31 Otorhinolaryngologic Procedures
OTORHINOLARYNGOLOGIC PROCEDURES REPRESENT A large segment of elective pediatric surgery. Anesthetic management of these children is provided by both pediatric and general anesthesiologists, frequently working in anesthesia care teams, most commonly in ambulatory surgery centers and office practices.1 Additionally, anesthesiologists are often consulted to help manage potentially life-threatening pediatric otolaryngologic emergencies. These include airway obstruction suffering from croup, foreign body aspiration, airway trauma and acute epiglottitis.2 In both the elective and emergent scenarios, it is essential to understand the pathophysiology, and to discuss the anesthetic plan in advance of the procedure with the surgeon, who will frequently be sharing the airway with the anesthesiologist. This ensures safe anesthetic management and ideal conditions for both children and surgeons.
Anesthesia for Otologic Procedures
Myringotomy and Ventilating Tube Insertion
Myringotomy with tube insertion is a very brief operation, usually performed as ambulatory surgery using a potent inhalational agent (e.g., sevoflurane), oxygen, and nitrous oxide administered by facemask with spontaneous respirations. An oropharyngeal airway may assist in maintaining a patent airway when the head is laterally rotated and reduces head movement (which is amplified through the microscope). Gentle manual assistance of ventilation can also help reduce head movement. Occasionally, a laryngeal mask airway (LMA) may be used in children in whom the procedure is expected to be prolonged (e.g., children with narrow ear canals) or those with a difficult airway. Most children can be managed safely without intravenous (IV) access,3 but it is reasonable to have an IV setup ready. Some children with severe underlying medical or surgical conditions will require IV access, despite the anticipated brief duration of the minor procedure. Although premedication is often omitted because their duration of action exceeds that of the procedure, an anxious child may still benefit from a sedative premedication.
Discomfort after myringotomy and tube insertion is usually managed by the administration of acetaminophen, either via the oral route preoperatively or the rectal route intraoperatively. The recommended dose of acetaminophen to achieve therapeutic blood levels is 10 to 20 mg/kg when administered via the oral route, and 30 to 40 mg/kg when administered via the rectal route.4–7 Oral acetaminophen is very rapidly absorbed, achieving therapeutic blood levels in minutes, whereas rectal acetaminophen is slowly absorbed, with a time to onset of action of 60 to 90 minutes, and a time to peak effect of 1 to 3 hours.8–11 Consequently, the oral route is preferred for this procedure.
Preschool-aged children who receive sevoflurane without an analgesic for myringotomy and tube insertion may exhibit emergence delirium and postoperative agitation (see Chapter 4). Although pain may be partially responsible for these responses, their etiologies are not completely understood. Because the procedure is so brief and IV access is not usually established, intranasal fentanyl, 1 to 2 µg/kg, has been shown to provide analgesia and to reduce the frequency of emergence agitation.12,13 The only significant side effect is a 12% incidence of vomiting when oral fluids are administered in the early postoperative period.5 Other medications, including IV ketorolac (1 mg/kg), or intranasal butorphanol (25 µg/kg), and intranasal dexmedetomidine (1 to 2 µg/kg), have been shown to reduce the pain after myringotomy and tube insertion.14–16 However larger doses of dexmedetomidine (2 µg/kg) significantly prolong the duration of stay in the postanesthesia care unit (PACU). Some practitioners prefer to use more soluble anesthetics, such as isoflurane, for anesthesia maintenance to reduce the incidence of agitation after myringotomy and tube insertion, although there is limited evidence to support this practice.
Children with chronic otitis frequently have persistent rhinorrhea and suffer recurrent upper respiratory tract infection (URI) (see Chapter 11). Eradication of middle ear congestion and improved fluid drainage often resolves the concomitant symptoms. The frequency of perioperative complications in children with mild URIs is similar to that in children who are asymptomatic. In general, morbidity is not increased in children who present for minor surgery with acute uncomplicated mild URIs, provided tracheal intubation can be avoided.17,18 Canceling this surgery because of rhinorrhea or recurrent mild respiratory symptoms is not usually justifiable. It is, however, recommended that children with respiratory symptoms have their oxygen saturation measured before induction of general anesthesia, and that supplemental oxygen is administered postoperatively to those whose oxygen-saturation readings are less than 93%.19
Middle Ear and Mastoid Surgery
To gain access to the surgical site, the child’s head is placed on a headrest, which may be positioned below the operating table. In addition, extreme degrees of lateral rotation may be required to visualize the middle ear anatomy. The anesthesiologist and surgeon must be especially vigilant to ensure that nerves, muscles, and bony structures are not injured as a result of this unusual positioning; the sternocleidomastoid muscles generally limit the safe degree of lateral head rotation. Left or right tilting (airplaning) of the operating room (OR) table minimizes the need for extreme lateral head rotation as in the case of children with Down syndrome. The laxity of the ligaments of the cervical spine, as well as immaturity of the odontoid process in these children predisposes them to C1-C2 subluxation. Of children with Down syndrome or achondroplasia, 15% to 31% have atlantoaxial instability.20–23 Anteroposterior positioning requires the utmost care to avoid injury. Positioning of the OR table to allow access to the respective middle ear and accommodate all the extra surgical equipment can also pose a challenge. Depending on the room configuration, the table may be rotated 90 degrees or even 180 degrees away from the anesthesia machine, necessitating the use of extra-long breathing circuits (Fig. 31-1). As a result of the limited access to the airway, very careful attention must be paid to securing the tracheal tube. Draping must allow immediate access to the airway should that be required.
The middle ear and sinuses are air-filled, nondistensible cavities. An increase in the volume of gas within these cavities increases the pressure within the cavities. Nitrous oxide diffuses along a concentration gradient into air-filled middle ear spaces more rapidly than nitrogen moves out because nitrous oxide is 34 times more soluble in blood than nitrogen. The middle ear is vented through the opening of the eustachian tube. Normal passive venting of the eustachian tube occurs at 20 to 30 cm H2O pressure. Nitrous oxide increases the pressures within the middle ear such that they exceed the ability of the eustachian tube to vent the middle ear within 5 minutes, leading to pressure buildup.24 If the function of the eustachian tube is compromised during the surgical procedure, then pressure in the middle ear can increase further. Venting the middle ear occurs intermittently, and leads to constant fluctuations in middle ear pressure that cause movement of the tympanic membrane.25 During procedures in which the tympanic membrane is replaced or a perforation is patched, nitrous oxide should be discontinued or, if this is not possible, limited to a maximum of 50% of the concentration before the application of the tympanic membrane graft to reduce the potential for pressure-related displacement.26 The omission of nitrous oxide does not significantly increase the requirements (minimal alveolar concentration) for the less-soluble inhaled anesthetics, desflurane, or sevoflurane in children.27 After nitrous oxide is discontinued, it is quickly reabsorbed, creating a void in the middle ear, with resulting negative pressure. This negative pressure may result in serous otitis, disarticulation of the ossicles in the middle ear (especially the stapes), and hearing impairment, which may last up to 6 weeks postoperatively. The use of nitrous oxide may increase the incidence of postoperative nausea and vomiting (PONV), as a direct result of negative middle ear pressure during recovery. The negative pressure created by the reabsorption of nitrous oxide stimulates the vestibular system by producing traction on the round window. Although all children are at risk for PONV, older children and adolescents, in particular, seem to be at greatest risk.28 Prophylactic administration of antiemetics (e.g., dexamethasone and ondansetron) is usually warranted. Local infiltration of the great auricular nerve can provide pain relief equivalent to that of opioids and may reduce the incidence of opioid-induced vomiting (see Chapter 41).29
Cochlear Implants
In recent years, the indications for cochlear implants have broadened and continue to evolve. With the application of universal neonatal hearing screening programs, a large pool of hearing-impaired infants has been identified. The benefits of early intervention with cochlear implants are being explored. Younger children with severe to profound hearing loss markedly improve their auditory, speech, and language skills after cochlear implants, and more of these children can be mainstreamed with their age-appropriate hearing peers when they receive an implant early in life. Experience has shown that cochlear implant surgery is safe in infants older than 6 months of age, provided that special attention is paid to the physiologic and anatomic differences present in this age-group. Surgery requires meticulous care with hemostasis, soft tissue dissection, and bone drilling because bleeding from bone can be difficult to control and can complicate the surgical outcome. Availability of skilled postoperative nursing and a pediatric intensive care unit (ICU) is also essential.30 Postoperative fitting of the externally worn speech processor is very important for successful use of the cochlear implant. However, this fitting process can be difficult, particularly in infants and young children, because of limited communication capabilities. Stapedius reflex thresholds obtained intraoperatively have been used for postoperative speech processor fitting, although the influence of anesthetics on the threshold values must be taken into account. More reliable threshold values can be obtained by adjusting the dosage of hypnotics to achieve a lighter level of hypnosis during stapedius reflex measurement.31 In most children, increasing the concentration of inhalational anesthetics increases the stapedius reflex threshold. As always, appropriate communication with the surgeon will help ensure a successful outcome.
Anesthesia for Rhinologic Procedures
Chronic sinusitis in children can be caused by antibiotic-resistant bacteria and is usually treated with broad-spectrum antibiotics. In some children with obstructive adenoid pads, adenoidectomy will improve the signs and symptoms of sinusitis. Functional endoscopic sinus surgery using sharp biting instruments and/or a microdebrider has become the primary method of surgical therapy for chronic sinusitis.32 Current techniques aim to leave the mucosa intact to prevent scarring in the frontal recess. Although sometimes controversial, there is no evidence at present that functional endoscopic sinus surgery affects facial growth in children. Of interest to the anesthesiologist is that many children who require functional endoscopic sinus surgery have coexisting medical problems, such as asthma and cystic fibrosis. These conditions must be optimized before surgery (see Chapter 11).
Because bleeding is inevitable with this surgery and can interfere with the surgical exposure, packing the nasal cavity with a vasoconstricting solution is frequently done before surgery commences. The most commonly used topical vasoconstrictors include oxymetazoline 0.025% to 0.05%, phenylephrine 0.25% to 1%, and cocaine 4% to 10%. It is important for the anesthesiologist to be aware of the type and dose of the vasoconstrictor used and that no more than the maximum effective dose is applied. Application of topical phenylephrine or other potent vasoconstrictors to mucous membranes or open surgical sites can cause severe hypertension, reflex bradycardia, and even cardiac arrest.33 Hypertension that is induced by topically applied vasoconstrictors often resolves spontaneously and may not require aggressive treatment. The use of β-adrenergic blockers or calcium-channel blockers to control blood pressure in these circumstances can depress cardiac output, leading to pulmonary edema and cardiac arrest.33 It is recommended that the initial topical dose of phenylephrine should not exceed 20 µg/kg in children.33
Corticosteroids, such as IV dexamethasone (0.25 to 0.5 mg/kg), are usually administered to reduce swelling and scarring. Frequently, the surgeon will want to leave an absorbable stenting material, such as MeroGel (Medtronic ENT, Jacksonville, Fla.), at the end of surgery. Unfortunately, this will interfere with nasal breathing and may increase the incidence of emergence agitation. An anesthetic technique that ensures adequate analgesia and rapid return of consciousness at the end of surgery is therefore desirable. One of us (RSH) has found that a combination of desflurane, fentanyl, and low-dose propofol works well in this regard. A unilateral or bilateral infraorbital nerve block can also be performed via the intraoral or extraoral route to provide analgesia (see Chapter 41).34 One further concern is the need to avoid nonsteroidal antiinflammatory drugs (NSAIDs) in children with asthma and sinusitis secondary to nasal polyps (Samter triad).35
Adenotonsillectomy
Adenotonsillectomy is one of the oldest and most commonly performed pediatric surgical procedures worldwide. More selective indications, however, have reduced the annual caseload.36,37 Chronic or recurrent tonsillitis and obstructive adenotonsillar hyperplasia are the major indications for surgical removal, although other indications do exist (Table 31-1).38,39 Surgical treatment is required when tonsillitis recurs despite adequate medical therapy, or when it is associated with peritonsillar abscess or acute airway obstruction. Halitosis, persistent pharyngitis, and cervical adenitis may accompany chronic tonsillitis. Tonsillar hyperplasia may lead to chronic airway obstruction, resulting in sleep apnea, CO2 retention, intermittent nocturnal hypoxemia, cor pulmonale, failure to thrive, swallowing disorders, and speech abnormalities (Fig. 31-2). Many of these adverse effects are reversible with surgical excision of the tonsils. Certain children with cardiac lesions may be at risk for endocarditis caused by recurrent streptococcal bacteremia secondary to infected tonsils and will require prophylactic antibiotics (see Chapter 14).
TABLE 31-1 Indications for Adenotonsillectomy
Surgical techniques for adenotonsillectomy include guillotine and snare techniques, cold and hot knife dissection, suction, radiofrequency ablation, and unipolar and bipolar electrocautery techniques. A major advantage of the electrocautery dissection technique is a reduction in the incidence of intraoperative blood loss, as well as postoperative hemorrhage; a major disadvantage is greater pain and poor oral intake postoperatively.40–44
The mortality associated with adenotonsillectomy is estimated at 1 per 16,000 to 1 per 35,000 procedures.45,46 Hospital-based reviews of anesthetic mortality continue to list adenotonsillectomy as a surgical procedure associated with perioperative cardiac arrest and death.47,48 Bleeding, burns, and airway fires account for over one third of malpractice claims associated with this procedure.49
Surgical complications after adenotonsillectomy are rare but include uvular amputation, uvular edema, velopharyngeal insufficiency, and nasopharyngeal stenosis. Atlantoaxial subluxation manifesting as neck pain and torticollis, mandibular subluxation and condylar fracture, cervical adenitis, and cervical osteomyelitis have also been reported.46,50
Throat pain, otalgia, emesis, poor oral intake, and dehydration are common morbidities. Respiratory morbidity after adenotonsillectomy in the otherwise healthy child affects less than 1%,51–53 but has assumed a greater importance since obstructive breathing has replaced infection and halitosis as the most common indication for adenotonsillectomy.
Age has a major influence on postadenotonsillectomy complications. Secondary postadenotonsillectomy hemorrhage is more common in children older than the age of 10 years.54,55 Young age is a risk factor for both poor oral intake and respiratory complications. The majority of children younger than age 3 years experience airway problems after adenotonsillectomy for obstructive breathing.56,57
Preoperative Evaluation
The general health of the child and the indications for surgery must be reviewed. URIs are frequent in these children and can interfere with the timing of adenotonsillectomy because the risk of respiratory morbidity and hemorrhage is increased.46,51,58,59 A history of bleeding tendencies requires investigation. Medications that interfere with coagulation include aspirin, NSAIDs, and valproic acid. Discontinuation of these drugs preoperatively is sometimes problematic, and preoperative consultation with neurology, cardiology, and hematology specialists may be indicated.
A careful cardiorespiratory history and physical examination is essential. Children with chronic tonsillar hypertrophy may have long-standing hypoxemia and hypercarbia, which can lead to cor pulmonale (see Fig. 31-2). The oropharynx should be evaluated and the tonsillar size classified (Fig. 31-3).60 In some centers, a complete blood cell count is required before adenotonsillectomy. There is no evidence that routinely performed preoperative coagulation studies are beneficial unless they are indicated by history.61,62 The indications for the procedure should be clearly delineated. If the indication is for obstructed breathing, then further evaluation for symptoms of possible obstructive sleep apnea (OSA) is indicated. Parents should be asked if the child snores loudly, if the snoring can be heard through a closed door, if there are gasps or pauses in respirations, if there is daytime somnolence, night terrors, nocturnal enuresis, attention deficit disorder, or poor school performance. A positive response to any of these questions is suggestive of OSA, in particular when combined with obesity (weight greater than the 95th percentile).63–67
Special Considerations for the Child with OSA
The single most important task during the preoperative evaluation of the child for adenotonsillectomy is to distinguish the child with OSA from the child with obstructive breathing, because the former is at greater risk for developing severe perioperative respiratory complications, possibly including death, after adenotonsillectomy.68–71b
OSA is the most severe form of sleep-disordered breathing. Sleep-disordered breathing ranges from normal respirations to primary snoring, upper airway resistance syndrome (UARS), obstructive hypopnea, and OSA. At the most extreme form of sleep-disordered breathing, OSA, clinical signs of partial or complete upper airway obstruction (UAO) must be present during sleep, as well as some degree of hypercarbia and/or hypoxemia.72 Although it is important to recognize the significance of OSA in children who are scheduled for adenotonsillectomy, children who do not meet the criteria for OSA but who have less severe forms of sleep-disordered breathing, such as UARS or obstructive hypopnea, may also be at increased risk for morbidity after surgery. Guidelines for the perioperative management of these children continue to be developed.71a,73,74
A high index of suspicion is required to identify the child with OSA on clinical criteria, although clinical criteria do not distinguish primary snoring from OSA in children.75 There is a greater incidence of OSA in Asian and African American populations.76,77 In addition, African American children desaturate more profoundly during sleep-related obstructive airway events than do Caucasian and Hispanic children78; the reason for this difference is unclear.
Anatomic features, including increased nasal resistance, may underlie the pathogenesis of OSA; common medical conditions and syndromes that predispose to the development of OSA are listed in Table 31-2. Infants suffering acute life-threatening events have a greater incidence of OSA in childhood and adolescence.79–81
TABLE 31-2 Medical Conditions in Children That Predispose to Development of Obstructive Sleep Apnea
The obstructive events that characterize OSA result in recurrent episodes of hypoxia, hypercarbia, and sleep disruption, a trilogy that has been linked to the development of medical sequelae that accompany severe OSA. Because adenotonsillectomy is very often the initial treatment for the majority of children, these children may present with a spectrum of disease affecting multiple organ systems. Failure to thrive is common. Cardiovascular abnormalities, including ventricular dysfunction, a depressed ventricular ejection fraction, right ventricular hypertrophy, and pulmonary hypertension, may be present.82–85 Repeat infections affecting the lower respiratory tract have been linked to chronic aspiration.86
The severity of OSA is assessed by the frequency and severity of the obstructive respiratory events during sleep; both vary with sleep stage and occur most often during rapid eye movement (REM) sleep. The frequency and severity of obstructive events worsen after midnight, a finding that may reflect the greater proportion of REM sleep in the latter part of the night and fatigue of the upper airway musculature.87–89
Apneas are classified as central, obstructive, and mixed. Central apnea occurs when there is no apparent respiratory effort. Obstructive apnea is associated with apparent, often vigorous, inspiratory efforts that are ineffective because lack of upper airway patency. A mixed obstructive apnea is diagnosed when both central and obstructive apnea occur without interruption by effective respirations. The presence of sleep-disordered breathing is documented by polysomnography and is quantitated by the frequency of obstructive events and by oxygen-desaturation indices. The polysomnogram simultaneously records the electroencephalogram, electromyogram, electrocardiogram, pulse oximetry, airflow, and thoracic and abdominal movement during sleep. A recent consensus paper on the criteria for diagnosing OSA in children has been developed.71a,84 A common definition of obstructive apnea in children is an obstructive effort that includes more than two obstructive breaths, regardless of the duration of the apnea.87 An obstructive apnea index of 1 is the cutoff for normality in children.90 Hypopnea is defined as a reduction in airflow of more than 50%.87 The apnea hypopnea index (AHI) is the summation of the number of obstructive apnea and hypopnea events and is analogous to the respiratory disturbance index (RDI). A common definition of desaturation is a 4% decrease in oxygen saturation from baseline. The saturation nadir is the minimum oxygen saturation recorded during the sleep study. A saturation nadir of 92% is the minimum normal saturation in children.90,91
The severity of OSA predicts the nature of perioperative respiratory complications (Table 31-3). An RDI of greater than 20 events per hour is associated with breath holding during induction, whereas an RDI greater than 30 is associated with laryngospasm and desaturation during emergence.92 Ten obstructive events per hour during a screening polysomnogram is the threshold for severe postoperative respiratory complications.69 An oxygen-saturation nadir less than 80% is associated with a greater incidence of respiratory morbidity after adenotonsillectomy, compared with a saturation nadir greater than 80%.68,71
TABLE 31-3 Clinical Diagnostic Criteria for Pediatric Obstructive Sleep Apnea Syndrome
1. Predisposing physical characteristics
2. History of apparent airway obstruction during sleep (two or more of the following)
Note: If signs and symptoms in at least two categories are present, there is a significant probability of moderate obstructive sleep apnea (OSA). If severe abnormalities are present, children should be treated as having severe OSA.
Modified from Table 1 in Gross JB, Bachenberg KL, Benumof JL, et al. Practice guidelines for the perioperative management of patients with obstructive sleep apnea: a report by the American Society of Anesthesiologists Task Force on Perioperative Management of patients with obstructive sleep apnea. Anesthesiology 2006;104:1081-93.
The RDI and AHI correlate inversely with the oxygen-saturation nadir,93,94 making simplified testing with continuous pulse oximetry a meaningful metric. The McGill oximetry score has been shown to correlate with the risk of respiratory complications after adenotonsillectomy in children (Fig. 31-4). Of children with a McGill oximetry score of 4, 24% experienced major postoperative respiratory complications.94
Consultations to plan the perioperative care of children with severe OSA are important. Young children with profound oxygen desaturation during sleep and CO2 retention may require admission to the pediatric ICU for optimization before and/or after adenotonsillectomy.36,94 Urgent adenotonsillectomy for severe OSA is associated with significant respiratory morbidity after surgery.70,95 On occasion, adenotonsillar hypertrophy may progress to compromise the upper airway during wakefulness. In some instances the anesthetic considerations for the obstructed and difficult airways may overlap.
Anesthetic Management and Postoperative Considerations
The anesthetic goals for adenotonsillectomy are (1) to provide a smooth, atraumatic induction; (2) to provide the surgeon with optimal operating conditions; (3) to establish IV access for volume expansion and medications as indicated; and (4) to provide rapid emergence so that the child is awake and able to protect the recently instrumented airway. The need for a premedication is determined during the preanesthetic evaluation. Children with symptoms of sleep-disordered breathing who require premedication should be closely observed, although the desaturation is transient and infrequent (1.5% of cases) after oral midazolam premedication.96 Monitoring with pulse oximetry after premedication may be indicated for select children with severe OSA and confounding variables.96 Premedication with short-acting drugs and/or those that can be antagonized is advised (see further).
The anesthetic techniques for adenotonsillectomy are varied and include the choice of an inhalational or IV technique, the choice of an ETT or LMA, and the choice of spontaneous or controlled ventilation. Of the currently available inhalational agents, sevoflurane provides a smooth induction of anesthesia, and desflurane (for those whose airway is secured with an ETT) used for maintenance provides a rapid emergence and recovery.97,98 The rapid return of airway reflexes is particularly important when the dose of opioids must be titrated after extubation.
Children who are scheduled for adenotonsillectomy have a high incidence of airway reactivity and laryngospasm. This will influence the choice of airway management. Placement of an oral RAE or standard uncuffed ETT with a leak at 20 cm H2O (the leak increases with neck extension and insertion of the mouth gag) is generally sufficient to prevent soiling of the trachea during the surgery, yet reduces the incidence of postextubation croup. Cuffed ETTs have become increasingly used in children of all age-groups.99 A cuffed tube prevents an air leak and the consequent bubbling of gases through the oropharyngeal secretions and blood that can interfere with surgery. It also minimizes pollution by anesthetic gases and decreases the risk of an airway fire when electrocautery is used.
Blood and secretions may be present in the oropharynx at the conclusion of surgery and should be carefully suctioned before emergence from anesthesia. Emptying the stomach with an orogastric tube, a maneuver frequently performed by the surgeon under direct vision after completion of surgery, does not reduce the incidence of PONV, although the study was underpowered to detect a difference.100
It is preferable to wait until the child is fully awake and able to clear blood and secretions from the oropharynx before removing the ETT. A common practice is to position the child in the lateral position (known as the “tonsil” or “recovery” position) with the head slightly down at the time of extubation to permit blood and secretions to pool in the dependent cheek and drain out of the mouth rather than accumulate at the laryngeal inlet. Intact airway and pharyngeal reflexes are of utmost importance in preventing aspiration, laryngospasm, and airway obstruction.101 The child should remain in the tonsil position postoperatively, while being carefully observed and monitored during transport to the recovery room.
The use of the LMA for adenotonsillectomy was described in 1990, but it was not until the widespread availability of a model with a flexible spiral, metallic reinforced shaft that it was widely used (E-Fig. 31-1).102,103 The wide, rigid tube of the original model did not fit under the mouth gag and was easily compressed or dislodged during full mouth opening. The newer, flexible model has a soft, reinforced shaft, which easily fits under the mouth gag without becoming dislodged or compressed. Adequate surgical access can be achieved and the airway is reasonably well protected from exposure to blood during the surgery.104,105 Early advantages cited for the LMA over the ETT include a decrease in the incidence of postoperative stridor and laryngospasm, and an increase in immediate postoperative oxygen saturation,106 although recent evidence disputes any difference in the frequency of laryngospasm.107 Insertion of the LMA, however, may be difficult in the presence of tonsillar enlargement, and careful placement to avoid kinking of the LMA is essential.108 Although it has been recommended that the LMA be used only in spontaneously breathing children and that positive-pressure ventilation be avoided, gentle assisted ventilation is both safe and effective if peak inspiratory pressure is limited to 20 cm H2O or less. If there is any leak of gases around the LMA then the potential for an airway fire must be considered and appropriate precautions taken if electrocautery is used.
Analgesic Management
Surgical technique has a major impact on the analgesic requirements after adenotonsillectomy because electrocautery techniques are generally associated with greater pain, presumably owing to increased thermal injury,40,41,109 although this is debated.110 Opioids have been the mainstay of perioperative analgesia. However, because opioids increase the incidence of emesis111 and respiratory morbidity, the use of opioid-sparing adjuncts has been advocated, including dexamethasone, acetaminophen, NSAIDs, and ketamine.
A single intraoperative dose of dexamethasone reduces postadenotonsillectomy pain and edema when electrocautery has been used. Large doses are traditionally used, especially in children with OSA. Dexamethasone (1 mg/kg) administration is associated with reduced parental- and physician-rated pain scores after adenotonsillectomy (Table 31-4).41 The minimum morphine-sparing dose for dexamethasone is reported to be 0.5 mg/kg.112 For dexamethasone doses between 0.0625 mg/kg and 1.0 mg/kg, the frequency of postoperative vomiting, pain scores, and times to first liquid and first analgesics were similar.113 A similar absence of a dose response for dexamethasone between 0.050 and 0.15 mg/kg for vomiting after tonsillectomy was reported in another study.114 Single doses of dexamethasone have not been associated with aseptic necrosis of the hip or infections, but have been responsible for several cases of acute tumor lysis syndrome, including one death.115–117 One study suggested an increased risk of bleeding after tonsillectomy in children who received dexamethasone up to 0.5 mg/kg (maximum 20 mg).118 These findings have been refuted by a recent meta-analysis and several studies.119–122a
TABLE 31-4 Effect of Single Intraoperative Dose of Dexamethasone on Postoperative Pain in Pediatric Tonsillectomy or Adenotonsillectomy: A Comparison of Randomized, Double-Blind Studies
The routine use of NSAIDs for adenotonsillectomy remains controversial because of the potential for postadenotonsillectomy hemorrhage. A meta-analysis of seven randomized controlled trials (505 children) on the effects of NSAIDs on bleeding risk after tonsillectomy reported the number needed to harm, in terms of reoperation for hemostasis, to be 29.123 NSAIDs were associated with a greater risk of both postoperative bleeding that required treatment and reoperation for hemostasis. The Cochrane Collaboration assessed the effect of NSAIDs on bleeding after pediatric tonsillectomy in 13 trials (955 children) and found no increase in bleeding that required reoperation for hemostasis.124 An audit of more than 4800 pediatric tonsillectomies in which the NSAIDs diclofenac and ibuprofen were routinely used, reported a primary hemorrhage rate of 0.9%.55 Because the effects of ketorolac on platelet function are reversible, the effect is dependent on the presence of ketorolac within the body.125 Thus, unlike the effect of aspirin, this effect is short-lived. However, we recommend administering NSAIDs only after consulting with the surgeon and, if in agreement, administering them after hemostasis is achieved.126 Acetaminophen is commonly used as a component of multimodal analgesic approach in these children.127 IV formulations of acetaminophen are now available in many countries, offering the theoretical advantage of greater predictability than the oral and rectal routes. However, recent studies suggest that the duration of analgesia after 15 mg/kg of acetaminophen given IV is less than that after 40 mg/kg given rectally.128 Furthermore, although introduced only 7 years ago, two reports of 10-fold overdoses of IV acetaminophen with near-catastrophic outcomes in infants should alert clinicians to the very serious risk of dosage errors with this medication.129
An IV infusion of dexmedetomidine 2 µg/kg over 10 minutes followed by 0.7 µg/kg/hr combined with an inhalation agent can provide satisfactory intraoperative conditions for adenotonsillectomy without adverse hemodynamic effects. In children with OSA syndrome, postoperative opioid requirements are significantly reduced and the incidence and severity of severe emergence agitation is reduced, with few children desaturating.130 After larger doses of dexmedetomidine (2 and 4 µg/kg), the opioid-free interval increases and the postoperative opioid requirements decrease. However, duration of stay in the PACU is prolonged.131
Infiltration of local anesthetics into the tonsillar fossa during tonsillectomy is sometimes reported to decrease postoperative pain, but the pain relief is transient (E-Fig. 31-2).132 In addition, life-threatening complications have been reported after local anesthetic infiltration in the tonsillar fossa, including intracranial hemorrhage, bulbar paralysis, deep cervical abscess, cervical osteomyelitis, medullopontine infarct, and cardiac arrest. The risks associated with injection of local anesthesia in the tonsillar fossa may outweigh its potential benefits, particularly in inexperienced hands.133,134
Postoperative Nausea and Vomiting
Emesis and poor oral intake are common comorbid conditions after adenotonsillectomy. Opioids increase the incidence of PONV, with two thirds of treated children experiencing PONV.37,55,135 The incidence of PONV increases with morphine dose.111,136 Propofol infusions,136a,136b ondansetron and dexamethasone are widely used to reduce the incidence of emesis after adenotonsillectomy. Postdischarge vomiting continues for days in some children. One study has shown that at-home use of oral ondansetron disintegrating tablets may prevent emesis during the first 3 days after adenotonsillectomy.137 A single intraoperative dose of dexamethasone reduces the incidence of emesis during the first 24 hours after adenotonsillectomy.138 The number of children needed to treat was only four, which means that the use of dexamethasone in four children undergoing adenotonsillectomy results in one less child experiencing PONV. In addition, children who received dexamethasone were more likely than those receiving placebo to advance to a soft diet on postoperative day 1, with a number needed to treat of five. Given the antiemetic and possible morphine-sparing advantages of a single dose of dexamethasone, and its low cost and safety profile, the evidence suggests that routine use of dexamethasone reduces morbidity after adenotonsillectomy in children.41,138 Although the literature supports the effectiveness of a single dose of dexamethasone, the smallest effective dose remains somewhat unclear. One study suggested an IV dose of 0.15 mg/kg,139 whereas another reported no difference in postoperative vomiting, pain scores, time to first liquid, and time to first analgesics between doses of 0.0625 and 1.0 mg/kg (Fig. 31-5).113 Acupuncture, acupressure, as well as therapeutic suggestion, have also been used with variable results.140–142
Special Considerations for Children with OSA
Children with OSA who require premedication should be closely observed, because transient oxygen desaturation has been reported in 1.5% of children with OSA who received 0.5 mg/kg oral midazolam.96
Induction of Anesthesia
Compared with children undergoing adenotonsillectomy for chronic tonsillitis, those whose indication was OSA experienced more respiratory complications during induction of anesthesia.92 The vulnerability of the upper airway musculature described for halothane143 has subsequently been reported for most anesthetic agents, resulting in a graded reduction in airway caliber with increasing anesthetic concentration.144–148 Airway obstruction occurs in the upper two thirds of the pharyngeal airway, and the smallest pharyngeal dimension is in the area of overlap between the adenoids and tonsils.147 During induction of anesthesia, early pharyngeal airway obstruction may require a jaw thrust maneuver, insertion of an oral or nasopharyngeal airway, and the application of continuous positive airway pressure (CPAP). Propofol-associated loss in airway caliber is reversed with the application of CPAP.148 CPAP acts as a pneumatic splint to increase the caliber of the pharyngeal airway (Fig. 31-6).149 Of equal importance, CPAP increases longitudinal tension on the pharyngeal airway, thereby decreasing the collapsibility of the upper airway (see Fig. 12-10), and increases lung volumes.150,151 Small increments in CPAP between 5 and 10 cm H2O increase the dimension of the pharyngeal airway dramatically (Fig. 31-7).152,153 The closing pressure of the pharynx increases with OSA severity, such that greater levels of CPAP are required in children with severe OSA compared with those with mild OSA. It is prudent to consider securing IV access before induction of anesthesia in children with severe OSA, to expedite administration of muscle relaxants or IV agents should pharyngeal obstruction or laryngospasm occur during induction. The small oropharynx and adenotonsillar hypertrophy associated with severe OSA may increase the difficulty in properly inserting an LMA.
Analgesic Management in Children with OSA
Severe OSA is characterized by recurrent episodes of transient hypoxia and hypercarbia during sleep. In animal models, exposure to intermittent hypoxia during development affects the opioid system, increasing the density of µ-opioid receptors in the respiratory-related areas of the brainstem. The cellular mechanism whereby this increased density is achieved has yet to be elucidated, but it may represent an adaptive response to the effects of recurrent intermittent hypoxia that allows µ-receptor–mediated opioid respiratory effects to predominate.154–157
For children with severe OSA, the severity of the nocturnal oxygen desaturation correlates with the sensitivity to exogenously administered opioids (Fig. 31-8).158–160 The morphine dose required to achieve a uniform analgesic endpoint in children with OSA who exhibited a low preoperative oxygen-saturation nadir during sleep (less than 85%) (Fig. 31-9) was less than in those whose preoperative saturation nadir was greater.159 Young age was also associated with an increased sensitivity to opioids. An unforeseen risk of perioperative opioid use in children with severe OSA is that smaller-than-expected doses of opioids may produce exaggerated respiratory depression. Of children with severe OSA who were anesthetized with halothane, 46% experienced apnea after a uniform dose of fentanyl, compared with 4% of controls.161 This increased sensitivity to the respiratory depressant effects of fentanyl in children with OSA is supported by the exaggerated respiratory depression to subsequent administration of a uniform dose of fentanyl in rat pups exposed to intermittent hypoxia.162 Hence, allowing spontaneous respirations during maintenance of anesthesia enables an assessment of the response to small challenges of opioid analgesics. In this manner, the anesthesiologist can assess the sensitivity of the child with OSA to opioids. Controlling respiration precludes such an evaluation. Sleep fragmentation blunts the arousal response to acute airway occlusion during sleep.163,164 In addition, exposure to intermittent hypoxia during development is associated with an increase in the arousal latency to hypoxia.165–167 Morphine acting at the level of the basal forebrain blunts arousal.168 If the increased sensitivity to both the analgesic and respiratory effects of exogenously administered opioids reported in children with OSA extends to arousal mechanisms, the use of opioids in children with severe OSA may further impair arousal mechanisms. Guidelines for the perioperative management of OSA assign a greater risk score if opioids are used for postoperative analgesic regimens in children with OSA.91 Although these guidelines suggest that the use of low-potency oral opioid analgesia carries a reduced perioperative risk, the use of codeine, a “low-risk” oral opioid commonly used in the ambulatory setting, may also be problematic in children with OSA. Codeine is metabolized by the cytochrome P450 debrisoquine 4-hydroxylase (CYP2D6) to its active analgesic metabolites. The CYP2D6 gene displays polymorphism, including gene duplication (ultra-rapid metabolizers) and inactive genes. Gene duplication may lead to ultra-rapid metabolism, which for prodrugs, such as codeine, might yield a 50% greater fraction of morphine and its glucuronides compared with extensive metabolizers.169 Respiratory arrest after codeine has been reported in both adults and children who demonstrate ultra-rapid metabolism of codeine.170–171a Whereas the ultra-rapid metabolizing genotype is present in 3% of Caucasians, it is present in 10% to 30% of Arabian and Northeast African populations. In contrast, almost 10% of children lack CYP2D6, rendering codeine an ineffective analgesic. Given the broad variability in codeine metabolism and our lack of knowledge of which polymorphism is carried by each child, the use of codeine and the dose prescribed for children with OSA must be very carefully considered or an alternate opioid selected.
Neural Blockade
Blockade of neural input to the upper airway dilator musculature in children with OSA is also problematic. Serious life-threatening complications, including severe UAO and pulmonary edema, have been reported after local anesthetics have been infiltrated in the tonsillar fossa to prevent pain after adenotonsillectomy. The pharynx in children with OSA is not only smaller in size,147,172 but also more collapsible, even during wakefulness, compared with those children who do not have OSA.173–175 Topical anesthesia applied to the mucosa of the pharynx of children with OSA reduces the caliber of the pharynx compared with control subjects.176
Extubation Strategy and Management of the Postoperative Period in Children with OSA
Extubation of the trachea is usually performed when the child is fully awake. Techniques that involve minimal stimulation of the airway have been suggested.101 Although a minority of children receive muscle relaxants for adenotonsillectomy, residual neuromuscular blockade in the recovery room will selectively depress the function of the upper airway dilators relative to the diaphragm, promoting collapse of the pharyngeal airway.177 Full antagonism of neuromuscular blockade is strongly recommended before extubating the tracheas of children with OSA.91 Antagonism of neuromuscular blockade with atropine and neostigmine after tonsillectomy has been associated with less PONV than antagonism with glycopyrrolate and neostigmine.178
Several other factors may increase the risk of respiratory difficulties after adenotonsillectomy in children with OSA. Otherwise healthy children with severe OSA, whose adenotonsillectomy is performed in the morning, are less likely than those whose surgery is performed in the afternoon to desaturate when managed in a PACU setting.179 In addition, meticulous attention to the position of the head and neck is required during recovery from anesthesia, because hypercarbia and a loss of lung volume (functional lung capacity) both promote collapse of the pharyngeal airway.151,180,181 Extension of the cervical spine, the sniffing position, the lateral recovery position, and mouth opening with anterior advancement of the mandible all increase the dimension of the pharynx182–186 and reduce the risk of UAO.
Two drugs, atropine and naloxone, have the potential to augment the function of the upper airway. Atropine administered after induction of anesthesia decreased the risk of postadenotonsillectomy respiratory complications.70 Of possible relevance is the report that muscarinic blockade of the hypoglossal nucleus in the rat model enhances activity of the genioglossus muscle.181 Agonists of opioid µ-receptors have been shown to depresses activity in the pharyngeal dilator muscles, including the genioglossus muscle.155,187–189 Given the increased sensitivity to both analgesic and respiratory effects of exogenously administered opioids in children with severe OSA, a similar sensitivity may also apply to the respiratory-related activity of the pharyngeal musculature. Small doses of naloxone may alleviate UAO after adenotonsillectomy if exogenous opioids have been administered.
The severity of OSA is a predictor of the outcome after adenotonsillectomy.190 A preoperative RDI above 19 may predict an RDI in excess of 5 in long-term follow-up.75,93 Children with OSA continue to demonstrate obstructive apnea and desaturation during sleep on the first night after adenotonsillectomy, with the frequency of the obstructive events and the severity of desaturation usually greater in those children with severe OSA (Fig. 31-10).191,192 Thus despite removal of the hypertrophied tonsils and adenoids, children with OSA continue to experience symptoms on the first postoperative night. This underscores the need to admit these children to a hospital for continuous overnight monitoring postoperatively, rather than discharge them home. Long-term follow-up studies more than 6 months after tonsillectomy in children with OSA show that symptoms completely resolve in those with mild OSA (AHI less than 10) but are persistent in 35% of those with severe OSA (AHI greater than 20).190 Furthermore, recent epidemiologic evidence suggests that residual sleep-disordered breathing is more likely to be present after adenotonsillectomy in older children (more than 7 years of age) and obese children. It has also been suggested that obese children with large tonsils and OSA also show evidence of systemic inflammatory disease that persists after the tonsillectomy.190,193
Measures to support airway patency in the postoperative period have included insertion of nasal airways, administration of noninvasive ventilatory support (e.g., CPAP), reintubation, ventilation, and the administration of bronchodilators, racemic epinephrine, and heliox. Bilevel positive airway pressure and/or CPAP may be useful in children with preexisting neurologic disorders.194 However, nasal secretions may be copious after adenotonsillectomy, limiting the efficacy of noninvasive ventilatory support. Children with complex medical diseases, who are critically dependent on the function of upper airway musculature, may benefit from delayed extubation. Acute relief of chronic UAO favors the exudation of intravascular fluid into the pulmonary interstitium and noncardiogenic pulmonary edema, which may present preoperatively, intraoperatively, and postoperatively. Supportive measures include the administration of oxygen, endotracheal intubation, mechanical ventilation with positive end-expiratory pressure, and administration of furosemide.195–197
Discharge Policy for Ambulatory Adenotonsillectomy
Children younger than 3 years of age and those with complex medical disorders are not candidates for adenotonsillectomy as outpatients.38,198 Although children undergoing adenotonsillectomy for obstructive breathing without apnea may undergo ambulatory surgery, those with OSA should not. A diagnosis of OSA increases the likelihood of respiratory complications after adenotonsillectomy from 1% in otherwise healthy children to 20% in those with OSA.37
The majority of children who are scheduled for adenotonsillectomy have symptoms of obstructive breathing,42,199,200 yet only 55% with clinical criteria suggestive of OSA subsequently meet sleep laboratory criteria for OSA.201 Sleep screening of children undergoing routine adenotonsillectomy for chronic tonsillitis revealed unexpectedly that 20% had severe obstructive episodes associated with desaturation.202 Because only a minority of children undergoing adenotonsillectomy undergo diagnostic testing for sleep-disordered breathing, the recently published guidelines on management of OSA have empowered clinical diagnostic criteria, such that a child with severe symptoms must be assumed to have moderate to severe OSA until proven otherwise by sleep laboratory testing (see Fig. 31-4). Ambulatory programs may now find it cost effective to screen children with a positive clinical history.
In otherwise healthy children, conversion from ambulatory to inpatient status was most frequently prompted by respiratory events in children whose indication for surgery was obstructive breathing.42 A systematic reduction in postoperative morphine use was associated with a reduced rate of hospital admission from 8% to 2.4%.55 Same-day discharge, which abbreviates the postoperative stay in hospital and the minimum period of observation before discharge from hospital, has been the subject of much debate. Because the onset of respiratory complications in these children may be delayed,69,179,191 a 6- to 8-hour period of observation for respiratory complications after adenotonsillectomy for OSA has been suggested. However, this extended period of observation does not preclude the delayed onset of sleep-related respiratory compromise after adenotonsillectomy. Table 31-5 presents common admission criteria for children undergoing elective tonsillectomy.
TABLE 31-5 Criteria for Overnight Admission after Tonsillectomy and Adenoidectomy
From Zalzal G: Personal communications, survey of major pediatric hospitals, 2006.
Post-Tonsillectomy Bleeding
Post-tonsillectomy bleeding is a surgical emergency. This can occur either within the first 24 hours (primary) or 5 to 10 days after surgery when the eschar covering the tonsillar bed retracts (secondary). Approximately 75% of postoperative tonsillar bleeding occurs within 6 hours of surgery. Sixty-seven percent of cases of postoperative bleeding originate in the tonsillar fossa, 27% in the nasopharynx, and 7% in both.203 Primary bleeding is typically more serious than secondary bleeding because it is usually more brisk and profuse. It is considered a surgical complication that is responsible for converting tonsillectomy from ambulatory surgery to a hospital admission in 1.6% of cases.42
In a review of more than 9000 adenotonsillectomies in children performed with blunt and sharp (cold) dissection, the incidence of postoperative bleeding was 2.15%, with 76% of the hemorrhages occurring in the first 6 hours postoperatively.204 The authors of an audit of 4800 pediatric tonsillectomies for which hemostasis was secured with electrocautery (hot) techniques, reported a primary postoperative hemorrhage rate of 0.9%, with 83% presenting within 4 hours of surgery.55 The consensus is that the period of observation for primary hemorrhage depends on the surgical technique: 6 hours and 4 hours, for cold and hot dissection, respectively,55,203,204 although abbreviated periods of observation have been advocated by some.42
The management of anesthesia in this situation can be challenging even in the hands of an experienced pediatric anesthesiologist.205 It often requires dealing with anxious parents, an upset surgeon, and a frightened anemic, hypovolemic child with a stomach full of blood. A thorough review of the anesthetic record of the original surgery will provide pertinent information about any existing medical condition, use of medications (such as aspirin), difficulty with airway management, and a rough estimate of intraoperative blood loss and fluid replacement, as well as the duration of known bleeding and the volume of blood vomited since the bleeding began. A quick history and examination of the child will provide vital information about the child’s current volume status. A history of dizziness and the presence of orthostatic hypotension may suggest a loss of more than 20% of the circulating blood volume and the need for aggressive fluid resuscitation and crossmatch of blood before induction.206 Even when severe hypotension is not present, the child with the bleeding tonsil is hypovolemic and has a decrease in cardiac output secondary to ongoing blood loss. If blood loss is severe, and/or fluid resuscitation is not vigorous, lactic acidosis and an eventual state of shock will develop. The compensatory response to acute blood loss is an outpouring of catecholamines. This causes peripheral vasoconstriction, which delays the clinical onset of hypotension in the awake child. When anesthesia-induced vasodilation occurs, profound hypotension may develop. Vigorous fluid resuscitation with crystalloids (repeated boluses of 20 mL/kg of balanced salt solution) and/or colloids is therefore the key to improve the cardiac output and achieve hemodynamic stability before induction of anesthesia. Hemoglobin or hematocrit determination should be interpreted in light of the child’s volume status and the type of fluid resuscitation administered. If the hemoglobin concentration is low, blood may be required; however, blood is rarely the primary solution for volume replacement in these children. If severe hypovolemia is suspected or if there may be a delay in obtaining blood, blood should be crossmatched for two or more units of packed red blood cells before the child reaches the OR. If a child bleeds after the tonsillectomy, and a bleeding blood vessel is not identified, it may be necessary to measure the prothrombin time, partial thromboplastin time, platelet count, and a bleeding time to rule out a bleeding diathesis. It cannot be overemphasized that the child must be adequately volume resuscitated before proceeding to the OR.
A child who presents with a bleeding tonsil has a full stomach (filled with swallowed blood) and may still be hypovolemic. A child who is spitting bright red blood may quickly exsanguinate, but the bleeding may be temporarily controlled by compression of the carotid artery ipsilateral to the bleeding source. The anesthesiologist may have difficulty visualizing the larynx because of the bleeding tonsillar bed and clots in the pharynx. A styletted ETT, two sets of well-illuminated laryngoscope blades and handles, and two large-bore Yankauer-type suction tubes must be available before induction of anesthesia (see also Chapter 4 and Fig. 38-5). On arrival in the OR and application of routine monitors, the child should be preoxygenated while positioned in the left lateral position and head down to drain blood out of the mouth (Fig. 31-11). The child is then turned supine, and a rapid-sequence induction is carried out with cricoid pressure (Sellick maneuver) applied by an assistant, to minimize the risk of aspirating blood into the lungs.207 There is no evidence that a rapid-sequence induction with cricoid pressure decreases the risk of aspiration in children with full stomachs, although this practice is commonplace. It should also be recognized that aspiration of blood into the lungs is not synonymous with acid particulate aspiration, unless the volume of blood aspirated compromises pulmonary oxygenation. The use of a full induction dose of propofol in a hypovolemic child could result in significant hypotension. A reduced dose of these induction agents (e.g., propofol, 1 to 2 mg/kg), or ketamine (1 to 2 mg/kg) or etomidate (0.2 mg/kg) for induction followed by atropine (0.02 mg/kg) combined with succinylcholine (1.5 to 2 mg/kg) or rocuronium (1.2 mg/kg) for tracheal intubation should facilitate a rapid control of the airway without hypotension. However, the systolic blood pressure after induction of anesthesia will provide a direction indication of the volume status of the child.
When possible, a cuffed ETT (one-half size smaller than usual for age or weight) should be used to minimize the chance of aspirating blood. The use of a stylet is strongly recommended in spite of a previous history of easy intubation. Titration of an inhalational anesthetic, such as sevoflurane or desflurane with nitrous oxide and oxygen,97 supplemented with an opioid, such as fentanyl, 1 to 2 µg/kg, will facilitate rapid recovery at the end of surgery.208 Often these surgeries are not excessively painful because surgery is limited to the area of bleeding. Controlling the bleeding vessel in the tonsillar bed can be accomplished rapidly if the blood pressure is maintained in the normal range. Hence, these surgeries are often quite brief and the anesthetic should be planned accordingly. Suctioning the stomach with a large-bore catheter under direct vision after the procedure does not guarantee an empty stomach, because much of the blood may be clotted and the clots are often too large to be suctioned. The use of prophylactic antiemetic therapy (e.g., ondansetron 0.1 mg/kg) is indicated.
Peritonsillar Abscess
Peritonsillar abscess (quinsy tonsil) occurs in older children and young adults. It is the most common deep neck-space infection treated by otolaryngologists. Infection originates in the tonsil and spreads to the peritonsillar space between the tonsillar capsule and the superior constrictor muscle, and usually into the soft palate in the region of the superior pole of the tonsil. Commonly cultured organisms include aerobes, such as Streptococcus pyogenes, S. milleri, S. viridans, β-hemolytic streptococci, Haemophilus influenzae, as well as anaerobes, such as Fusobacterium and Prevotella species.209
Preoperative evaluation includes careful assessment of the airway, with special emphasis on the degree of trismus. Blood specimens should be analyzed for total and differential white blood cell count to ascertain the response to the infection, and for cultures for appropriate antibiotic therapy. Computed tomography of the tonsillar area will identify airway deviation or compromise and the extent of spread of the abscess (Fig. 31-12).
While awaiting the results of the cultures, treatment should begin with establishing IV access, hydration, and appropriate antibiotic coverage. The majority of organisms, including anaerobes, are penicillin sensitive. Consequently, penicillin is usually the antibiotic of choice.209 The three different procedures currently used to drain a peritonsillar abscess are needle aspiration, incision and drainage, and abscess tonsillectomy.210 Most children undergo general anesthesia for treatment of peritonsillar abscess by incision and drainage, although in some centers, moderate to deep sedation has been successfully used.211 If the abscess is small and well confined, immediate tonsillectomy is performed.
These children are often older and do not require preoperative sedation. If trismus is present, an inhalational induction should be performed, using sevoflurane and oxygen while the anesthesiologist assesses mobility of the temporomandibular joint under anesthesia. An oropharyngeal airway is best avoided, lest the abscess is traumatized. Usually, awake trismus resolves once an adequate depth of anesthesia has been achieved. When this is confirmed, or if there was minimal trismus to begin with, then a short-acting muscle relaxant (or propofol) should be given to facilitate tracheal intubation. Alternatively, if there is minimal trismus and the preoperative airway assessment indicates minimal distortion, a rapid-sequence IV induction after adequate preoxygenation may be the best way to avoid trauma to the pharyngeal structures while struggling with a mask induction, needing to insert an oropharyngeal airway, and possibly rupturing the abscess.2
To avoid aspiration of purulent material during intubation and drainage, a cuffed ETT is recommended and the child is placed in Trendelenburg position. At the end of surgery, the child should be extubated awake, preferably in the lateral decubitus position.2
Anesthesia for Endoscopy
Anesthesia for rigid bronchoscopy in young children presents a significant challenge. Not only does the child have a compromised airway, but we also must share it with the surgeon. The importance of constant communication between the endoscopist and the anesthesiologist cannot be overstated. In general, the goals of anesthesia for endoscopy are analgesia, an unconscious child, and a quiet surgical field.212 Coughing, bucking, or straining during instrumentation with a rigid bronchoscope may cause difficulty for the surgeon and damage the child’s airway. At the conclusion of the procedure, children should be returned to consciousness quickly with airway reflexes intact to protect the recently instrumented airway. General principles for the anesthetic management will be outlined first. Disease-specific requirements will be discussed under appropriate subheadings.
For most children, a pulse oximeter, blood pressure cuff, electrocardiogram, and precordial stethoscope are applied before induction. Continuous monitoring of ventilation by capnography is not always possible during bronchoscopy, particularly when the Hopkins optical telescope is in place for optimal viewing. Clinical observation of the chest wall movement and the use of a precordial stethoscope are useful. In many cases, intermittent capnography is possible when the bronchoscope is withdrawn by the surgeon. Although greater than normal CO2 tensions are inevitable with intermittent ventilation, they are generally well tolerated in the presence of sevoflurane. In contrast, if halothane is used, ventricular arrhythmias may occur and should be treated by hyperventilation and deepening halothane anesthesia, or by substituting isoflurane or sevoflurane for halothane (see Chapter 6).213 Hypoxia, on the other hand, is not well tolerated, and the procedure should be stopped while the child is oxygenated.
Diagnostic Laryngoscopy and Bronchoscopy
Although diagnostic laryngoscopy and bronchoscopy procedures are usually of brief duration, the anesthetic management can be challenging in small infants with an already compromised airway. Stridor, or noisy breathing due to obstructed airflow, is a common indication for a diagnostic laryngoscopy and bronchoscopy in infants and children. Inspiratory stridor results from UAO, expiratory stridor results from lower airway obstruction, and biphasic stridor is present with mid-tracheal lesions (see Chapters 11 and 12). Subglottic stenosis may follow prolonged tracheal intubation in an infant that was born preterm.
The evaluation of a child with stridor begins with taking a thorough history. The age at symptom onset helps suggest a cause; for instance, laryngotracheomalacia and vocal cord paralysis are usually present at or shortly after birth, whereas cysts or mass lesions develop later in life (Table 31-6). Information indicating positions that make the stridor better or worse should be obtained, because placing a child in a position that allows gravity to aid in reducing obstruction can be of benefit during induction.
Laryngomalacia is the most common cause of stridor in infants and most often results from a long epiglottis that prolapses posteriorly and prominent arytenoid cartilages with redundant aryepiglottic folds that prolapse into the glottic opening during inspiration.214 The definitive diagnosis is obtained by direct laryngoscopy and by rigid or flexible bronchoscopy.
After completion of pharyngoscopy and/or laryngoscopy, the surgeon generally proceeds to rigid bronchoscopy. The size of a rigid bronchoscope refers to the internal diameter (ID). Because the external diameter may be significantly greater than that of an ETT of similar size, care must be taken to select a bronchoscope of proper external diameter, to avoid damage to the laryngeal structures (Table 31-7). The rigid bronchoscope can be used for ventilation through the side port attached to the anesthesia circuit with a flexible extension. It is often most useful to paralyze the child with a fixed lesion, which diminishes the risk of vocal cord injury secondary to movement. For nonfixed lesions, such as an aspirated foreign body, and for assessment for bronchomalacia or tracheomalacia, it is preferable to proceed with spontaneous ventilation, a deep level of anesthesia, and good topical anesthesia of the vocal cords and carina. Adequate oxygenation should be maintained in these infants throughout the procedure. Because ventilation may be intermittent and at times suboptimal, it is recommended that 100% oxygen be used as the carrier gas during the bronchoscopic examination. During ventilation of the infant with the optical telescope in place, high resistance may be encountered as a result of partial occlusion of the lumen. This is especially likely when the 2.5-, 3.0-, and 3.5-mm ID scopes are used. Large fresh-gas flow rates, large tidal volumes with high inflation pressures, and large concentrations of inspired inhalational anesthetic (or TIVA) are often necessary to compensate for leaks around the ventilating bronchoscope and the high resistance encountered when the optical telescope is in place. Hand ventilation at greater than normal rates is most effective in achieving adequate ventilation. Sufficient time for exhalation must be provided for passive recoil of the chest. In small infants, there may be room for only the optical telescopic light source, which does not have a ventilation channel. In these cases, insufflation of oxygen via a small tube placed in the hypopharynx via the nose or mouth will delay the onset of desaturation in a spontaneously breathing child. If (when) desaturation occurs, the surgeon must stop and allow the child to be oxygenated before continuing with the examination.
TABLE 31-7 External Diameter of Standard Endotracheal Tube versus Rigid Bronchoscope
Internal Diameter (mm) | External Diameter (mm) | |
---|---|---|
Endotracheal Tube* | Rigid Bronchoscope† | |
2.0 | 2.9 | |
2.5 | 3.6 | 4.2 |
3.0 | 4.3 | 5.0 |
3.5 | 4.9 | 5.7 |
3.7 (bronchoscope) | 6.3 | |
4.0 | 5.6 | 6.7 |
5.0 | 6.9 | 7.8 |
6.0 | 8.2 | 8.2 |
*Mallinckrodt Medical, Inc., St Louis.
†Karl Storz Endoscopy-America, Inc., El Segundo, Calif.
At the conclusion of bronchoscopy, the surgeon may wish to determine the size of the larynx and determine the degree of airway narrowing. An uncuffed ETT is inserted beyond the narrowest portion of the obstructed airway, and the airway is assessed by applying positive pressure between 10 and 25 cm H2O to the airway and listening with a stethoscope for an air leak around the ETT at the level of the suprasternal notch. The outer diameter of the appropriate ETT is compared with the inner diameter of the child’s larynx and trachea, and the percentage of obstruction is calculated. Grade I obstruction involves up to 50% of the airway, grade II is from 51% to 70%, and grade III is greater than 70% (Fig. 31-13, B).215
An alternative method of ventilation during bronchoscopy is the Sanders jet ventilation technique. The principle of jet ventilation involves intermittent bursts of oxygen delivered at a maximum pressure of 50 psi from a hand-regulated pressure-reducing valve to the lungs, through a 16-gauge catheter attached to a rigid bronchoscope.216 Current jet ventilators include adjustable pressure-control valves that permit attenuation of the peak pressure, a desirable feature if this device is to be used in a child. Intermittent flow is accomplished by depressing the lever of an on-off valve. A jet of oxygen is released at the tip of the 16-gauge catheter, creating a Venturi effect that entrains room air into the bronchoscope. This jet of oxygen and room air mixture allows inflation of the lungs to occur. Exhalation is passive and depends on the recoil of the chest wall. Although this technique is usually effective for both oxygenation and ventilation in experienced hands, a number of potential problems exist. Because of potentially high inflation pressure, pneumothorax, pneumomediastinum, and death can occur.217 Blood or infectious or particulate matter in the airway may be forced distally by high-pressure bursts. There is also the possibility of hypoxemia in some children, because the high-pressure oxygen entrains room air, diluting the oxygen.
High-frequency jet ventilation is also possible for upper airway endoscopy and laryngotracheal surgery. Obstruction to expiratory flow is a major concern and is dependent on good positioning of the rigid laryngoscope. Complications, such as barotrauma, pneumopericardium, CO2 retention, necrotizing tracheobronchitis, and gastric rupture, dictate a fastidious technique.218
Upper Airway Obstruction
Laryngotracheobronchitis (CROUP)
Croup is a symptom complex of inspiratory stridor; suprasternal, intercostal, and substernal retractions; barking cough; and hoarseness that results from swelling of the mucosa in the subglottic area of the larynx.2 There are two common entities that account for most cases of croup: spasmodic croup, and laryngotracheobronchitis. Spasmodic croup has been diagnosed in about 3% of children with stridor.219 The child is otherwise healthy and afebrile, presenting with nocturnal episodes of spasmodic cough, which is described as barking and high pitched. The disease is self-limiting. Besides viruses, allergic and psychological factors are blamed for this acute phenomenon. It differs from acute laryngotracheitis in that it is considered an allergic reaction to viral antigens rather than a true infection with the viruses.220 Besides lack of fever, spasmodic croup is usually remarkable for lack of severe laryngeal inflammation, and, in general, supportive therapy on an outpatient basis is all that is required.
Viral laryngotracheitis is by far the most common form of infectious croup. The disease has a gradual onset, usually after a URI in a young child. Low-grade fever is common. Children who have more than two episodes of croup requiring hospitalization should be evaluated for subglottic narrowing from stenosis or cysts. Clinical scoring systems based on objective criteria are helpful in following the progress of the disease and in judging the effectiveness of therapy (Table 31-8).221
Anteroposterior radiographs of the neck will confirm the diagnosis and rule out acute epiglottitis or the possibility of a foreign body in the airway (Table 31-9).222 The viral infection affects the subglottic region of the larynx, causing edema. The characteristic radiograph of croup, therefore, includes blurring of the tracheal air shadow on lateral neck films, and symmetrical narrowing of the subglottic air shadow, described as “church steeple” or “sharpened pencil” sign on anteroposterior films (Fig. 31-14 and E-Fig 31-3). The lateral neck radiographs show normal supraglottic structures and normal epiglottic shadow.
Croup* | Epiglottitis | |
---|---|---|
Incidence | More common | Less common |
Obstruction | Subglottic | Supraglottic |
Age | Younger (<3 years) | Older (3-6 years) |
Etiology | Viral | Bacterial |
Recurrence | Possible (5%) | Rare |
Clinical Features | ||
Onset | Gradual (days) | Sudden (hours) |
Fever | Low grade | High |
Dysphagia | None | Marked |
Drooling | None | Present |
Posture | Recumbent | Sitting |
Toxemia | None | Present |
Cough | Barking | Usually none |
Voice | Hoarse | Clear to muffled |
Respiratory rate | Rapid | Normal/slow |
Larynx palpation | Not tender | Tender |
Leukocytosis | + (Lymphocytic) | +++ (Polymorphonuclear cells) |
Neck radiographs | Anteroposterior: steeple sign | Lateral: thumb-like mass |
Clinical course | Longer | Shorter |
Treatment | ||
Primary therapy | Medical and supportive | Secure airway first |
O2 and humidity | Essential | Usually desirable |
Hydration | Oral or IV | Intravenous |
Racemic epinephrine | Usually effective | No value |
Corticosteroids | Controversial | Not indicated |
Antibiotics | Not indicated | Effective |
Airway support | Occasionally needed (<3%) | Always indicated (100%) |
Preferred airway | Nasotracheal | Nasotracheal |
Tracheostomy (rarely) | ||
Extubation | 4-7 days | 1-3 days |
*Foreign bodies in the airway should also be considered.
From Hannallah R. Epiglottitis. In: Stehling L, editor. Common problems in pediatric anesthesia. 2nd ed. St Louis: Mosby-Year Book; 1992, p. 277-81.
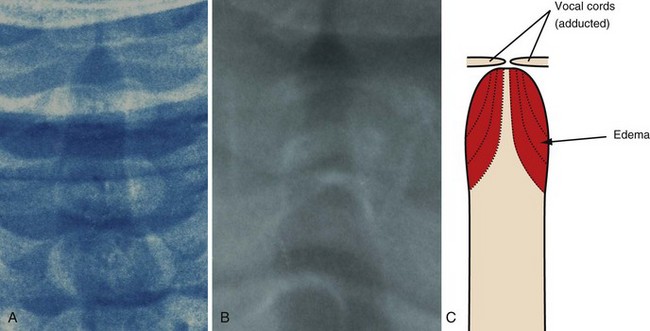
FIGURE 31-14 A, Radiograph of the normal upper airway (anteroposterior view). Note that the subglottic area is rounded. B, Laryngotracheobronchitis (croup) produces swelling (edema and inflammation), which obliterates the normal rounded subglottic area, producing the so-called sharpened pencil or steeple sign. C, Schematic representation showing progressive swelling of the subglottic area. For an additional view, see E-Figure 31-3.
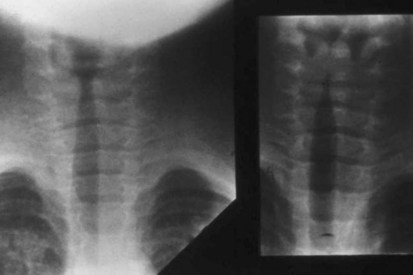
E-FIGURE 31-3 Additional view of normal upper airway (left) and a child with laryngotracheobronchitis and the “steeple” or “sharpened pencil” sign (right) (see Fig. 31-14).
The majority of cases will resolve quickly with simple conservative measures, such as breathing humidified air or oxygen. Less than 10% of cases require hospitalization because of significant respiratory difficulty, and fewer still require an artificial airway.214 Humidification of inspired gases is usually effective in improving respiratory distress, and it prevents drying of secretions, although despite the popularity of cool mist therapy, it is not evidence-based practice.223 Oxygen is obviously essential to prevent or to treat hypoxemia, which may result from ventilation-perfusion mismatching caused by accumulation of secretions. Hydration prevents thickening of tracheal secretions.
Racemic epinephrine is the most effective drug therapy for these children, although l-epinephrine has also been effective. Racemic epinephrine is available as a 2.25% solution, which is diluted in water or saline, and administered either by intermittent positive-pressure ventilation via a face-mask or nebulization.224 Nebulized racemic epinephrine is administered in cases of mild-to-moderate obstruction. The solution is prepared by diluting a volume of 2.25% racemic epinephrine in 2 mL of saline or sterile water according to the child’s weight in kilograms (i.e., 0.25 mL of racemic epinephrine for 0 to 20 kg, 0.5 mL for 20 to 40 kg and 0.75 mL for greater than 40 kg).225 Because the duration of action of racemic epinephrine is brief, rebound edema may occur. Treatments are required every 1 to 2 hours, and the child should be observed for at least 2 hours after treatment. For l-epinephrine, the volume of a 1% solution is the same as that for racemic epinephrine.
If treatment with racemic epinephrine is unsuccessful, in addition to edema, the underlying problem may be obstruction caused by thick, inspissated secretions possibly related to bacterial superinfection, such as bacterial tracheitis.226,227 In this situation, or if the child appears exhausted from the increased work of breathing, relief of the obstruction must be obtained through endotracheal intubation, followed by pulmonary suctioning. The clinical assessment of “exhaustion” in children with croup may be difficult. An alternative approach is to consider intubating the tracheas of those children who have arterial oxygen saturation less than 90% when breathing air, despite nebulized epinephrine and steroid treatment. Laryngotracheobronchitis is also a disease of the lower airways. An inability to clear secretions contributes to atelectasis and arterial oxygen desaturation. Intubation is often required to allow suctioning of the copious yellow secretions.
One large series (512 consecutive admissions in a single year) reported that approximately 6% of children who had sternal and chest retractions on admission and failed to respond to conventional medical therapy required endotracheal intubation.228 Intubation should be performed in the OR under controlled anesthetic conditions, as for a child with severe epiglottitis. The tracheal tube selected should be at least one-half size smaller (0.5 mm ID) than would normally be chosen, to avoid aggravating the subglottic edema and possibly causing subglottic stenosis.229–231 Children whose airways have been intubated are admitted to the ICU, and special care is provided for suctioning of inspissated secretions. The tracheal tube usually remains in place for 3 to 5 days.
Corticosteroid therapy for laryngotracheobronchitis has become the standard of care.232 Single-dose corticosteroid therapy appears safe and effective,233 although large studies of the risk of progression of viral infection or the development of secondary bacterial infections in moderate to severe laryngotracheobronchitis are lacking; reports suggest that IV dexamethasone (0.5 to 1 mg/kg) may be effective.234 There is evidence that there is rapid clinical improvement 12 and 24 hours after steroid treatment, which significantly reduces the incidence of endotracheal intubation.235–237 Antibiotics are generally not indicated in the treatment of uncomplicated viral croup.
Acute Epiglottitis
Although rarely seen today, acute epiglottitis can be fatal because it can produce seemingly unprovoked sudden and complete airway obstruction. It is a clinical and pathologic entity that should more correctly be termed supraglottitis, because the arytenoids and aryepiglottic folds, as well as the epiglottis itself, are usually affected. All supraglottic structures become swollen and stiffened by inflammatory edema. Although the main focus of infection is the oropharynx, the disease produces a generalized toxemia. Epiglottitis is most common between the ages of 3 and 5 years, but it can occur in any age; historically, the causative organism was typically Haemophilus influenzae type B.2,238–240 However, with the widespread use of H. influenzae vaccination, the incidence of epiglottitis in children has all but disappeared in medically advantaged countries.241,242 Streptococcus,243 Staphylococcus,244 Candida,245 and other fungal pathogens246 have become more frequent causes of this now rare disorder in children, although the incidence in adults has not diminished substantially.247 It should be noted that vaccine failures may occur or parents may refuse proper immunizations of their child, resulting in susceptibility to H. influenzae type B infection.248,249 Epiglottitis has become more of a disease of adults.250
The onset is usually abrupt, with a brief history of high fever, severe sore throat, and difficulty in swallowing. Stridor, if present, is usually inspiratory, and because the subglottic structures are usually unaffected, there is little or no hoarseness. An expiratory snore, rather than inspiratory stridor can often be heard. The child appears toxic, and, in an attempt to improve airflow past the swollen epiglottis, assumes the sitting position, leaning forward in the sniffing position (E-Fig. 31-4). The mouth is open, with the tongue protruding. The child frequently drools because of difficulty and pain on swallowing. The mnemonic SNORED is often useful for diagnosis: Septic, NO cough, Rapid onset, Expiratory snore, Drool.
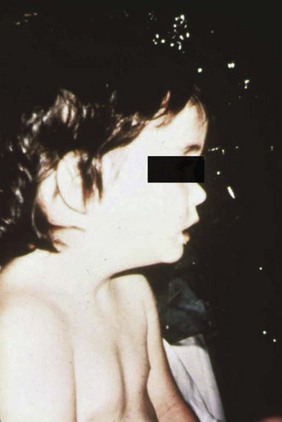
E-FIGURE 31-4 Clinical appearance of a child with epiglottitis; this child is sitting upright, leaning forward, and drooling.
Acute epiglottitis is a clinical diagnosis. It must remain prominent in the differential diagnosis of a child presenting with signs and symptoms of UAO. However, in some early cases, the clinical presentation alone may be inconclusive. If so, a lateral radiograph of the neck will usually demonstrate a swollen epiglottis and aryepiglottic folds (Fig. 31-15 and E-Fig 31-5). The vallecula may be obliterated, but subglottic structures are usually clear. A physician capable of establishing an airway should always be in the child’s attendance (especially if in a remote area of the hospital, such as Radiology), because total airway obstruction can develop during the radiologic examination, especially if the child is forced to lie supine. This is one reason why the use of lateral neck radiographs in the differential diagnosis of UAO is avoided. Examination of the pharynx and larynx should only be attempted in an area with adequate equipment and staff prepared to intervene should UAO develop; ideally, the OR. The safest, most conservative approach to the management of acute epiglottitis is to establish an artificial airway as soon as the diagnosis is made, and then, with the airway secured, to proceed with appropriate antibiotic and supportive therapy. The child should remain in the sitting position at all times and never forced into the supine position. No attempts to examine the larynx should be made in the emergency room.2
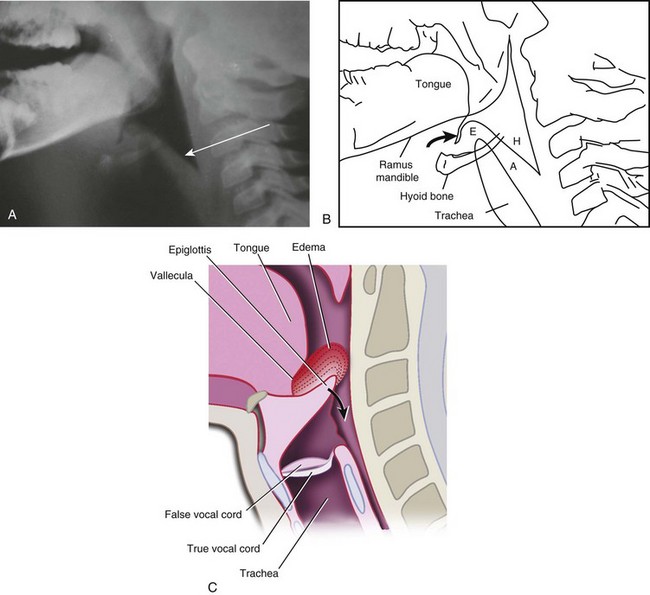
FIGURE 31-15 A, Lateral neck radiograph of child with epiglottitis. Note the marked thickening of the aryepiglottic folds (arrow). B, Schematic representation of A. Note the marked thickening of the aryepiglottic folds (A), loss (“amputation”) of the vallecula (curved arrow), swelling of the epiglottis (E ), and distention of the hypopharynx (H). C, Schematic representation of epiglottitis demonstrating progressive swelling of the lingual surface of the epiglottis, resulting in “amputation” of the vallecula. Progressive swelling leads to trapdoor-like occlusion of the glottic opening (curved arrow). For additional views, see E-Figure 31-5.
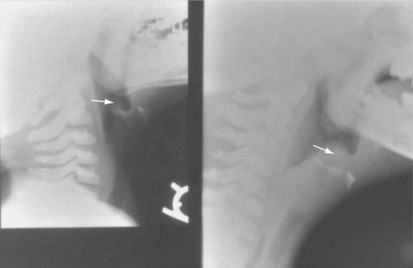
E-FIGURE 31-5 Additional view of child with epiglottitis. Arrow points to the swollen epiglottis, known as the “thumb sign” (see Fig. 31-15).
When a surgical stage of anesthesia is achieved, IV access is established and secured. A large fluid bolus of balanced salt solution is infused (20 to 30 mL/kg) because these children are often dehydrated and will require a deep plane of anesthesia to permit tracheal intubation while spontaneous respirations are preserved. Some anesthesiologists would also administer an antisialagogue to reduce secretions. Epiglottitis is marked by progressive swelling of the lingual surface of the epiglottis with resultant obliteration of the vallecula (see Fig. 31-15). Viewing the glottic opening without traumatizing the epiglottis may usually be accomplished by forcing the tip of the laryngoscope blade along the center of the base of the tongue into the vallecula, where the vallecula has been obliterated by the swollen lingual surface of the epiglottis (see Fig. 31-15, C). Lifting the base of the tongue, without directly touching the epiglottis, can then expose the glottis. A stylet within the ETT may be helpful because it provides increased rigidity to facilitate introduction through a partially obstructed glottic aperture. The size of the ETT should be one-half size smaller (0.5 mm ID) than otherwise selected for the same age child. By choosing an ETT with a smaller ID than usual, one also lessens the risks of pressure necrosis on the mucosa. Figure 31-16 illustrates a case of severe epiglottitis (before [A] and after [B] the airway was secured). A styletted orotracheal tube is inserted first, and may be replaced by a nasotracheal tube of appropriate size. However, if the glottic opening was difficult to visualize, then no attempt should be made to replace the oral tube with a nasal one. The orotracheal tube may displace supraglottic edema, improving the view of the glottic opening and facilitating placement of the nasotracheal tube. An air leak at 20 to 25 cm of H2O, when present, confirms the selection of an appropriate size tube. Because the airway obstruction in epiglottitis is supraglottic, not subglottic, the nasotracheal tube size is often the usual size or one-half size smaller for the child’s age. A larger tube is not necessary and may contribute to the possible development of serious laryngeal complications, such as subglottic stenosis. The child should be able to breathe around the tube, as well as through it. If the anesthesiologist is unable to intubate the trachea, then a rigid bronchoscope should be used. If both of those maneuvers fail, then a tracheostomy or cricothyrotomy should be performed (see Fig. 12-25).251,252
Once the airway is secured, a culture of the pharynx and blood cultures are obtained, and aggressive medical therapy beginning with antibiotics should be commenced. It is recommended that cephalosporins (such as ceftriaxone 50 mg/kg/day)253–255 be used initially for antibiotic therapy, with the first dose immediately after the diagnosis is made and appropriate cultures taken.2 The duration of the treatment is controversial, but at least 3 to 5 days of IV antibiotics followed by oral therapy is usually the minimum. Steroids are not indicated. Supportive measures include IV hydration, airway care, sedation as necessary, and acetaminophen for fever. Negative-pressure pulmonary edema can develop after tracheal intubation in children with severe epiglottitis.195 When the child resumes swallowing and the fever abates, usually 24 to 48 hours after initiation of therapy, the acute supraglottic edema should be resolving and the child may be prepared for tracheal extubation.
Obstructive Laryngeal Papillomatosis
Recurrent respiratory papillomatosis, also known as juvenile laryngeal papillomatosis, is the most commonly found tumor in the larynx and upper airway in children. Recurrent respiratory papillomatosis is caused by the human papilloma virus (HPV). The incidence is only 1 in 400 births, even though active or latent viral infection is present in 10% to 25% of pregnant women.256 This disease is caused by HPV 6 and 11; the incidence may markedly decrease in the future, with the introduction of a maternal vaccine to prevent HPV infection for types 6, 11, 16, and 18.257–260 The papillomas are usually found in the larynx on the vocal cord margins, epiglottis, pharynx, or trachea (Fig. 31-17). If left untreated, symptoms of aphonia, respiratory distress, hoarseness, stridor, right ventricular hypertrophy, and cor pulmonale may occur.
The current treatment is primarily surgical removal of the papillomatous tissue using the CO2 laser under microscopic visualization. Alternatively, papillomas can be surgically debulked using an ultrasonic microdebrider or cup forceps before laser treatment. Topical application of mitomycin C (usually in combination with debulking procedures) has also been shown to be effective in suppressing these tumors. Nonsurgical treatment using interferon alfa-n1 has been beneficial in some children.261 The main goal of the treatments is to reduce the bulk of the lesion without scarring and permanent damage to the underlying mucosa.
The perioperative care can be very challenging and often depends on the degree of obstruction to airflow and the type and location of the papillomas.262 Pedunculated papillomas can produce complete ball-valve obstruction of the upper airway in certain positions. It is therefore prudent to avoid paralysis and to maintain spontaneous respirations until the airway is examined and the anesthesiologist is certain that assisted or controlled ventilation is possible. These children must be approached and monitored in the same manner as any child with anticipated severe airway obstruction (e.g., acute epiglottitis). The surgeon must be present in the OR when anesthesia is induced, with equipment immediately available to deal with complete airway obstruction, including rigid bronchoscopes and a tracheostomy–cricothyrotomy set. The problem of sharing the already compromised airway with the surgeon is worsened by the need to use a laser to excise these lesions. A laser (an acronym for light amplified by stimulated emission of radiation) consists of a tube with reflective mirrors at either end with an amplifying medium between them to generate electron activity in the form of light. The CO2 laser is the most widely used in medical practice, having particular application in the treatment of laryngeal or vocal cord papillomas, laryngeal webs, and resection of subglottic tissue and hemangiomas. A laser is useful for endoscopic procedures because the beam may be directed down open-tube endoscopes and is invisible, thereby affording the surgeon an unobstructed view of the lesion during resection. Laser energy is absorbed by tissue water, rapidly increasing its temperature, denaturing protein, and causing vaporization of the target tissue. The thermal energy produced by the laser beam cauterizes capillaries as it vaporizes tissues; therefore, bleeding is minimal and very little postoperative edema occurs.
These properties give the laser a high degree of specificity; however, they also provide the route by which a misdirected laser beam may cause injury to a child or to unprotected OR personnel.263 Laser radiation increases the temperature of absorbent material; therefore, flammable objects, such as surgical drapes, must be kept away from the path of the laser beam. Unprotected surfaces, such as skin, can be burned and must be shielded. Wet towels should be applied to cover the skin of the face and neck when the laser is being used to avoid burns from deflected beams.
The anesthetic management of these children depends on the approach the surgeon will use to remove the lesions. The basic choice is between intubation and nonintubation techniques. For the latter approach, the choice is between intermittent apnea versus jet ventilation.217 The ETT used during laser surgery can affect the safety of the technique. All standard polyvinylchloride ETTs are flammable and can be ignited and vaporized by a laser beam. Although red rubber ETTs do not vaporize, they deflect the laser beam when wrapped with metallic tape. The metallic tape can only be applied along the stem of the tube down to the cuff. As a result, the laser may damage the tube below the vocal cords. Alternatively, nonlatex ETTs are manufactured specifically for use during laser surgery. Some have a double cuff to protect the airway in the event the outer cuff is damaged by the laser beam. Others have a special matte finish that is effective in deflecting the laser beam along its entire length. Nonreflective flexible metal ETTs and specifically wrapped ETTs are also specifically manufactured for use during laser surgery (Fig. 31-18). The outer diameters of these special tubes are considerably larger than the polyvinylchloride counterpart, especially in the small sizes. Thus they may not be appropriate for use in very small infants or children with a severely narrowed airway. Table 31-10 presents a variety of such specialized tubes compared with standard ETTs. Although these ETTs offer some advantage, they are considerably more expensive than metallic-tape–wrapped red rubber ETTs. A syringe or a bag (500 or 1000 mL) of normal saline should be immediately available to douse ignited tissues in the event of an airway fire.
Although the goal is to achieve the desired depth of anesthesia to secure the airway with the child still spontaneously breathing, partial obstruction is frequently encountered before an adequate depth of anesthesia for laryngoscopy is achieved. In these cases, thrusting the jaw forward and applying positive pressure in the anesthetic circuit will maintain an open airway in most situations (see Chapter 4). If complete obstruction is encountered, then a single IV bolus of propofol (2 to 3 mg/kg) or a short-acting muscle relaxant may be necessary for immediate laryngoscopy and intubation, or to allow the surgeon to perform rigid bronchoscopy.
An apneic anesthetic technique without an ETT offers the best unobstructed view of the larynx and avoids the presence of flammable material (e.g., the ETT) in the path of the laser beam. The child is positioned for suspension laryngoscopy with eyes protected with moist eye pads, and the otomicroscope and CO2 laser equipment are aligned. The ETT is then removed and surgical resection is carried out during repeated periods of apnea. The need for reintubation is guided by the adequacy of oxygenation as reflected by the pulse oximeter. Reintubation can be readily performed by the surgeon by introducing the tracheal tube through the suspension laryngoscope under direct vision. After each reintubation, the lungs are ventilated manually to restore both the oxygen saturation and end-tidal CO2 to baseline. When those baselines are reached and surgery can resume, the trachea is extubated. This process is repeated until the surgery is complete.2
A modification of the apneic technique that avoids tracheal intubation is the jet ventilator. The operating laryngoscope may be fitted with a catheter through which O2 flows, entraining ambient air. In this manner, the lungs are intermittently inflated by the pressure delivered by the jet. The advantage of this technique is twofold. The surgical field is extremely quiet because large excursions of the diaphragm are eliminated and ventilation is uninterrupted. However, transtracheal jet ventilation carries a greater risk of pneumothorax in children than the transglottic approach.217 In the past, tension pneumothorax and pneumomediastinum occurred because of excessive peak inspiratory pressures during jetting. Maximum peak inspiratory jet pressures of approximately 15 mm Hg have reduced this risk dramatically. In morbidly obese children and those with severe disease of the small airways, effective ventilation may be difficult with this technique, and an alternate approach should be used.264 In addition, jet ventilation may theoretically distribute papilloma virus throughout the tracheobronchial tree, although this technique continues to be used.
Aspirated Foreign Bodies
Curious young children can push many loose objects into almost any orifice in their body. Objects inserted in the nose or ear are usually benign in nature and simple to remove once the child is anesthetized. Small button-sized battery foreign bodies require urgent removal because of their potential for extensive local damage.265 Impacted, or displaced objects present greater challenges for the anesthesiologist and endoscopist, and the danger of misplacement into the respiratory tract must always be considered.266
Tracheobronchial foreign-body aspiration is most common in toddlers 1 to 3 years of age. The majority (95%) of foreign bodies lodge in the right main-stem bronchus.267 A history of choking while eating or playing, persistent cough, or wheezing that does not respond to medical treatment may be the only manifestations. If the foreign body completely obstructs a bronchus, or creates a ball-valve phenomenon, distal hyperinflation from air trapping may occur; a hyperinflated lung during the expiratory phase may be the only indication of an aspirated foreign body on chest radiography (Fig. 31-19). The more distal the object is lodged in the airway, the more atelectatic changes are noted.
Foreign bodies lodge in the trachea (less than 5% of airway foreign bodies) if they are too large to pass the carina.268 The signs of a tracheal foreign body may include a brassy cough with or without abnormal voice, bidirectional stridor, or complete airway obstruction in the case of laryngeal foreign bodies. Any sharp object, or any object that causes acute UAO with cyanosis and an inability to maintain ventilation, requires emergent removal. Unroasted peanuts (with unsaturated double bonds in the oils) should be removed promptly, because the oil can cause an inflammatory response and subsequent pneumonitis (Fig. 31-20).269 In contrast, roasted peanuts (with saturated double bonds in the oils) may be present in the lungs for greater periods without inducing as severe an inflammatory response. In addition, peanuts tend to swell, fragment, and crumble over time, making removal “en bloc” extremely difficult. The child who presents with a history of cyanosis after aspiration of a nut, very likely has aspirated material into the trachea or into the lungs bilaterally (as in the case of a broken nut or multiple nuts) and should be assessed emergently. It should be noted that esophageal foreign bodies may compress the trachea as well (Fig. 31-21).
The anesthetic management of these children depends on the level, degree, and duration of obstruction. A child who aspirates a foreign body while eating, or soon thereafter, presents with the additional risk of a full stomach. Waiting for the stomach to empty may not be appropriate or even effective in the acute situation. IV metoclopramide (0.15 mg/kg) may be used to hasten stomach emptying but does not guarantee that the stomach empties.270 If time permits, the administration of an anticholinergic agent may be useful to reduce secretions. In the debate of how best to anesthetize a child with a full stomach and a compromised airway from an aspirated foreign body, concern for the airway takes precedence over the full stomach and an inhalational induction is recommended.
One of the major controversies in the anesthetic management of foreign body aspiration is whether to control ventilation or allow spontaneous respirations during bronchoscopy. Some endoscopists prefer a spontaneously breathing child, to prevent movement of the foreign body as it is being retrieved out of the airway. Sevoflurane is the preferred inhalational anesthetic in these children because it maintains spontaneous respirations, does not trigger upper airway reflex responses, and maintains hemodynamic stability.271 Anesthesia is usually maintained with 100% oxygen and sevoflurane, or a propofol-based TIVA technique.2 Halothane has some advantages over sevoflurane during maintenance because it is more soluble and more slowly eliminated, which allows more time to manipulate the airway without the child recovering from the anesthetic and reacting to the procedure. Alternatively, a propofol TIVA technique allows a steady level of anesthesia that is independent of ventilation and does not expose the OR personnel to waste anesthetic agents that inevitably egress from the airway around the bronchoscope. In some cases, a combined approach of sevoflurane in oxygen, as well as IV propofol, may be used. Often these children have very irritable airways because of the presence of the foreign body. The use of topical lidocaine (3 to 4 mg/kg) divided between the laryngeal structures and tracheal mucosa may be used to suppress airway reflexes and prevent coughing and bronchospasm.
Tracheostomy
Tracheostomy in infants and children is usually performed electively, as a planned procedure after an airway has already been established with an ETT. Indications for a planned tracheostomy include lesions, such as congenital or acquired vocal cord paralysis, central hypoventilation syndrome (Ondine curse), craniofacial abnormalities (e.g., Pierre Robin malformation), persistent laryngotracheomalacia, and congenital or acquired subglottic stenosis (see Fig. 36-3). Usually these children have had a period of watchful waiting, with the hope of avoiding tracheostomy. However, persistent hypoxemia, hypercarbia, or intermittent obstruction that cannot be eliminated with the natural airway, or failed extubation will force the need to secure the airway via a tracheostomy. Children from intensive care sometimes require tracheostomy for long-term ventilation or secretion management. Still other children have acute deteriorations of the airway and require tracheostomy on an emergent basis.
Once the trachea has been entered, a portion of the delivered tidal volume is lost through the incision, and ventilation may become inadequate. This is less of a problem if spontaneous respirations are maintained at this point. It is prudent to leave the ETT within the lumen of the trachea but withdrawn just proximal to the tracheal incision, so that it can be readily advanced should difficulty be encountered with passing the tracheotomy tube. Once the tracheotomy tube is in place and ventilation is confirmed, the ETT is removed, the sterile distal end of the clean anesthesia circuit is attached to the tracheotomy tube (and the proximal end to the anesthesia machine), and the wound is closed. In the event of the tracheostomy tube becoming dislodged or removed, the tracheal incision will close and attempts at reinsertion may cause bleeding, the creation of a false passage, or trauma to the tracheal wall. The tracheal lumen is identified by internal traction sutures, which are placed by the surgeon at the end of the surgical procedure (E-Fig. 31-6). With the surgeon pulling up on the external ends of these sutures, the tracheal incision is identified and the tracheotomy is opened so that an artificial airway can be inserted. The child should not leave the OR without the potentially lifesaving sutures in place and their laterality (right vs. left) properly identified. Flexible fiberoptic bronchoscopy through the new tracheostomy tube can be performed to confirm appropriate location of the tip of the tracheostomy tube above the carina.
Laryngotracheal Reconstruction
Glottic and subglottic stenosis, although rare, can be life threatening and difficult to manage, from both the surgeon’s and anesthesiologist’s points of view. Congenital laryngeal atresia and congenital laryngeal webs can be incompatible with life unless an emergent tracheostomy is performed at birth. When diagnosed antenatally, such an intervention can be undertaken before placental separation, described as an operation on placental support, or the ex-utero intrapartum treatment (EXIT) procedure (see Chapter 37). Treatment depends on the severity of laryngeal obstruction. In some instances, the defects are sufficiently severe to require immediate intubation or tracheostomy (see Fig. 36-2). Others may be discovered as an incidental finding while attempting to intubate the trachea for an unrelated surgical problem.272,201 Most membranous defects can be broken down by passing a bronchoscope through the lumen or incised using a surgical knife or scissors. Thin anterior webs can be managed by microendoscopic incision with a microsurgical knife or CO2 laser, staging the procedure for each side separately to avoid recurrence. The anesthetic management is similar to that for children undergoing laser excision of laryngeal papillomatosis.
Acquired subglottic stenosis is usually the result of prolonged tracheal intubation for respiratory support of infants born prematurely. In older children, it is often the result of laryngeal trauma. Symptoms usually relate to airway, voice, and feeding and, in the case of a laryngeal insult, often occur 2 to 4 weeks later. Progressive respiratory difficulty with biphasic stridor, dyspnea, air hunger, and retractions are typical. These children usually have a tendency toward prolonged courses of URIs. Soft tissue radiographs of the neck and computed axial tomography will locate the exact site and length of the stenotic segment. Because both gastroesophageal and gastrolaryngopharyngeal reflux disease are thought to contribute to the development and exacerbation of subglottic stenosis, these conditions must be excluded, usually by a 24-hour esophageal pH probe placement. However, direct endoscopic visualization of the larynx is ultimately required to fully evaluate the stenosis. Rigid and flexible endoscopy of the airway and esophagus is performed in the OR. Because of the small diameter of the airway, the rigid rod-lens telescope and/or a flexible bronchoscope are used to visualize the larynx and trachea beyond the obstruction. The trachea is then intubated, and the degree of air leak around the tube helps establish the degree of stenosis (see Fig. 31-13; see also Fig. 36-3, A).
The surgical management of these infants must be individualized according to the degree of obstruction and the general condition of the child.273 Most cases of moderate or severe subglottic stenosis require a tracheostomy at or below the third tracheal ring to establish a safe airway. The presence of a tracheostomy also helps to facilitate the airway management during subsequent procedures. For less severe cases, endoscopic dilation or CO2-laser endoscopic scar excision may be sufficient.
The more severe cases of laryngeal stenosis require external reconstruction. Of the many available options, an anterior cricoid split operation and laryngotracheal reconstructions are more frequently used. The cricoid split operation is performed with the use of general endotracheal anesthesia. The largest possible tracheal tube is inserted through the nose. An incision is made through the cricoid, and the cartilage springs open. The ETT will be readily visible in the lumen. Frequently, the incision will be extended to include the upper two tracheal rings and even the lower third of the thyroid cartilage (Fig. 31-22). Stay sutures are placed on each side of the incised cricoid, and the skin is loosely approximated. The ETT is left in place for about 7 days to act as a splint while the mucosal swelling subsides and the split cricoid heals. Endoscopy is not usually required, but corticosteroids are administered before extubation.
Single-stage laryngotracheal reconstruction is sometimes used in children without significant obstruction. A full-length nasotracheal tube is used to support the graft for 3 to 7 days, depending on the type of graft. The advantages of immediate decannulation, and possible avoidance of a tracheostomy altogether, make this approach appealing in appropriate candidates (Fig. 31-23).
The anesthetic challenges in these cases are many. The general condition of the child may not be perfect. Residual stigmata of prematurity are often present. The airway will be shared with the surgeon, and the tracheal tube that is placed in the stoma will need to be intermittently removed for surgical access and stent placement. A quiet surgical field is essential. The possibility of a pneumothorax during the cartilage graft harvesting should be kept in mind. The need to ensure that the stent, or the tracheal tube in case of a single-stage laryngotracheal reconstruction, is not dislodged in the ICU cannot be overstated. Accidental extubation cannot be allowed. A combination of sedation techniques and/or pharmacologic relaxation is necessary. The choice is often dictated by the individual policy in each ICU. Dexmedetomidine sedation may offer advantages as an alternative to propofol by providing a relatively rapid recovery from short-term sedation.274
Tracheocutaneous Fistula
Approximately 12% of children who have had their tracheostomy removed will retain a tracheocutaneous fistula.275 Surgical repair is generally required to remove the fistula track, followed by a primary closure of the defect.276–278 In some, this may be accomplished with simple endoscopic cauterization.279 Usually there is a preliminary endoscopic assessment of the stoma site, removal of residual granuloma, and then a determination of the best approach to closure.280 A multilayered closure is commonly used to close the fistula,281,282 whereas others recommend direct primary closure of the defect.283 The anesthesia approach generally includes maintenance of spontaneous respirations, and methods to avoid coughing or straining at the time of extubation. The main concern is the potential for the development of subcutaneous emphysema caused by residual air leak if the child coughs or strains.279,284–287 This complication may result in life-threatening pneumothorax or pneumomediastinum, which may occur up to 7 days following closure280; these complications require emergent removal of sutures, reestablishment of a tracheostomy, and thoracostomy and/or pericardial drainage.288 Some children may require postoperative ventilation, but most are extubated and admitted for observation after extubation and conclusion of the surgery. The vast majority have an uneventful recovery.
Airway Trauma
Nasal fractures are frequently seen in older children and adolescents. They may result from a direct hit (fight) or an accident.289 Because the nasal mucosa is very vascular, a lot of blood is usually swallowed. A stomach full of blood is to be assumed for the first 24 to 48 hours after the injury. A rapid-sequence intubation is the safest approach during that period. However, closed reduction of a nasal fracture is often delayed for a few days to allow swelling to subside. At that time, gastroparesis has resolved and an LMA may be considered. These operations can be very brief. Frequently, the surgeon will leave a nasal pack in situ and apply an external splint over the nose. If a throat pack was not inserted, then the stomach should be suctioned to remove blood that may have accumulated during the surgery. At the conclusion of the surgery, the stomach and pharynx should be suctioned. The tracheal tube or LMA should be removed only when the child is awake, cooperative, and understands the need for mouth breathing; a combative, semi-awake adolescent who is unable to breathe through the nose, can hurt himself or herself and others.
Closed or open injuries to the larynx and trachea in children can result from bicycle accidents, falls, direct trauma from sharp objects, and, rarely, a “clothesline” injury. The more cephalad cervical position of the pediatric larynx behind the mandibular arch and the pliability of the cricothyroid structures usually limit the extent of injury and prevent severe fractures.289 However, the small size of the laryngotracheal airway and the potential for massive soft tissue swelling because of the loose attachment of the submucosal tissue to the perichondrium make early diagnosis and treatment critical. The injury can range from minor laryngeal hematoma to a severe form of laryngotracheal separation. This extreme and often fatal condition can occur after a clothesline mechanism of injury and is often associated with bilateral vocal cord paralysis resulting from recurrent laryngeal nerve damage.290 Hoarseness, cough, dyspnea, hemoptysis, and voice changes suggest laryngeal damage. Clinical subcutaneous emphysema, pneumothorax, and pneumomediastinum signify definite disruption of the laryngotracheal complex. Computed tomography is the most appropriate imaging modality to identify the extent of laryngeal injury.291
Brown KA, Laferrière A, Lakheeram I, Moss IR. Recurrent hypoxemia in children is associated with increased analgesic sensitivity to opiates. Anesthesiology. 2006;105:665–669.
Gross JB, Bachenberg KL, Benumof JL, et al. Practice guidelines for the perioperative management of patients with obstructive sleep apnea: a report by the American Society of Anesthesiologists Task Force on Perioperative Management of patients with obstructive sleep apnea. Anesthesiology. 2006;104:1081–1093.
Marcus CL, Brooks LJ, Draper KA, et al. Diagnosis and management of childhood obstructive sleep apnea syndrome. Pediatrics. 2012;130:576–584.
Nixon GM, Kermack AS, Davis GM, et al. Planning adenotonsillectomy in children with obstructive sleep apnea: the role of overnight oximetry. Pediatrics. 2004;113:e19–e25.
Nixon GM, Kermack AS, McGregor CD, et al. Sleep and breathing on the first night after adenotonsillectomy for obstructive sleep apnea. Pediatr Pulmonol. 2005;39:332–338.
Schwengel DA, Sterni LM, Tunkel DE, Heitmilller ES. Perioperative management of children with obstructive sleep apnea. Anesth Analg. 2009;109:60–75.
Verghese ST, Hannallah RS. Otolaryngological emergencies. Anesthesiol Clin North Am. 2001;19:237–256.
A comprehensive review of pediatric airway emergencies is presented.
1 Shah RK, Welborn L, Ashktorab S, Stringer E, Zalzal GH. Safety and outcomes of outpatient pediatric otolaryngology procedures at an ambulatory surgery center. Laryngoscope. 2008;118:1937–1940.
2 Verghese ST, Hannallah RS. Pediatric otolaryngologic emergencies. Anesthesiol Clin North America. 2001;19:237–256.
3 Wilson G, Engelhardt T. Who needs an IV? Retrospective service analysis in a tertiary pediatric hospital. Paediatr Anaesth. 2012;22:442–444.
4 Korpela R, Korvenoja P, Meretoja OA. Morphine-sparing effect of acetaminophen in pediatric day-case surgery. Anesthesiology. 1999;91:442–447.
5 Birmingham PK, Tobin MJ, Fanta KB, et al. 24 hour pharmacokinetics of rectal acetaminophen in children: an old drug with new recommendations. Anesthesiology. 1995;83:A1127.
6 Birmingham PK, Tobin MJ, Fisher DM, et al. Initial and subsequent dosing of rectal acetaminophen in children: a 24-hour pharmacokinetic study of new dose recommendations. Anesthesiology. 2001;94:385–389.
7 Rampersad S, Jimenez N, Bradford H, Seidel K, Lynn A. Two-agent analgesia versus acetaminophen in children having bilateral myringotomies and tubes surgery. Paediatr Anaesth. 2010;20:1028–1035.
8 Anderson BJ, Woolard GA, Holford NH. Pharmacokinetics of rectal paracetamol after major surgery in children. Paediatr Anaesth. 1995;5:237–242.
9 Anderson BJ, Holford NH, Woollard GA, Kanagasundaram S, Mahadevan M. Perioperative pharmacodynamics of acetaminophen analgesia in children. Anesthesiology. 1999;90:411–421.
10 Anderson BJ, Rees SG, Liley A, Stewart AW, Wardill MJ. Effect of preoperative paracetamol on gastric volumes and pH in children. Paediatr Anaesth. 1999;9:203–207.
11 Anderson BJ, van Lingen RA, Hansen TG, Lin YC, Holford NH. Acetaminophen developmental pharmacokinetics in premature neonates and infants: a pooled population analysis. Anesthesiology. 2002;96:1336–1345.
12 Finkel JC, Cohen IT, Hannallah RS, et al. The effect of intranasal fentanyl on the emergence characteristics after sevoflurane anesthesia in children undergoing surgery for bilateral myringotomy tube placement. Anesth Analg. 2001;92:1164–1168.
13 Galinkin JL, Fazi LM, Cuy RM, et al. Use of intranasal fentanyl in children undergoing myringotomy and tube placement during halothane and sevoflurane anesthesia. Anesthesiology. 2000;93:1378–1383.
14 Davis PJ, Greenberg JA, Gendelman M, Fertal K. Recovery characteristics of sevoflurane and halothane in preschool-aged children undergoing bilateral myringotomy and pressure equalization tube insertion. Anesth Analg. 1999;88:34–38.
15 Bennie RE, Boehringer LA, Dierdorf SF, Hanna MP, Means LJ. Transnasal butorphanol is effective for postoperative pain relief in children undergoing myringotomy. Anesthesiology. 1998;89:385–390.
16 Pestieau SR, Quezado ZM, Johnson YJ, et al. The effect of dexmedetomidine during myringotomy and pressure-equalizing tube placement in children. Paediatr Anaesth. 2011;21:1128–1135.
17 Tait AR, Knight PR. The effects of general anesthesia on upper respiratory tract infections in children. Anesthesiology. 1987;67:930–935.
18 Tait AR, Pandit UA, Voepel-Lewis T, Munro HM, Malviya S. Use of the laryngeal mask airway in children with upper respiratory tract infections: a comparison with endotracheal intubation. Anesth Analg. 1998;86:706–711.
19 DeSoto H, Patel RI, Soliman IE, Hannallah RS. Changes in oxygen saturation following general anesthesia in children with upper respiratory infection signs and symptoms undergoing otolaryngological procedures. Anesthesiology. 1988;68:276–279.
20 Williams JP, Somerville GM, Miner ME, Reilly D. Atlanto-axial subluxation and trisomy-21: another perioperative complication. Anesthesiology. 1987;67:253–254.
21 Pueschel SM, Scola FH, Perry CD, Pezzullo JC. Atlanto-axial instability in children with Down syndrome. Pediatr Radiol. 1981;10:129–132.
22 Moore RA, McNicholas KW, Warran SP. Atlantoaxial subluxation with symptomatic spinal cord compression in a child with Down’s syndrome. Anesth Analg. 1987;66:89–90.
23 Kalla GN, Fening E, Obiaya MO. Anaesthetic management of achondroplasia. Br J Anaesth. 1986;58:117–119.
24 Casey WF, Drake-Lee AB. Nitrous oxide and middle ear pressure. A study of induction methods in children. Anaesthesia. 1982;37:896–900.
25 Patterson ME, Bartlett PC. Hearing impairment caused by intratympanic pressure changes during general anesthesia. Laryngoscope. 1976;86:399–404.
26 Munson ES. Transfer of nitrous oxide into body air cavities. Br J Anaesth. 1974;46:202–209.
27 Fisher DM, Zwass MS. MAC of desflurane in 60% nitrous oxide in infants and children. Anesthesiology. 1992;76:354–356.
28 Lerman J. Surgical and patient factors involved in postoperative nausea and vomiting. Br J Anaesth. 1992;69:24S–32S.
29 Suresh S, Barcelona SL, Young NM, Heffner CL, Cote CJ. Does a preemptive block of the great auricular nerve improve postoperative analgesia in children undergoing tympanomastoid surgery? Anesth Analg. 2004;98:330–333.
30 James AL, Papsin BC. Cochlear implant surgery at 12 months of age or younger. Laryngoscope. 2004;114:2191–2195.
31 Schultz A, Berger FA, Weber BP, et al. Intraoperative electrically elicited stapedius reflex threshold is related to the dosage of hypnotic drugs in general anesthesia. Ann Otol Rhinol Laryngol. 2003;112:1050–1055.
32 Clement PA, Bluestone CD, Gordts F, et al. Management of rhinosinusitis in children: consensus meeting, Brussels, Belgium, September 13, 1996. Arch Otolaryngol Head Neck Surg. 1998;124:31–34.
33 Groudine SB, Hollinger I, Jones J, DeBouno BA. New York State guidelines on the topical use of phenylephrine in the operating room. The Phenylephrine Advisory Committee. Anesthesiology. 2000;92:859–864.
34 Suresh S, Voronov P. Head and neck blocks in children: an anatomical and procedural review. Paediatr Anaesth. 2006;16:910–918.
35 Palmer GM. A teenager with severe asthma exacerbation following ibuprofen. Anaesth Intensive Care. 2005;33:261–265.
36 Grundfast KM, Wittich DJ, Jr. Adenotonsillar hypertrophy and upper airway obstruction in evolutionary perspective. Laryngoscope. 1982;92:650–656.
37 Carithers JS, Gebhart DE, Williams JA. Postoperative risks of pediatric tonsilloadenoidectomy. Laryngoscope. 1987;97:422–429.
38 Berkowitz RG, Zalzal GH. Tonsillectomy in children under 3 years of age. Arch Otolaryngol Head Neck Surg. 1990;116:685–686.
39 Potsic WP. Tonsillectomy and adenoidectomy. Int Anesthesiol Clin. 1988;26:58–60.
40 MacGregor FB, Albert DM, Bhattacharyya AK. Post-operative morbidity following paediatric tonsillectomy; a comparison of bipolar diathermy dissection and blunt dissection. Int J Pediatr Otorhinolaryngol. 1995;31:1–6.
41 Hanasono MM, Lalakea ML, Mikulec AA, et al. Perioperative steroids in tonsillectomy using electrocautery and sharp dissection techniques. Arch Otolaryngol Head Neck Surg. 2004;130:917–921.
42 Lalakea ML, Marquez-Biggs I, Messner AH. Safety of pediatric short-stay tonsillectomy. Arch Otolaryngol Head Neck Surg. 1999;125:749–752.
43 Blomgren K, Qvarnberg YH, Valtonen HJ. A prospective study on pros and cons of electrodissection tonsillectomy. Laryngoscope. 2001;111:478–482.
44 Leach J, Manning S, Schaefer S. Comparison of two methods of tonsillectomy. Laryngoscope. 1993;103:619–622.
45 Pratt LW, Gallagher RA. Tonsillectomy and adenoidectomy: incidence and mortality, 1968–1972. Otolaryngol Head Neck Surg. 1979;87:159–166.
46 Randall DA, Hoffer ME. Complications of tonsillectomy and adenoidectomy. Otolaryngol Head Neck Surg. 1998;118:61–68.
47 Flick RP, Sprung J, Harrison TE, et al. Perioperative cardiac arrests in children between 1988 and 2005 at a tertiary referral center: a study of 92,881 patients. Anesthesiology. 2007;106:226–237.
48 Newland MC, Ellis SJ, Lydiatt CA, et al. Anesthetic-related cardiac arrest and its mortality: a report covering 72,959 anesthetics over 10 years from a US teaching hospital. [see comment]. Anesthesiology. 2002;97:108–115.
49 Simonsen AR, Duncavage JA, Becker SS. A review of malpractice cases after tonsillectomy and adenoidectomy. Int J Pediatr Otorhinolaryngol. 2010;74:977–979.
50 Onerci M, Ogretmenoglu O, Ozcan OE. Atlantoaxial subluxation after tonsillectomy and adenoidectomy. Otolaryngol Head Neck Surg. 1997;116:271–273.
51 Schloss MD, Tan AK, Schloss B, Tewfik TL. Outpatient tonsillectomy and adenoidectomy: complications and recommendations. Int J Pediatr Otorhinolaryngol. 1994;30:115–122.
52 Richmond KH, Wetmore RF, Baranak CC. Postoperative complications following tonsillectomy and adenoidectomy—who is at risk? Int J Pediatr Otorhinolaryngol. 1987;13:117–124.
53 Kendrick D, Gibbin K. An audit of the complications of paediatric tonsillectomy, adenoidectomy and adenotonsillectomy. Clin Otolaryngol. 1993;18:115–117.
54 Wiatrak BJ, Myer CM, III., Andrews TM. Complications of adenotonsillectomy in children under 3 years of age. Am J Otolaryngol. 1991;12:170–172.
55 Mills N, Anderson BJ, Barber C, et al. Day stay pediatric tonsillectomy—a safe procedure. Int J Pediatr Otorhinolaryngol. 2004;68:1367–1373.
56 Tom LW, DeDio RM, Cohen DE, et al. Is outpatient tonsillectomy appropriate for young children? Laryngoscope. 1992;102:277–280.
57 Berkowitz RG. Tonsillectomy in children under 3 years of age. Arch Otolaryngol Head Neck Surg. 1990;116:685–686.
58 Cohen MM, Cameron CB, Duncan PG. Pediatric anesthesia morbidity and mortality in the perioperative period. Anesth Analg. 1990;70:160–167.
59 Cohen MM, Cameron CB. Should you cancel the operation when a child has an upper respiratory tract infection? Anesth Analg. 1991;72:282–288.
60 Brodsky L. Modern assessment of tonsils and adenoids. Pediatr Clin North Am. 1989;36:1551–1569.
61 Zwack GC, Derkay CS. The utility of preoperative hemostatic assessment in adenotonsillectomy. Int J Pediatr Otorhinolaryngol. 1997;39:67–76.
62 Howells RC, Wax MK, Ramadan HH. Value of preoperative prothrombin time/partial thromboplastin time as a predictor of postoperative hemorrhage in pediatric patients undergoing tonsillectomy. Otolaryngol Head Neck Surg. 1997;117:628–632.
63 Barone JG, Hanson C, DaJusta DG, et al. Nocturnal enuresis and overweight are associated with obstructive sleep apnea. Pediatrics. 2009;124:e53.
64 Basha S, Bialowas C, Ende K, Szeremeta W. Effectiveness of adenotonsillectomy in the resolution of nocturnal enuresis secondary to obstructive sleep apnea. Laryngoscope. 2005;115:1101–1103.
65 MS Su, AM Li, HK So, et al. Nocturnal enuresis in children: prevalence, correlates, and relationship with obstructive sleep apnea. J Pediatr. 2011;159:238–242.
66 Umlauf MG, Chasens ER. Bedwetting—not always what it seems: a sign of sleep-disordered breathing in children. J Spec Pediatr Nurs. 2003;8:22–30.
67 Xu Z, Cheuk DK, Lee SL. Clinical evaluation in predicting childhood obstructive sleep apnea. Chest. 2006;130:1765–1771.
68 Rosen GM, Muckle RP, Mahowald MW, Goding GS, Ullevig C. Postoperative respiratory compromise in children with obstructive sleep apnea syndrome: can it be anticipated? Pediatrics. 1994;93:784–788.
69 McColley SA, April MM, Carroll JL, Naclerio RM, Loughlin GM. Respiratory compromise after adenotonsillectomy in children with obstructive sleep apnea. Arch Otolaryngol Head Neck Surg. 1992;118:940–943.
70 Brown KA, Morin I, Hickey C, et al. Urgent adenotonsillectomy: an analysis of risk factors associated with postoperative respiratory morbidity. Anesthesiology. 2003;99:586–595.
71 Wilson K, Lakheeram I, Morielli A, Brouillette R, Brown K. Can assessment for obstructive sleep apnea help predict postadenotonsillectomy respiratory complications? Anesthesiology. 2002;96:313–322.
71a Marcus CL, Brooks LJ, Draper KA, et al. Diagnosis and management of childhood obstructive sleep apnea syndrome. Pediatrics. 2012;130:576–584.
71b Goldman JL, Baugh RF, Davie L, et al. Mortality and major morbidity after tonsillectomy: etiologic factors and strategies for prevention. Laryngoscope. 2012. In press
72 Carroll JL. Obstructive sleep-disordered breathing in children: new controversies, new directions. Clin Chest Med. 2003;24:261–282.
73 Schwengel DA, Sterni LM, Tunkel DE, Heitmiller ES. Perioperative management of children with obstructive sleep apnea. Anesth Analg. 2009;109:60–75.
74 Raghavendran S, Bagry H, Detheux G, et al. An anesthetic management protocol to decrease respiratory complications after adenotonsillectomy in children with severe sleep apnea. Anesth Analg. 2010;110:1093–1101.
75 Carroll JL, McColley SA, Marcus CL, Curtis S, Loughlin GM. Inability of clinical history to distinguish primary snoring from obstructive sleep apnea syndrome in children. Chest. 1995;108:610–618.
76 Redline S, Tishler PV, Hans MG, et al. Racial differences in sleep-disordered breathing in African-Americans and Caucasians. Am J Respir Crit Care Med. 1997;155:186–192.
77 Palmer LJ, Buxbaum SG, Larkin EK, et al. Whole genome scan for obstructive sleep apnea and obesity in African-American families. Am J Respir Crit Care Med. 2004;169:1314–1321.
78 Stepanski E, Zayyad A, Nigro C, Lopata M, Basner R. Sleep-disordered breathing in a predominantly African-American pediatric population. J Sleep Res. 1999;8:65–70.
79 McNamara F, Sullivan CE. Obstructive sleep apnea in infants: relation to family history of sudden infant death syndrome, apparent life-threatening events, and obstructive sleep apnea. J Pediatr. 2000;136:318–323.
80 Guilleminault C, Stoohs R. From apnea of infancy to obstructive sleep apnea syndrome in the young child. Chest. 1992;102:1065–1071.
81 Guilleminault C, Robinson A. Developmental aspects of sleep and breathing. Curr Opin Pulm Med. 1996;2:492–499.
82 Tal A, Leiberman A, Margulis G, Sofer S. Ventricular dysfunction in children with obstructive sleep apnea: radionuclide assessment. Pediatr Pulmonol. 1988;4:139–143.
83 Amin RS, Kimball TR, Bean JA, et al. Left ventricular hypertrophy and abnormal ventricular geometry in children and adolescents with obstructive sleep apnea. Am J Respir Crit Care Med. 2002;165:1395–1399.
84 Dyson M, Beckerman RC, Brouillette RT. Obstructive sleep apnea syndrome. In: Beckerman RC, Brouillette RT, Hunt CE, eds. Respiratory control disorders in infants and children. Baltimore: Williams & Wilkins; 1992:212–230.
85 Guilleminault C, Pelayo R, Leger D, Clerk A, Bocian RC. Recognition of sleep-disordered breathing in children. Pediatrics. 1996;98:871–882.
86 Konno A, Hoshino T, Togawa K. Influence of upper airway obstruction by enlarged tonsils and adenoids upon recurrent infection of the lower airway in childhood. Laryngoscope. 1980;90:1709–1716.
87 Ward SL, Marcus CL. Obstructive sleep apnea in infants and young children. J Clin Neurophysiol. 1996;13:198–207.
88 Goh DY, Galster P, Marcus CL. Sleep architecture and respiratory disturbances in children with obstructive sleep apnea. Am J Respir Crit Care Med. 2000;162:682–686.
89 McGuire M, MacDermott M, Bradford A. The effects of chronic episodic hypercapnic hypoxia on rat upper airway muscle contractile properties and fiber-type distribution. Chest. 2002;122:1400–1406.
90 Marcus CL, Omlin KJ, Basinki DJ, et al. Normal polysomnographic values for children and adolescents. Am Rev Respir Dis. 1992;146:1235–1239.
91 Clinical practice guideline: diagnosis and management of childhood obstructive sleep apnea syndrome. Pediatrics. 2002;109:704–712.
92 Sanders JC, King MA, Mitchell RB, Kelly JP. Perioperative complications of adenotonsillectomy in children with obstructive sleep apnea syndrome. Anesth Analg. 2006;103:1115–1121.
93 Suen JS, Arnold JE, Brooks LJ. Adenotonsillectomy for treatment of obstructive sleep apnea in children. Arch Otolaryngol Head Neck Surg. 1995;121:525–530.
94 Weinberg S, Kravath R, Phillips L, Mendez H, Wolf GL. Episodic complete airway obstruction in children with undiagnosed obstructive sleep apnea. Anesthesiology. 1984;60:356–358.
95 Price SD, Hawkins DB, Kahlstrom EJ. Tonsil and adenoid surgery for airway obstruction: perioperative respiratory morbidity. Ear Nose Throat J. 1993;72:526–531.
96 Francis A, Eltaki K, Bash T, et al. The safety of preoperative sedation in children with sleep-disordered breathing. Int J Pediatr Otorhinolaryngol. 2006;70:1517–1521.
97 Welborn LG, Hannallah RS, Norden JM, Ruttimann UE, Callan CM. Comparison of emergence and recovery characteristics of sevoflurane, desflurane, and halothane in pediatric ambulatory patients. Anesth Analg. 1996;83:917–920.
98 Mayer J, Boldt J, Rohm KD, Scheuermann K, Suttner SW. Desflurane anesthesia after sevoflurane inhaled induction reduces severity of emergence agitation in children undergoing minor ear-nose-throat surgery compared with sevoflurane induction and maintenance. Anesth Analg. 2006;102:400–404.
99 Cox RG. Should cuffed endotracheal tubes be used routinely in children? Can J Anaesth. 2005;52:669–674.
100 Jones JE, Tabaee A, Glasgold R, Gomillion MC. Efficacy of gastric aspiration in reducing posttonsillectomy vomiting. Arch Otolaryngol Head Neck Surg. 2001;127:980–984.
101 Tsui BC, Wagner A, Cave D, et al. The incidence of laryngospasm with a “no touch” extubation technique after tonsillectomy and adenoidectomy. Anesth Analg. 2004;98:327–329.
102 Alexander CA. A modified Intavent laryngeal mask for ENT and dental anaesthesia. Anaesthesia. 1990;45:892–893.
103 Haynes SR, Morton NS. The laryngeal mask airway: a review of its use in paediatric anaesthesia. Paediatr Anaesth. 1993;3:65–73.
104 Nair I, Bailey PM. Review of uses of the laryngeal mask in ENT anaesthesia. Anaesthesia. 1995;50:898–900.
105 Williams PJ, Bailey PM. Comparison of the reinforced laryngeal mask airway and tracheal intubation for adenotonsillectomy. Br J Anaesth. 1993;70:30–33.
106 Webster AC, Morley-Forster PK, Dain S, et al. Anaesthesia for adenotonsillectomy: a comparison between tracheal intubation and the armoured laryngeal mask airway. Can J Anaesth. 1993;40:1171–1177.
107 Peng A, Dodson KM, Thacker LR, et al. Use of laryngeal mask airway in pediatric adenotonsillectomy. Arch Otolaryngol Head Neck Surg. 2011;137:42–46.
108 Mason DG, Bingham RM. The laryngeal mask airway in children. Anaesthesia. 1990;45:760–763.
109 Nunez DA, Provan J, Crawford M. Postoperative tonsillectomy pain in pediatric patients: electrocautery (hot) vs cold dissection and snare tonsillectomy—a randomized trial. Arch Otolaryngol Head Neck Surg. 2000;126:837–841.
110 Leaper M, Mahadevan M, Vokes D, et al. A prospective randomised single blinded study comparing harmonic scalpel tonsillectomy with bipolar tonsillectomy. Int J Pediatr Otorhinolaryngol. 2006;70:1389–1396.
111 Anderson BJ, Ralph CJ, Stewart AW, Barber C, Holford NH. The dose-effect relationship for morphine and vomiting after day-stay tonsillectomy in children. Anaesth Intensive Care. 2000;28:155–160.
112 Elhakim M, Ali NM, Rashed I, Riad MK, Refat M. Dexamethasone reduces postoperative vomiting and pain after pediatric tonsillectomy. Can J Anaesth. 2003;50:392–397.
113 Kim MS, Coté CJ, Cristoloveanu C, et al. There is no dose-escalation response to dexamethasone (0.0625-1.0 mg/kg) in pediatric tonsillectomy or adenotonsillectomy patients for preventing vomiting, reducing pain, shortening time to first liquid intake, or the incidence of voice change. Anesth Analg. 2007;104:1052–1058.
114 Celiker V, Celebi N, Canbay O, Basgul E, Aypar U. Minimum effective dose of dexamethasone after tonsillectomy. Paediatric Anaesthesia. 2004;14:666–669.
115 Corcoran TB, Truyens EB, Ng A, et al. Anti-emetic dexamethasone and postoperative infection risk: a retrospective cohort study. Anaesth Intensive Care. 2010;38:654–660.
116 Chanimov M, Koren-Michowitz M, Cohen ML, Pilipodi S, Bahar M. Tumor lysis syndrome induced by dexamethasone. Anesthesiology. 2006;105:633–634.
117 McDonnell C, Barlow R, Campisi P, Grant R, Malkin D. Fatal peri-operative acute tumour lysis syndrome precipitated by dexamethasone. Anaesthesia. 2008;63:652–655.
118 Czarnetzki C, Elia N, Lysakowski C, et al. Dexamethasone and risk of nausea and vomiting and postoperative bleeding after tonsillectomy in children: a randomized trial. JAMA. 2008;300:2621–2630.
119 Windfuhr JP, Chen YS, Propst EJ, Guldner C. The effect of dexamethasone on post-tonsillectomy nausea, vomiting and bleeding. Braz J Otorhinolaryngol. 2011;77:373–379.
120 Shakeel M, Trinidade A, Al-Adhami A, et al. Intraoperative dexamethasone and the risk of secondary posttonsillectomy hemorrhage. J Otolaryngol Head Neck Surgery. 2010;39:732–736.
121 Brigger MT, Cunningham MJ, Hartnick CJ. Dexamethasone administration and postoperative bleeding risk in children undergoing tonsillectomy. Arch Otolaryngol Head Neck Surg. 2010;136:766–772.
122 Geva A, Brigger MT. Dexamethasone and tonsillectomy bleeding: a meta-analysis. Otolaryngol Head Neck Surg. 2011;144:838–843.
122a Plante J, Turgeon AF, Zarychanski R, et al. Effect of systemic steroids on post-tonsillectomy bleeding and reinterventions: systematic review and meta-analysis of randomised controlled trials. BMJ. 2012;345:e5389.
123 Marret E, Flahault A, Samama CM, Bonnet F. Effects of postoperative, nonsteroidal, antiinflammatory drugs on bleeding risk after tonsillectomy: meta-analysis of randomized, controlled trials. Anesthesiology. 2003;98:1497–1502.
124 Cardwell M, Siviter G, Smith A. Non-steroidal anti-inflammatory drugs and perioperative bleeding in paediatric tonsillectomy. Cochrane Database Syst Rev. 2005. CD003591
125 Dsida RM, Wheeler M, Birmingham PK, et al. Age-stratified pharmacokinetics of ketorolac tromethamine in pediatric surgical patients. Anesth Analg. 2002;94:266–270.
126 Agrawal A, Gerson CR, Seligman I, Dsida RM. Postoperative hemorrhage after tonsillectomy: use of ketorolac tromethamine. Otolaryngol Head Neck Surg. 1999;120:335–339.
127 Anderson BJ, Woollard GA, Holford NH. Acetaminophen analgesia in children: placebo effect and pain resolution after tonsillectomy. Eur J Clin Pharmacol. 2001;57:559–569.
128 Capici F, Ingelmo PM, Davidson A, et al. Randomized controlled trial of duration of analgesia following intravenous or rectal acetaminophen after adenotonsillectomy in children. Br J Anaesth. 2008;100:251–255.
129 Beringer RM, Thompson JP, Parry S, Stoddart PA. Intravenous paracetamol overdose: two case reports and a change to national treatment guidelines. Arch Dis Child. 2011;96:307–308.
130 Patel A, Davidson M, Tran MC, et al. Dexmedetomidine infusion for analgesia and prevention of emergence agitation in children with obstructive sleep apnea syndrome undergoing tonsillectomy and adenoidectomy. Anesth Analg. 2010;111:1004–1010.
131 Pestieau SR, Quezado ZM, Johnson YJ, et al. High-dose dexmedetomidine increases the opioid-free interval and decreases opioid requirement after tonsillectomy in children. Can J Anaesth. 2011;58:540–550.
132 Wong AK, Bissonnette B, Braude BM, et al. Post-tonsillectomy infiltration with bupivacaine reduces immediate postoperative pain in children. Can J Anaesth. 1995;42:770–774.
133 Bean-Lijewski JD. Glossopharyngeal nerve block for pain relief after pediatric tonsillectomy: retrospective analysis and two cases of life-threatening upper airway obstruction from an interrupted trial. Anesth Analg. 1997;84:1232–1238.
134 Ohlms LA. Injection of local anesthetic in tonsillectomy. Arch Otolaryngol Head Neck Surg. 2001;127:1276–1278.
135 Mather SJ, Peutrell JM. Postoperative morphine requirements, nausea and vomiting following anaesthesia for tonsillectomy. Comparison of intravenous morphine and non-opioid analgesic techniques. Paediatr Anaesth. 1995;5:185–188.
136 Anderson BJ, Pearce S, McGann JE, Newson AJ, Holford NH. Investigations using logistic regression models on the effect of the LMA on morphine induced vomiting after tonsillectomy. Paediatr Anaesth. 2000;10:633–638.
136a Ved SA, Walden TL, Montana I, et al. Vomiting and recovery after outpatient tonsillectomy and adenoidectomy in children. Anesthesiology. 1996;85:4–10.
136b Erdem AF, Yoruk O, Alici HA, et al. Subhypnotic propofol infusion plus dexamethasone is more effective than dexamethasone alone for the prevention of vomiting in children after tonsillectomy. Pediatr Anesth. 2008;18:878–883.
137 Davis PJ, Fertal KM, Boretsky KR, et al. The effects of oral ondansetron disintegrating tablets for prevention of at-home emesis in pediatric patients after ear-nose-throat surgery. Anesth Analg. 2008;106:1117–1121.
138 Steward DL, Welge JA, Myer CM. Steroids for improving recovery following tonsillectomy in children. Cochrane Database Syst Rev. 2003. Issue 1, CD003997
139 Splinter WM, Roberts DJ. Dexamethasone decreases vomiting by children after tonsillectomy. Anesth Analg. 1996;83:913–916.
140 Liodden I, Howley M, Grimsgaard AS, et al. Perioperative acupuncture and postoperative acupressure can prevent postoperative vomiting following paediatric tonsillectomy or adenoidectomy: a pragmatic randomised controlled trial. Acupunct Med. 2011;29:9–15.
141 Norheim AJ, Liodden I, Howley M. Implementation of acupuncture and acupressure under surgical procedures in children: a pilot study. Acupunct Med. 2010;28:71–73.
142 Fortier MA, Weinberg M, Vitulano LA, et al. Effects of therapeutic suggestion in children undergoing general anesthesia: a randomized controlled trial. Paediatric Anaesthesia. 2010;20:90–99.
143 Ochiai R, Guthrie RD, Motoyama E. Effects of varying concentrations of halothane on the activity of the genioglossus, intercostals, and diaphragm in cats: An electromyographic study. Anesthesiology. 1989;70:812–816.
144 Drummond GB. Comparison of sedation with midazolam and ketamine: effects on airway muscle activity. Br J Anaesth. 1996;76:663–667.
145 Crawford MW, Arrica M, Macgowan CK, Yoo SJ. Extent and localization of changes in upper airway caliber with varying concentrations of sevoflurane in children. Anesthesiology. 2006;105:1147–1152.
146 Evans RG, Crawford MW, Noseworthy MD, Yoo SJ. Effect of increasing depth of propofol anesthesia on upper airway configuration in children. Anesthesiology. 2003;99:596–602.
147 Arens R, McDonough JM, Corbin AM, et al. Upper airway size analysis by magnetic resonance imaging of children with obstructive sleep apnea syndrome. Am J Respir Crit Care Med. 2003;167:65–70.
148 Crawford MW, Rohan D, Macgowan CK, Yoo SJ, Macpherson BA. Effect of propofol anesthesia and continuous positive airway pressure on upper airway size and configuration in infants. Anesthesiology. 2006;105:45–50.
149 Issa FG, Sullivan CE. Upper airway closing pressures in obstructive sleep apnea. J Appl Physiol. 1984;57:520–527.
150 Schwab RJ, Pack AI, Gupta KB, et al. Upper airway and soft tissue structural changes induced by CPAP in normal subjects. Am J Respir Crit Care Med. 1996;154:1106–1116.
151 Hoffstein V, Zamel N, Phillipson EA. Lung volume dependence of pharyngeal cross-sectional area in patients with obstructive sleep apnea. Am Rev Respir Dis. 1984;130:175–178.
152 Isono S, Tanaka A, Nishino T. Dynamic interaction between the tongue and soft palate during obstructive apnea in anesthetized patients with sleep-disordered breathing. J Appl Physiol. 2003;95:2257–2264.
153 Isono S, Remmers JE, Tanaka A, Sho Y, Nishino T. Static properties of the passive pharynx in sleep apnea. Sleep. 1996;19:S175–S177.
154 Laferriere A, Liu JK, Moss IR. Neurokinin-1 versus mu-opioid receptor binding in rat nucleus tractus solitarius after single and recurrent intermittent hypoxia. Brain Res Bull. 2003;59:307–313.
155 Lalley PM. Mu-opioid receptor agonist effects on medullary respiratory neurons in the cat: evidence for involvement in certain types of ventilatory disturbances. Am J Physiol Regul Integr Comp Physiol. 2003;285:R1287–R1304.
156 Moss IR, Laferriere A. Central neuropeptide systems and respiratory control during development. Respir Physiol Neurobiol. 2002;131:15–27.
157 Moss IR. Maturation of respiratory control in the behaving mammal. Respir Physiol Neurobiol. 2002;132:131–144.
158 Brown KA, Laferriere A, Moss IR. Recurrent hypoxemia in young children with obstructive sleep apnea is associated with reduced opioid requirement for analgesia. Anesthesiology. 2004;100:806–810.
159 Brown KA, Laferriere A, Lakheeram I, Moss IR. Recurrent hypoxemia in children is associated with increased analgesic sensitivity to opiates. Anesthesiology. 2006;105:665–669.
160 Lerman J. Unraveling the mysteries of sleep-disordered breathing in children. Anesthesiology. 2006;105:645–647.
161 Waters KA, McBrien F, Stewart P, Hinder M, Wharton S. Effects of OSA, inhalational anesthesia, and fentanyl on the airway and ventilation of children. J Appl Physiol. 2002;92:1987–1994.
162 Moss IR, Brown KA, Laferriere A. Recurrent hypoxia in rats during development increases subsequent respiratory sensitivity to fentanyl. Anesthesiology. 2006;105:715–718.
163 Brooks D, Horner RL, Kimoff RJ, et al. Effect of obstructive sleep apnea versus sleep fragmentation on responses to airway occlusion. Am J Respir Crit Care Med. 1997;155:1609–1617.
164 Gozal E, Row BW, Schurr A, Gozal D. Developmental differences in cortical and hippocampal vulnerability to intermittent hypoxia in the rat. Neurosci Lett. 2001;305:197–201.
165 Waters KA, Tinworth KD. Depression of ventilatory responses after daily, cyclic hypercapnic hypoxia in piglets. J Appl Physiol. 2001;90:1065–1073.
166 Durand E, Lofaso F, Dauger S, et al. Intermittent hypoxia induces transient arousal delay in newborn mice. J Appl Physiol. 2004;96:1216–1222.
167 Waters KA, Tinworth KD. Habituation of arousal responses after intermittent hypercapnic hypoxia in piglets. Am J Respir Crit Care Med. 2005;171:1305–1311.
168 Osman NI, Baghdoyan HA, Lydic R. Morphine inhibits acetylcholine release in rat prefrontal cortex when delivered systemically or by microdialysis to basal forebrain. Anesthesiology. 2005;103:779–787.
169 Kirchheiner J, Schmidt H, Tzvetkov M, et al. Pharmacokinetics of codeine and its metabolite morphine in ultra-rapid metabolizers due to CYP2D6 duplication. Pharmacogenomics J. 2007;7:257–265.
170 Gasche Y, Daali Y, Fathi M, et al. Codeine intoxication associated with ultrarapid CYP2D6 metabolism. N Engl J Med. 2004;351:2827–2831.
171 Ciszkowski C, Madadi P, Phillips MS, Lauwers AE, Koren G. Codeine, ultrarapid-metabolism genotype, and postoperative death. N Engl J Med. 2009;361:827–828.
171a Kelly LE, Rieder M, van den Anker J, et al. More codeine fatalities after tonsillectomy in North American children. Pediatrics. 2012;129:e1343–e1347.
172 Schwab RJ, Gupta KB, Gefter WB, et al. Upper airway and soft tissue anatomy in normal subjects and patients with sleep-disordered breathing. Significance of the lateral pharyngeal walls. Am J Respir Crit Care Med. 1995;152:1673–1689.
173 Isono S, Remmers JE, Tanaka A, et al. Anatomy of pharynx in patients with obstructive sleep apnea and in normal subjects. J Appl Physiol. 1997;82:1319–1326.
174 Isono S, Shimada A, Utsugi M, Konno A, Nishino T. Comparison of static mechanical properties of the passive pharynx between normal children and children with sleep-disordered breathing. Am J Respir Crit Care Med. 1998;157:1204–1212.
175 Eastwood PR, Platt PR, Shepherd K, Maddison K, Hillman DR. Collapsibility of the upper airway at different concentrations of propofol anesthesia. Anesthesiology. 2005;103:470–477.
176 Gozal D, Burnside MM. Increased upper airway collapsibility in children with obstructive sleep apnea during wakefulness. Am J Respir Crit Care Med. 2004;169:163–167.
177 Isono S, Kochi T, Ide T, et al. Differential effects of vecuronium on diaphragm and geniohyoid muscle in anaesthetized dogs. Br J Anaesth. 1992;68:239–243.
178 Chhibber AK, Lustik SJ, Thakur R, Francisco DR, Fickling KB. Effects of anticholinergics on postoperative vomiting, recovery, and hospital stay in children undergoing tonsillectomy with or without adenoidectomy. Anesthesiology. 1999;90:697–700.
179 Koomson A, Morin I, Brouillette R, Brown KA. Children with severe OSAS who have adenotonsillectomy in the morning are less likely to have postoperative desaturation than those operated in the afternoon: [Les enfants atteints d’un SAOS severe, operes pour amygdalectomie le matin, sont moins susceptibles de desaturation postoperatoire que les operes d’apres-midi]. Can J Anaesth. 2004;51:62–67.
180 Horner RL, Liu X, Gill H, et al. Effects of sleep-wake state on the genioglossus vs. diaphragm muscle response to CO(2) in rats. J Appl Physiol. 2002;92:878–887.
181 Liu X, Sood S, Liu H, Horner RL. Opposing muscarinic and nicotinic modulation of hypoglossal motor output to genioglossus muscle in rats in vivo. J Physiol. 2005;565:965–980.
182 Gleadhill IC, Schwartz AR, Schubert N, et al. Upper airway collapsibility in snorers and in patients with obstructive hypopnea and apnea. Am Rev Respir Dis. 1991;143:1300–1303.
183 Thut DC, Schwartz AR, Roach D, et al. Tracheal and neck position influence upper airway airflow dynamics by altering airway length. J Appl Physiol. 1993;75:2084–2090.
184 Litman RS, Wake N, Chan LM, et al. Effect of lateral positioning on upper airway size and morphology in sedated children. Anesthesiology. 2005;103:484–488.
185 Arai YC, Fukunaga K, Hirota S, Fujimoto S. The effects of chin lift and jaw thrust while in the lateral position on stridor score in anesthetized children with adenotonsillar hypertrophy. Anesth Analg. 2004;99:1638–1641.
186 Isono S, Tanaka A, Nishino T. Lateral position decreases collapsibility of the passive pharynx in patients with obstructive sleep apnea. Anesthesiology. 2002;97:780–785.
187 Bouryi VA, Lewis DI. Enkephalinergic inhibition of raphe pallidus inputs to rat hypoglossal motoneurones in vitro. Neuroscience. 2004;129:55–64.
188 Hajiha M, DuBord MA, Liu H, Horner RL. Opioid receptor mechanisms at the hypoglossal motor pool and effects on tongue muscle activity in vivo 1. J Physiol. 2009;587:2677–2692.
189 Skulsky EM, Osman NI, Baghdoyan HA, Lydic R. Microdialysis delivery of morphine to the hypoglossal nucleus of Wistar rat increases hypoglossal acetylcholine release. Sleep. 2007;30:566–573.
190 Bhattacharjee R, Kheirandish-Gozal L, Spruyt K, et al. Adenotonsillectomy outcomes in treatment of obstructive sleep apnea in children: a multicenter retrospective study. Am J Respir Crit Care Med. 2010;182:676–683.
191 Nixon GM, Kermack AS, McGregor CD, et al. Sleep and breathing on the first night after adenotonsillectomy for obstructive sleep apnea. Pediatr Pulmonol. 2005;39:332–338.
192 Helfaer MA, McColley SA, Pyzik PL, et al. Polysomnography after adenotonsillectomy in mild pediatric obstructive sleep apnea. Crit Care Med. 1996;24:1323–1327.
193 Mitchell RB. Adenotonsillectomy for obstructive sleep apnea in children: outcome evaluated by pre- and postoperative polysomnography. Laryngoscope. 2007;117:1844–1854.
194 Friedman O, Chidekel A, Lawless ST, Cook SP. Postoperative bilevel positive airway pressure ventilation after tonsillectomy and adenoidectomy in children–a preliminary report. Int J Pediatr Otorhinolaryngol. 1999;51:177–180.
195 Thiagarajan RR, Laussen PC. Negative pressure pulmonary edema in children—pathogenesis and clinical management. Paediatr Anaesth. 2007;17:307–310.
196 Chowdary YC, Patel JP. Recurrent pulmonary edema: an uncommon presenting feature of childhood obstructive sleep apnea hypoventilation syndrome in an otherwise healthy child. Clin Pediatr (Phila). 2001;40:287–290.
197 Feinberg AN, Shabino CL. Acute pulmonary edema complicating tonsillectomy and adenoidectomy. Pediatrics. 1985;75:112–114.
198 Brown OE, Cunningham MJ. Tonsillectomy and adenoidectomy inpatient guidelines: recommendations of the AAO-HNS Pediatric Otolaryngology Committee. Am Acad Otolaryngol-Head Neck Surg Bulletin. 1996;15:13–15.
199 Weatherly RA, Mai EF, Ruzicka DL, Chervin RD. Identification and evaluation of obstructive sleep apnea prior to adenotonsillectomy in children: a survey of practice patterns. Sleep Med. 2003;4:297–307.
200 Rothschild MA, Catalano P, Biller HF. Ambulatory pediatric tonsillectomy and the identification of high-risk subgroups. Otolaryngol Head Neck Surg. 1994;110:203–210.
201 Brietzke SE, Katz ES, Roberson DW. Can history and physical examination reliably diagnose pediatric obstructive sleep apnea/hypopnea syndrome? A systematic review of the literature. Otolaryngol Head Neck Surg. 2004;131:827–832.
202 Croft CB, Brockbank MJ, Wright A, Swanston AR. Obstructive sleep apnoea in children undergoing routine tonsillectomy and adenoidectomy. Clin Otolaryngol Allied Sci. 1990;15:307–314.
203 McGoldrick KE. Outpatient tonsilloadenoidectomy: avoiding complications. Ambul Surg. 1994;2:23–26.
204 Crysdale WS, Russel D. Complications of tonsillectomy and adenoidectomy in 9409 children observed overnight. CMAJ. 1986;135:1139–1142.
205 Fields RG, Gencorelli FJ, Litman RS. Anesthetic management of the pediatric bleeding tonsil. Paediatr Anaesth. 2010;20:982–986.
206 Berry F. Post-tonsillectomy bleeding. In: Stehling L, ed. Common problems in pediatric anesthesia. St Louis: Mosby-Year Book, 1992.
207 Sellick BA. Cricoid pressure to control regurgitation of stomach contents during induction of anaesthesia. Lancet. 1961;2:404–406.
208 Cohen IT, Finkel JC, Hannallah RS, Hummer KA, Patel KM. The effect of fentanyl on the emergence characteristics after desflurane or sevoflurane anesthesia in children. Anesth Analg. 2002;94:1178–1181.
209 Snow DG, Campbell JB, Morgan DW. The microbiology of peritonsillar sepsis. J Laryngol Otol. 1991;105:553–555.
210 Herzon FS. Harris P. Mosher Award thesis. Peritonsillar abscess: incidence, current management practices, and a proposal for treatment guidelines. Laryngoscope. 1995;105:1–17.
211 Suskind DL, Park J, Piccirillo JF, Lusk RP, Muntz HR. Conscious sedation: a new approach for peritonsillar abscess drainage in the pediatric population. Arch Otolaryngol Head Neck Surg. 1999;125:1197–1200.
212 Woods AM. Pediatric bronchoscopy, bronchography, and laryngoscopy. In: Berry FA, Jr., eds. Anesthetic management of difficult and routine pediatric patients. New York: Churchill Livingstone; 1986:189–250.
213 Rolf N, Coté CJ. Persistent cardiac arrhythmias in pediatric patients: effects of age, expired carbon dioxide values, depth of anesthesia, and airway management. Anesth Analg. 1991;73:720–724.
214 Zalzal GH. Stridor and airway compromise. Pediatr Clin North Am. 1989;36:1389–1402.
215 Myer CM3, O’Connor DM, Cotton RT. Proposed grading system for subglottic stenosis based on endotracheal tube sizes. Ann Otol Rhinol Laryngol. 1994;103:319–323.
216 Sanders RB. Two ventilating attachments for bronchoscopes. Del Med. 1967:170–192.
217 Jaquet Y, Monnier P, Van MG, et al. Complications of different ventilation strategies in endoscopic laryngeal surgery: a 10-year review. Anesthesiology. 2006;104:52–59.
218 Koomen E, Poortmans G, Anderson BJ, Janssens MM. Jet ventilation for laryngotracheal surgery in an ex-premature infant. Paediatr Anaesth. 2005;15:786–789.
219 Arthurton MW. Stridor in a paediatric department. Proc R Soc Med. 1970;63:712–714.
220 Skolnik NS. Treatment of croup. A critical review. Am J Dis Child. 1989;143:1045–1049.
221 Downes JJ, Raphaely RC. Pediatric intensive care. Anesthesiology. 1975;43:238–250.
222 Hannallah R. Epiglottitis. In: Stehling LC, ed. Common problems in pediatric anesthesia. 2nd ed. St Louis: Mosby-Year Book; 1992:277–281.
223 Neto GM, Kentab O, Klassen TP, Osmond MH. A randomized controlled trial of mist in the acute treatment of moderate croup. Acad Emerg Med. 2002;9:873–879.
224 Taussig LM, Castro O, Beaudry PH, Fox WW, Bureau M. Treatment of laryngotracheobronchitis (croup). Use of intermittent positive-pressure breathing and racemic epinephrine. Am J Dis Child. 1975;129:790–793.
225 Drugs for pediatric emergencies. Committee on Drugs [no authors listed]. Pediatrics. 1998;101:e13.
226 Henry RL, Mellis CM, Benjamin B. Pseudomembranous croup. Arch Dis Child. 1983;58:180–183.
227 Friedman EM, Jorgensen K, Healy GB, McGill TJ. Bacterial tracheitis–two-year experience. Laryngoscope. 1985;95:9–11.
228 Wagener JS, Landau LI, Olinsky A, Phelan PD. Management of children hospitalized for laryngotracheobronchitis. Pediatric Pulmonology. 1986;2:159–162.
229 Downes JJ, Striker TW, Stool S. Complications of nasotracheal intubation in children with croup. N Engl J Med. 1966;274:226–227.
230 McEniery J, Gillis J, Kilham H, Benjamin B. Review of intubation in severe laryngotracheobronchitis. Pediatrics. 1991;87:847–853.
231 Allen TH, Steven IM. Prolonged nasotracheal intubation in infants and children. Br J Anaesth. 1972;44:835–840.
232 Rittichier KK. The role of corticosteroids in the treatment of croup. Treat Respir Med. 2004;3:139–145.
233 Bjornson CL, Klassen TP, Williamson J, et al. A randomized trial of a single dose of oral dexamethasone for mild croup 1. N Engl J Med. 2004;351:1306–1313.
234 Cherry JD. State of the evidence for standard-of-care treatments for croup: are we where we need to be? Pediatr Infect Dis J. 2005;24:S198–S202. discussion
235 Russell KF, Liang Y, O’Gorman K, Johnson DW, Klassen TP. Glucocorticoids for croup. Cochrane Database Syst Rev. 2011. CD001955
236 Ausejo M, Saenz A, Pham B, et al. The effectiveness of glucocorticoids in treating croup: meta-analysis. West J Med. 1999;171:227–232.
237 Chub-Uppakarn S, Sangsupawanich P. A randomized comparison of dexamethasone 0.15 mg/kg versus 0.6 mg/kg for the treatment of moderate to severe croup. Int J Pediatr Otorhinolaryngol. 2007;71:473–477.
238 Emmerson SG, Richman B, Spahn T. Changing patterns of epiglottitis in children. Otolaryngol Head Neck Surg. 1991;104:287–292.
239 Wurtele P. Acute epiglottitis in children and adults: a large-scale incidence study. Otolaryngol Head Neck Surg. 1990;103:902–908.
240 Crysdale WS, Sendi K. Evolution in the management of acute epiglottitis: a 10-year experience with 242 children. Int Anesthesiol Clin. 1988;26:32–38.
241 Adams WG, Deaver KA, Cochi SL, et al. Decline of childhood Haemophilus influenzae type b (Hib) disease in the Hib vaccine era. JAMA. 1993;269:221–226.
242 Hickerson SL, Kirby RS, Wheeler JG, Schutze GE. Epiglottitis: a 9-year case review. South Med J. 1996;89:487–490.
243 Faden H. The dramatic change in the epidemiology of pediatric epiglottitis. Pediatr Emerg Care. 2006;22:443–444.
244 Rafei K, Lichenstein R. Airway infectious disease emergencies. Pediatr Clin North Am. 2006;53:215–242.
245 Sharma N, Berman DM, Scott GB, Josephson G. Candida epiglottitis in an adolescent with acquired immunodeficiency syndrome. Pediatr Infect Dis J. 2005;24:91–92.
246 Eckmann DM, Seligman I, Cote CJ, Hussong JW. Mucormycosis supraglottitis on induction of anesthesia in an immunocompromised host. Anesth Analg. 1998;86:729–730.
247 McVernon J, Slack MP, Ramsay ME. Changes in the epidemiology of epiglottitis following introduction of Haemophilus influenzae type b (Hib) conjugate vaccines in England: a comparison of two data sources. Epidemiol Infect. 2006;134:570–572.
248 Rogers DJ, Sie KC, Manning SC. Epiglottitis due to nontypeable Haemophilus influenzae in a vaccinated child. Int J Pediatr Otorhinolaryngol. 2010;74:218–220.
249 Communicable Disease Report. Unvaccinated child dies of Haemophilus influenzae type b infection. CDR Weekly [no authors listed]. Commun Dis Rep CDR Wkly. 1999;9:167–170.
250 Frantz TD, Rasgon BM. Acute epiglottitis: changing epidemiologic patterns. Otolaryngol Head Neck Surg. 1993;109:457–460.
251 Coté CJ, Eavey RD, Todres ID, Jones DE. Cricothyroid membrane puncture: oxygenation and ventilation in a dog model using an intravenous catheter. Crit Care Med. 1988;16:615–619.
252 Coté CJ, Hartnick CJ. Pediatric transtracheal and cricothyrotomy airway devices for emergency use: which are appropriate for infants and children? Paediatr Anaesth. 2009;19(Suppl 1):66–76.
253 Guardiani E, Bliss M, Harley E. Supraglottitis in the era following widespread immunization against Haemophilus influenzae type B: evolving principles in diagnosis and management. Laryngoscope. 2010;120:2183–2188.
254 Shah RK, Roberson DW, Jones DT. Epiglottitis in the Hemophilus influenzae type B vaccine era: changing trends. Laryngoscope. 2004;114:557–560.
255 Knight GJ, Harris MA, Parbari M, O’Callaghan MJ, Masters IB. Single daily dose ceftriaxone therapy in epiglottitis. J Paediatr Child Health. 1992;28:220–222.
256 Shah K, Kashima H, Polk BF, et al. Rarity of cesarean delivery in cases of juvenile-onset respiratory papillomatosis. Obstet Gynecol. 1986;68:795–799.
257 Mudry P, Vavrina M, Mazanek P, et al. Recurrent laryngeal papillomatosis: successful treatment with human papillomavirus vaccination. Arch Dis Child. 2011;96:476–477.
258 Syrjanen S. Current concepts on human papillomavirus infections in children. APMIS. 2010;118:494–509.
259 Mammas IN, Sourvinos G, Spandidos DA. Human papilloma virus (HPV) infection in children and adolescents. Eur J Pediatr. 2009;168:267–273.
260 Freed GL, Derkay CS. Prevention of recurrent respiratory papillomatosis: role of HPV vaccination. Int J Pediatr Otorhinolaryngol. 2006;70:1799–1803.
261 Leventhal BG, Kashima HK, Mounts P, et al. Long-term response of recurrent respiratory papillomatosis to treatment with lymphoblastoid interferon alfa-N1. Papilloma Study Group. N Engl J Med. 1991;325:613–617.
262 Li SQ, Chen JL, Fu HB, Xu J, Chen LH. Airway management in pediatric patients undergoing suspension laryngoscopic surgery for severe laryngeal obstruction caused by papillomatosis. Paediatr Anaesth. 2010;20:1084–1091.
263 Sosis MB. Anesthesia for laser surgery. Anesth Clin N Am. 1993;11:537–552.
264 Weeks DB. Laboratory and clinical description of the use of jet-venturi ventilator during laser microsurgery of the glottis and subglottis. Anesth Rev. 1985;12:32–36.
265 Skinner DW, Chui P. The hazards of ‘button-sized’ batteries as foreign bodies in the nose and ear. J Laryngol Otol. 1986;100:1315–1318.
266 Yasny JS. Nasal foreign bodies in children: considerations for the anesthesiologist. Paediatr Anaesth. 2011.
267 Black RE, Johnson DG, Matlak ME. Bronchoscopic removal of aspirated foreign bodies in children. J Pediatr Surg. 1994;29:682–684.
268 Cohen SR, Herbert WI, Lewis GB, Jr., Geller KA. Foreign bodies in the airway. Five-year retrospective study with special reference to management. Ann Otol Rhinol Laryngol. 1980;89:437–442.
269 Cataneo AJ, Reibscheid SM, Ruiz Junior RL, Ferrari GF. Foreign body in the tracheobronchial tree. Clin Pediatr (Phila). 1997;36:701–706.
270 Solanki DR, Suresh M, Ethridge HC. The effects of intravenous cimetidine and metoclopramide on gastric volume and pH. Anesth Analg. 1984;63:599–602.
271 Meretoja OA, Taivainen T, Raiha L, Korpela R, Wirtavuori K. Sevoflurane-nitrous oxide or halothane-nitrous oxide for paediatric bronchoscopy and gastroscopy. Br J Anaesth. 1996;76:767–771.
272 Hannallah R, Rosales JK. Laryngeal web in an infant with tracheoesophageal fistula. Anesthesiology. 1975;42:96–97.
273 Zalzal GH, Cotton RT. Glottic and subglottic stenosis. In: Cummings CW, ed. Otolaryngology, head & neck surgery. 4th ed. St Louis: Mosby; 2005:4266–4283.
274 Silver AL, Yager P, Purohit P, Noviski N, Hartnick CJ. Dexmedetomidine use in pediatric airway reconstruction. Otolaryngol Head Neck Surg. 2011;144:262–267.
275 Tasca RA, Clarke RW. Tracheocutaneous fistula following paediatric tracheostomy—a 14-year experience at Alder Hey Children’s Hospital. Int J Pediatr Otorhinolaryngol. 2010;74:711–712.
276 Schroeder JW, Jr., Greene RM, Holinger LD. Primary closure of persistent tracheocutaneous fistula in pediatric patients. J Pediatr Surg. 2008;43:1786–1790.
277 Stern Y, Cosenza M, Walner DL, Cotton RT. Management of persistent tracheocutaneous fistula in the pediatric age group. Ann Otol Rhinol Laryngol. 1999;108:880–883.
278 Priestley JD, Berkowitz RG. Closure of tracheocutaneous fistula in children. Int J Pediatr Otorhinolaryngol. 2006;70:1357–1359.
279 Eaton DA, Brown OE, Parry D. Simple technique for tracheocutaneous fistula closure in the pediatric population. Ann Otol Rhinol Laryngol. 2003;112:17–19.
280 Geyer M, Kubba H, Hartley B. Experiences of tracheocutaneous fistula closure in children: how we do it. Clin Otolaryngol. 2008;33:367–369.
281 Licameli GR, Marsh BR, Tunkel DE. A simple method for closure of tracheocutaneous fistula in children. Arch Otolaryngol Head Neck Surg. 1997;123:1066–1068.
282 Goldsmith AJ, Abramson AL, Myssiorek D. Closure of tracheocutaneous fistula using a modified cutaneous Z-plasty. Am J Otolaryngol. 1993;14:240–245.
283 Bressler KL, Kaiser PC, Dunham ME, Holinger LD. Primary closure of persistent tracheocutaneous fistula in children. Ann Otol Rhinol Laryngol. 1994;103:835–837.
284 Mohan VK, Kashyap L, Verma S. Life threatening subcutaneous emphysema following surgical repair of tracheocutaneous fistula. Paediatr Anaesth. 2003;13:339–341.
285 White AK, Smitheringale AJ. Treatment of tracheocutaneous fistulae in children. J Otolaryngol. 1989;18:49–52.
286 Wheeler WB, Kurachek SC, Lobas JG, Lipscomb TS. Respiratory complications of tracheocutaneous fistula closure. Crit Care Med. 1991;19:580–582.
287 Keenan JP, Snyder GG, III., Lehmann WB, Ruiz JW. Management of tracheocutaneous fistula. Arch Otolaryngol. 1978;104:530–531.
288 Wiel E, Fayoux P, Vilette B. Complications of surgical closure of tracheo-cutaneous fistula in pediatric patients: two case reports. Int J Pediatr Otorhinolaryngol. 2000;52:97–99.
289 Merritt RM, Bent JP, Porubsky ES. Acute laryngeal trauma in the pediatric patient. Ann Otol Rhinol Laryngol. 1998;107:104–106.
290 Gold SM, Gerber ME, Shott SR, Myer CM, III. Blunt laryngotracheal trauma in children. Arch Otolaryngol Head Neck Surg. 1997;123:83–87.
291 Schaefer SD. Use of CT scanning in the management of the acutely injured larynx. Otolaryngol Clin North Am. 1991;24:31–36.
292 Catlin FI, Grimes WJ. The effect of steroid therapy on recovery from tonsillectomy in children. Arch Otolaryngol Head Neck Surg. 1991;117:649–652.
293 Ohlms LA, Wilder RT, Weston B. Use of intraoperative corticosteroids in pediatric tonsillectomy. Arch Otolaryngol Head Neck Surg. 1995;121:737–742.
294 Tom LW, Templeton JJ, Thompson ME, Marsh RR. Dexamethasone in adenotonsillectomy. Int J Pediatr Otorhinolaryngol. 1996;37:115–120.
295 April MM, Callan ND, Nowak DM, Hausdorff MA. The effect of intravenous dexamethasone in pediatric adenotonsillectomy. Arch Otolaryngol Head Neck Surg. 1996;122:117–120.