVIEW OF ASCENDING SENSORY PATHWAYS
Sensory modalities are conventionally described as being either special senses or general senses. The special senses are olfaction, vision, taste, hearing and vestibular function. Afferent information is encoded by highly specialized sense organs and transmitted to the brain in cranial nerves I, II, VII, VIII and IX (Ch. 25).
The general senses include touch, pressure, vibration, pain, thermal sensation and proprioception (perception of posture and movement). Stimuli from the external and internal environments activate a diverse range of receptors in the skin, viscera, muscles, tendons and joints (Ch. 3). Afferent impulses from the trunk and limbs are conveyed to the spinal cord in spinal nerves whilst those from the head are carried to the brain in cranial nerves. The detailed anatomy of the complex pathways by which the various general senses impinge upon conscious levels is better understood by reference to certain common organizational principles. Whilst undoubtedly oversimplified and subject to exceptions, this schema is helpful in emphasizing the essential similarities that exist between the ascending sensory systems.
In essence, ascending sensory projections related to the general senses consist of a sequence of three neurones that extends from peripheral receptor to contralateral cerebral cortex (Fig. 15.10). These are often referred to as either primary, secondary and tertiary neurones or first-, second- and third-order neurones. Primary afferents have peripherally located sensory endings and cell bodies that lie in dorsal root ganglia or the sensory ganglia associated with certain of the cranial nerves. Their axons enter the CNS through spinal or trigeminal nerves and terminate by synapsing on the cell bodies of ipsilateral second-order neurones: the precise location of this termination depends upon the modality.
OVERVIEW OF DESCENDING MOTOR PATHWAYS
Corticofugal fibres descend through the internal capsule and pass into the brain stem, where some of them (corticobulbar fibres) terminate. Corticobulbar fibres control the activity of brain stem neurones, including motor neurones within the cranial nerve nuclei. Current neuroanatomical nomenclature does not include a generally accepted term that denotes projections from the cerebral cortex to the brain stem as a whole. Such a term is, however, required in order to draw clinical comparison between the corticospinal system, coursing through the pyramids, and the functionally homologous fibres that terminate in the brain stem. The term ‘corticonuclear’ is sometimes used, but this implies specific innervation of cranial nerve nuclei and ignores the fact that numerous cortical efferents project to other sites, such as the reticular formation. The term ‘corticobulbar’ is also sometimes used but this, strictly speaking, refers to projections to the ‘bulb’, an archaic term for the medulla oblongata. In this text, ‘corticobulbar’ is used to denote cortical projections to the brain stem as a whole.
Corticospinal fibres (pyramidal tract) originate from widespread regions of the cerebral cortex, including the primary motor cortex of the frontal lobe where the opposite half of the body is represented in a detailed somatotopic fashion. They descend throughout the length of the brain stem; the majority cross to the contralateral side in the decussation of the pyramids in the medulla, subsequently continuing caudally as the lateral corticospinal tract of the spinal cord which terminates in association with interneurones and motor neurones of the spinal grey matter (Fig. 15.11). The principal function of the corticospinal and corticobulbar tracts is the control of fine, fractionated movements, particularly of those parts of the body where delicate muscular control is required. These tracts are particularly important in speech (corticobulbar tract) and movement of the hand (corticospinal tract).
The concept of upper and lower motor neurones is fundamental to the clinical description of the effects of lesions of the motor system. The signs and symptoms of upper and lower motor neurone lesions are very different and are indicative of the anatomical site of the lesion. ‘Lower motor neurones’ are the alpha motor neurones that innervate the extrafusal muscle fibres of skeletal muscle. In theory, the term ‘upper motor neurones’ refers collectively to all the descending pathways that impinge upon the activity of lower motor neurones. In common parlance, however, the term is often equated with the corticospinal tract. The terms upper and lower motor neurone lesion are used clinically to distinguish, for example, between the effects of a stroke in the internal capsule (a typical upper motor neurone lesion) and those of motor neurone disease (a typical lower motor neurone lesion).
Two other major systems that contribute to the control of movement are the basal ganglia and the cerebellum. The basal ganglia are a group of large subcortical nuclei, the major components being the caudate nucleus, putamen and globus pallidus (Fig. 15.9; Ch. 22). These structures have important connections with the cerebral cortex, certain nuclei of the thalamus and subthalamus and with the brain stem. They appear to be involved in the selection of appropriate movements and the suppression of inappropriate ones. Disorders of the basal ganglia cause either too little movement (akinesia) or abnormal involuntary movements (dyskinesias) as well as tremor and abnormalities of muscle tone. The basal ganglia are sometimes described as being part of the so-called ‘extrapyramidal (motor) system’. This term is used to distinguish between the effects of basal ganglia disease and those of damage to the ‘pyramidal’ (corticospinal) system. However, the progressive elucidation of the anatomy of the basal ganglia and of the pathophysiology of motor disorders has revealed the close functional interrelationship between the two ‘systems’, and has rendered the terms that distinguish them largely obsolete (Brodal 1981). The cerebellum (Ch. 20) has rich connections with the brain stem, particularly the reticular and vestibular nuclei, and with the thalamus. It is concerned with the coordination of movement: cerebellar disorders cause ataxia, intention tremor and hypotonia.
PERIPHERAL NERVOUS SYSTEM
CRANIAL NERVES
Briefly, there are 12 pairs of cranial nerves. They are individually named and numbered (Roman numerals) in a rostrocaudal sequence (Table 15.1). Unlike spinal nerves, only some cranial nerves are mixed in function, carrying both sensory and motor fibres. Others are purely sensory or purely motor. The first cranial nerve (I; olfactory) has an ancient lineage and is derived from the forerunner of the cerebral hemisphere. It retains this unique position through the connections of the olfactory bulb, and is the only sensory cranial nerve that projects directly to the cerebral cortex rather than indirectly via the thalamus. The areas of cerebral cortex receiving olfactory input have a primitive cellular organization and are an integral part of the limbic system, which is concerned with the emotional aspects of behaviour (Ch. 23). The second cranial nerve (II; optic) consists of the axons of second order visual neurones and these terminate in the thalamus. The other ten pairs of cranial nerves attach to the brain stem and most of their component fibres originate from, or terminate in, the cranial nerve nuclei of the brain stem (Ch. 19).
The sensory fibres in individual spinal and cranial nerves have characteristic, but often overlapping, peripheral distributions. As far as the innervation of the body surface is concerned, the area that is supplied by a particular spinal or cranial nerve is referred to as a dermatome. There is marked overlap between dermatomes of adjacent spinal nerves, particularly for the segments least affected by development of the limbs, i.e. the second thoracic to the first lumbar. In some regions, e.g. the upper anterior thoracic wall, the cutaneous nerves that supply adjoining areas are not from consecutive spinal nerves and the overlap is minimal. Maps of dermatome distribution are useful in clinical neurology to identify the location of pathology in patients with peripheral sensory deficits. Dermatome maps are somewhat inconsistently reported by different authors, reflecting the fact that the maps are composite constructs that have been compiled to a large extent by clinical observations on patients with cranial or spinal nerve pathology (Fig. 15.12). Detailed dermatome maps are described on a regional basis (Chs 42, 45, 79). The motor axons of individual spinal and cranial nerves tend to innervate anatomically and functionally related groups of skeletal muscles, that are referred to as myotomes.
AUTONOMIC NERVOUS SYSTEM
Visceral efferent pathways differ from their somatic equivalents in that the former are interrupted by peripheral synapses, there being a sequence of at least two neurones between the CNS and the target structure (Fig. 15.13). These are referred to as preganglionic and postganglionic neurones, respectively. The somata of preganglionic neurones are located in the visceral efferent nuclei of the brain stem and in the lateral grey columns of the spinal cord. Their axons, which are usually finely myelinated, exit from the CNS in certain cranial and spinal nerves and then pass to peripheral ganglia, where they synapse with postganglionic neurones. The axons of postganglionic neurones are usually unmyelinated. Postganglionic neurones are more numerous than preganglionic ones; one preganglionic neurone may synapse with 15 to 20 postganglionic neurones, which permits the wide distribution of many autonomic effects.
Two long-held dogmas are that the sympathetic and parasympathetic nervous systems are functionally antagonistic (because activation of their respective efferents has opposing actions upon target structures) and that sympathetic reactions are mass responses whereas parasympathetic reactions are usually more localized. A better concept is that the two sets of neurones constitute an integrated system for the coordinated neural regulation of visceral and homeostatic functions. Moreover, even though widespread activation of the sympathetic nervous system may occur, e.g. in association with fear or rage, it is recognized that the sympathetic nervous system is also capable of discrete activation. In general, sympathetic activity results in the constriction of cutaneous arteries (increasing blood supply to the heart, muscles and brain), cardiac acceleration, an increase in blood pressure, contraction of sphincters and depression of peristalsis, all of which mobilize body energy stores for dealing with increased activity. Parasympathetic activity results in cardiac slowing and an increase in intestinal glandular and peristaltic activities, which may be considered to conserve body energy stores.
The classical concept of autonomic neurotransmission is that preganglionic neurones of both sympathetic and parasympathetic systems are cholinergic and that postganglionic parasympathetic neurones are also cholinergic while those of the sympathetic nervous system are noradrenergic. The discovery of neurones that do not use either acetylcholine or noradrenaline (norepinephrine) as their primary transmitter, and the recognition of a multiplicity of substances in autonomic nerves which fulfil the criteria for a neurotransmitter or neuromodulator, have greatly complicated neuropharmacological concepts of the autonomic nervous system. Thus, adenosine 5′-triphosphate (ATP), numerous peptides and nitric oxide have all been implicated in the mechanisms of cell signalling in the autonomic nervous system. The principal cotransmitters in sympathetic nerves are ATP and neuropeptide Y, in parasympathetic nerves vasoactive intestinal polypeptide (VIP) and in enteric nerves ATP, VIP and substance P.
SYMPATHETIC NERVOUS SYSTEM
The sympathetic trunks are two ganglionated nerve cords that extend on either side of the vertebral column from the cranial base to the coccyx (Ch. 55). The ganglia are joined to spinal nerves by short connecting nerves called white and grey rami communicantes. Preganglionic axons join the trunk through the white rami communicantes while postganglionic axons leave the trunk in the grey rami. In the neck each sympathetic trunk lies posterior to the carotid sheath and anterior to the transverse processes of the cervical vertebrae. In the thorax the trunks are anterior to the heads of the ribs, in the abdomen they lie anterolateral to the bodies of the lumbar vertebrae, and in the pelvis they are anterior to the sacrum and medial to the anterior sacral foramina. Anterior to the coccyx the two trunks meet in a single, median, terminal ganglion. Cervical sympathetic ganglia are usually reduced to three by fusion. The internal carotid nerve, a continuation of the sympathetic trunk, issues from the cranial pole of the superior ganglion and accompanies the internal carotid artery through its canal into the cranial cavity. There are from 10 to 12 (usually 11) thoracic ganglia, 4 lumbar ganglia and 4 or 5 ganglia in the sacral region.
The cell bodies of preganglionic sympathetic neurones are located in the lateral horn of the spinal grey matter of all thoracic segments and the upper two or three lumbar segments (Fig. 15.14). Their axons are myelinated with diameters of 1.5–4 μm. They leave the cord in the corresponding ventral nerve roots and pass into the spinal nerves, but soon leave in white rami communicantes to join the sympathetic trunk (Fig. 15.15). Neurones like those in the lateral grey column exist at other levels of the cord above and below the thoracolumbar outflow and small numbers of their fibres leave in other ventral roots. Preganglionic sympathetic neurones release acetylcholine as their principal neurotransmitter.
On reaching the sympathetic trunk, preganglionic fibres behave in one of several ways (Fig. 15.15). They may synapse with neurones in the nearest ganglion, or traverse the nearest ganglion and ascend or descend in the sympathetic chain to end in another ganglion. A preganglionic fibre may terminate in a single ganglion or, through collateral branches, synapse with neurones in several ganglia. Preganglionic fibres may traverse the nearest ganglion, ascend or descend and, without synapsing, emerge in one of the medially directed branches of the sympathetic trunk to synapse in the ganglia of autonomic plexuses (mainly situated in the midline, e.g. around the coeliac and mesenteric arteries). More than one preganglionic fibre may synapse with a single postganglionic neurone. Uniquely, the suprarenal gland is innervated directly by preganglionic sympathetic neurones that traverse the sympathetic trunk and coeliac ganglion without synapse.
PARASYMPATHETIC NERVOUS SYSTEM
Preganglionic parasympathetic neurone cell bodies are located in certain cranial nerve nuclei of the brain stem (see Fig. 19.1) and in the grey matter of the second to fourth sacral segments of the spinal cord. Efferent fibres, which are myelinated, emerge from the CNS only in cranial nerves III, VII, IX and X and in the second to fourth sacral spinal nerves. Preganglionic parasympathetic neurones are cholinergic.
The cell bodies of postganglionic parasympathetic neurones are mostly sited distant from the CNS, either in discrete ganglia located near the structures innervated, or dispersed in the walls of viscera. In the cranial part of the parasympathetic system there are four small peripheral ganglia, ciliary, pterygopalatine, submandibular and otic, that are all described on a regional basis in Section 4. These are solely efferent parasympathetic ganglia, unlike the trigeminal, facial, glossopharyngeal and vagal ganglia, all of which are concerned exclusively with afferent impulses and contain the cell bodies of sensory neurones. The cranial parasympathetic ganglia are also traversed by afferent fibres, postganglionic sympathetic fibres, and, in the case of the otic ganglion, even by branchial efferent fibres; none of these fibres synapse in the ganglia. Postganglionic parasympathetic fibres are usually unmyelinated and shorter than their counterparts in the sympathetic system, because the ganglia in which the parasympathetic fibres synapse are in or near the viscera they supply. Postganglionic parasympathetic neurones are cholinergic.
ENTERIC NERVOUS SYSTEM AND INTRINSIC NEURONES
Many peripheral autonomic ganglia contain neurones derived from the neural crest during embryonic development that are anatomically distinct from classical sympathetic and parasympathetic neurones. Connections between these intrinsic neurones allow them to sustain and modulate visceral activities by local reflex mechanisms. The enteric nervous system consists of many millions of neurones and enteric glial cells grouped into ganglionated plexuses lying in the wall of the gastrointestinal tract: ganglia containing neuronal cell bodies and glia are connected by bundles of axons to form myenteric and submucous plexi that extend from the oesophagus to the anal sphincter (Chs 3 and 60). This intrinsic circuitry mediates numerous reflex functions including the contractions of the muscular coats of the alimentary tract, secretion of gastric acid, intestinal transport of water and electrolytes and the regulation of mucosal blood flow. Although complex interactions occur between the enteric and sympathetic and parasympathetic nervous systems, the enteric nervous system is capable of sustaining local reflex activity independent of the CNS.
VISCERAL AFFERENT PATHWAYS
In general, afferent fibres that accompany pre- and postganglionic sympathetic fibres have a segmental arrangement and end in spinal cord segments from which preganglionic fibres innervate the region or viscus concerned. General visceral afferents entering thoracic and upper lumbar spinal segments are largely concerned with pain. Nociceptive impulses from the pharynx, oesophagus, stomach, intestines, kidneys, ureter, gallbladder and bile ducts seem to be carried in sympathetic pathways. Cardiac nociceptive impulses enter the spinal cord in the first to fifth thoracic spinal nerves, mainly via the middle and inferior cardiac nerves, but some fibres pass directly to the spinal nerves. It is said that there are no general visceral afferents in the superior cardiac nerves. Peripherally, the fibres pass through the cardiac plexuses and along the coronary arteries. Myocardial anoxia may evoke symptoms of angina pectoris in which pain is typically presternal, and is also referred to much of the left chest, and radiates to the left shoulder, the medial aspect of the left arm, along the left side of the neck to the jaw and occiput, and down to the epigastrium. Cardiac afferents carried in vagal cardiac branches are concerned with the reflex depression of cardiac activity. Ureteric pain fibres, also running with sympathetic fibres, are presumably involved in the agonizing renal colic that follows obstruction by calculi. Afferent fibres from the testis and ovary run through the corresponding plexuses to somata in the tenth and eleventh thoracic dorsal root ganglia.
PARAGANGLIA
Paraganglia are extrasuprarenal aggregations of chromaffin tissue, distributed near to or within the autonomic nervous system (Fig. 15.16). Cells like those in paraganglia also occur in the sympathetic ganglia of various viscera and in a variety of retroperitoneal and mediastinal sites. All are derived from the neural crest, and all synthesize and store catecholamines. Their functions differ with location: some cells act as interneurones, others as sources of neuroendocrine secretion. Paraganglionic catecholamine release occurs mainly in response to chemical rather than to neural stimuli, e.g. in the suprarenal medulla. Extrasuprarenal chromaffin tissue is prominent in the fetus, and acts as the main source of catecholamines while the suprarenal medulla is immature. Although many paraganglia degenerate soon after birth, others persist into adulthood, often as microscopic paraganglia.
. The Peripheral Nervous Systems. Björklund A, Hökfelt T, Owman C, editors. Handbook of Chemical Neuroanatomy, Vol. 6. Amsterdam: Elsevier, 1988.
Description of sensory-motor nerves..
Brodal A. Neurological Anatomy in Relation to Clinical Medicine, 3rd edn. Oxford: Oxford University Press, 1981.
Burnstock G. Co-transmission. The fifth Heymans lecture. Arch Int Pharmacodyn Ther. 1990;304:7-33.
A review of neurotransmission in the autonomic nervous system..
Burnstock G, editor. The Autonomic Nervous System, Vols 1–14. Switzerland: Harwood Academic Publishers, 1992–95.
Contains extensive material on the neurotransmitters of the autonomic nervous system..
Crossman AR, Neary D. Neuroanatomy. An Illustrated Colour Text. 2nd edn.. Edinburgh: Churchill Livingstone; 2000.
England MA, Wakely J. A Colour Atlas of the Brain and Spinal Cord. London: Wolfe Publishing, 1991.
Greenberg SA. The history of dermatome mapping. Arch Neurol. 2003;60:126-131.
Haines DE. Neuroanatomy. An Atlas of Structures, Sections and Systems. 5th edn.. Philadelphia: Lippincott Williams & Wilkins; 2000.
Maggi CA. The pharmacology of the efferent function of sensory nerves. J Auton Pharmacol. 1991;11:173-208.
Vinken PJ, Bruyn GW. The Autonomic Nervous System. Part 1. Normal Functions. Appenzeller O, volume ed., Amsterdam and London: Elsevier Science, 1999.
Vinken PJ, Bruyn GW. The Autonomic Nervous System. Part 2. Dysfunctions. Appenzeller O, volume ed., Amsterdam and London: Elsevier Science, 2000.