CHAPTER 39 Osteoporosis
INTRODUCTION
Osteoporosis is a systemic skeletal disease characterized by compromised bone strength that predisposes the skeleton to fracture. The strength of bone is related to the mass of the bone, the distribution of the mass, the microarchitectural alignment, the age of the bone, and the quality of the bone. The connectivity and microarchitectural alignment of the bone matrix play a critical role in providing strength to the bone. Niebur and colleagues have clearly demonstrated that loss of connectivity or the presence of sites of limited dimension increase the likelihood of local trabecular fracture.1 At the onset of bone formation, the initial bone that is deposited is undermineralized with respect to the final bone product and over time there is increased mineralization and saturation with crystal. The strength of bone increases with aging as a result of this maturation process. High-turnover bone states are associated with increased risk of fracture. Biochemically, bone is a composite material consisting of collagen and mineral. Collagen provides tensile strength, while mineral provides compressive strength. Collagen is biased in its orientation and bone is stronger along the primary axis of the collagen fibers and weaker in the transverse axis. In disease states of altered bone mineral content, such as osteomalacia, rickets, and osteogenesis imperfecta, the strength of bone can be seriously compromised. Consequently, both the quantity and quality of bone profoundly affect the overall strength of bone. Altered performance in either of these two areas can lead to an increased risk of low-energy fracture.
FRACTURE RISK
In the year 2002 an estimated 30 million women aged 50 or older had osteoporosis or were at risk of developing this condition (National Osteoporosis Foundation data). Fifty-five percent of people aged 55 and older have either osteoporosis or low bone mass. With the aging of the population this trend will increase. The lifetime risk of fracture of the hip, wrist, and spine approximates 40%.2–4
BONE BIOLOGY
All individuals increase their bone mass after birth with a peak gain in bone mass occurring at the onset of rapid growth and during adolescence. Final peak bone mass is achieved between the ages of 25 and 30.4 Men can expect to achieve a higher peak bone mass than women. Certain ethnic groups such as African-Americans have greater bone mass than do Caucasian Americans. Once peak bone mass is achieved in women, there is a plateauing of bone mass followed by a very gradual decline in bone mass of approximately 0.3% per year until menopause. At menopause, the rate of bone loss will accelerate and women will lose 2% per year for a short period of time and then resume the gradual decline thereafter. After achieving peak bone mass, men also lose bone gradually. During the period of greatest bone loss, trabecular bone is resorbed at least eight times greater than cortical bone due to the increased surface area of trabecular bone. Fortunately, in an effort to compensate for this loss of bone, the endosteal bone is preferentially lost, leaving the bulk of the bone at the greatest distance from the axial center which takes advantage of the moments of inertia to partially compensate for loss of bone. There is also a gradual increase in the dimensions of the cortical side of bone to partially compensate for this diminished mass. These findings are most evident in the diaphysis of long bones, and are less visible in the metaphyseal regions and in bones with a high proportion of trabecular bone. As bone is resorbed, the individual trabeculae begin to narrow and sites of prominent osteoclastic resorption begin to develop. These areas eventually lead to disconnectivity of the trabeculae. Since these areas of bone loss are not recognized as fractures, there is no effort on the part of the body to heal these trabeculae. As a result, the interconnectivity of the trabeculae declines in the trabecular bone, particularly during times of rapid resorption. The collagen in bone is continuously being turned over through osteoclastic bone resorption. This process is driven by the metabolic state or the presence of a site of imperfection. Osteoclasts work to carve out an area of Howship’s lacunae along the trabeculae or penetrating into the cortical bone. Following osteoclastic resorption, osteoblasts will begin to reestablish the skeleton. After the age of 40, this metabolic bone disease unit is incompletely reconstituted, leading to a net loss of bone through every cycle.
BONE TURNOVER
Calcium and vitamin D metabolism are intimately related and are both a part of the bone turnover cycle. Vitamin D is produced in the skin from 1 hour of sunlight or can be obtained by dietary supplementation. Vitamin D itself is made in the skin and is then transported to the liver where it is converted to 25-hydroxy vitamin D. This form of vitamin D has a half-life of 3 days. Alternatively, the liver can degrade vitamin D by activating the P-450 hydrolase system. Certain drugs that activate the P-450 system can lead to enhanced vitamin D catabolism and in some cases vitamin D deficiency. In particular, this has been well documented with the barbiturates and the seizure medications.5 In the face of low serum calcium levels, the human body activates parathyroid hormone, which travels to the kidney where it stimulates the conversion of 25-hydoxy vitamin D into the active vitamin D metabolite, 1,25 dihydroxy vitamin D. Activated vitamin D will then stimulate the kidney to retain calcium and excrete phosphate. The 1,25 dihydroxy vitamin D also travels to the intestine where it activates a cascade that ultimately leads to the increased absorption of calcium from the diet. Parathyroid hormone also travels to the bone where it activates bone resorption. Osteoblasts have receptors for parathyroid hormone and initiate the Rank-L system that ultimately leads to the development of new osteoclasts via activation of preosteoclasts. Thus, calcium can be elevated by retaining calcium from the kidney, increasing calcium absorption from the gut, and releasing calcium by catabolizing the skeleton. Vitamin D has several other functions, most notably the physiological differentiation of osteoclasts. Vitamin D is also critical in calcium membrane transport in skeletal muscle. Disorders of the kidney such as renal osteodystrophy, which leads to destruction of the kidney, will lower the production of 1,25 dihydroxy vitamin D. Intestinal absorption is compromised in malabsorption syndromes, most notably celiac sprue, Crohn’s disease, and steroid overdose.6 In fact, serological evidence of celiac sprue is found in approximately 9% of osteoporotic patients.7
Calcium plays a critical role in bone homeostasis. As an intracellular signaler, calcium is tightly regulated by the body’s homeostatic mechanisms, and the body will maintain calcium levels at the expense of other tissues. The requirements of calcium vary with age and activity. Simple dairy products such as an 8-ounce glass of milk or a container of yogurt contain 280 mg of calcium. Certain foods rich in calcium, such as spinach, are unavailable for absorption. This results from the high oxalate content in spinach which binds the calcium and prevents its absorption. Calcium requirements for a growing child are 715 mg per day. From the ages of 10 to 25, 1300 mg of calcium are required per day. A healthy adult needs 800 mg per day. A pregnant woman requires 1500 mg of calcium per day, and this requirement increases to 2000 mg per day in lactating women. A postmenopausal woman needs 1500 mg per day, and any individual experiencing negative nitrogen balance or recovering from a major fracture requires 1500 mg per day.8,9
There are a number of drugs that interfere with calcium retention, including cortical steroids, heparin, isoniazid, tetracycline, furosemide, and very large doses of caffeine. Vitamin D nutrition remains critical and studies have demonstrated the efficacy of calcium in the prevention of fractures. Calcium is typically supplemented in conjunction with Vitamin D. A recent review by Dawson-Hughes has indicated that the RDA of 400 units of vitamin D may in fact be insufficient.10 Eight hundred units, particularly in the older patient, may be more reasonable and appears to be the cutoff point at which improved muscle function and a subsequent decrease in falls and fractures were identified. Vitamin D in doses of up to 2000 units per day appear to be safe except in individuals with noted history of multiple kidney stones. Vitamin D can be given either as vitamin D2 or D3 or as final pathway products (1,25 dihydroxy vitamin D). The former will last for 2 months while the latter has a 4-hour half-life. Drugs with hydroxyl groups are degraded in the liver and often activate the P-450 hydrolase system leading to premature degradation of vitamin D. Most notable in this group are the antiepileptic seizure medications. In patients taking antiepileptics or any other drug that is degraded in the liver, vitamin D metabolism may be disrupted and higher dosages of vitamin D may be warranted.11
Peak bone mass is very closely associated with menstrual status. Women who have normal menstrual cycles of 10 episodes per year of approximately 4 days per episode are able to maintain their skeletal integrity and will lose bone at a maximal rate of 0.3% per year.12 This loss can be partially compensated with better nutrition and supplemental calcium. Women who are amenorrheic or who have irregular menstrual cycles may lose bone at a rate of up to 2% per year. In the young amenorrheic individual who has yet to achieve peak bone mass, there is a diminished ability to gain bone at precisely the time when one should be increasing her peak bone mass. Primary amenorrhea is defined as the absence of menses before the age of 16; however, women who have irregular or no periods after the age of 14 are susceptible to compromises of skeletal integrity. Common disorders that affect the menstrual cycle are low body weight, eating disorders, and excessive exercise. Young athletes who lack a normal menstrual cycle have an increased rate of stress fracture compared to women who have a normal menstrual cycle.13 Birth control pills, while reestablishing cycles, may not be totally successful in protecting the skeleton.14 Low body mass is frequently the common factor in most of these disorders and good nutrition is critical, particularly during skeleton growth and development.
Mechanical factors affect bone mass. Bone responds to mechanical load by increasing bone mass and remodeling along lines of increased stress. Bone that lacks stress suffers a decline in bone mass. Normal load bearing will maintain the skeleton. Elevated loading will lead to changes in the skeleton as well. This is found primarily in bones of younger individuals with thick periosteum and decreases with aging and in those bones with limited periosteum. Very high loads overcome the ability of the body to adjust and can lead to stress fractures. In growing individuals up to the age of 30, exercise can lead to enhanced bone mass. High-impact programs have led to almost an 8% increase in bone mass compared to peers without similar programs.15 Once individuals reach a postmenopausal state, exercise rarely changes bone density. However, detailed studies have suggested that bone turnover is in fact decreased in such individuals. They have a lower rate of falling and, when corrected for the number of falls, the fracture rate is decreased. N-telopeptide, a measure of bone turnover, is similarly decreased in those individuals who exercise.16
BONE MASS
Low bone mass is the single most accurate predictor of increased fracture risk. Bone mineral density is used to establish a diagnosis of postmenopausal osteoporosis and can predict future fracture risk. In the National Osteoporosis Risk Assessment (NORA) study, approximately 150 000 women were evaluated for 1 year in order to define a critical role for bone density in predicting fracture risk.17 Results from this study suggested a strong continuous relationship between lower bone mineral density and higher fracture rate as expressed by the number of women who experienced fractured vertebrae. Fracture rates were highest among women with the lowest T-scores. Bone density can be determined by dual-energy X-ray absorptiometry (DEXA scan), quantitative computed tomography (q-CT), peripheral measurements, and ultrasound. Of these modalities, DEXA (Fig. 39.1) is the most accurate and is considered to be the gold standard.18 Furthermore, DEXA is the most common form of bone density utilized in centers that measure the area of bone density of the spine and several parameters within the hip, most notably, the total femur, femoral neck, and intertrochanteric area. During DEXA scanning, an intervertebral assessment of the spine (Fig. 39.2) can be performed to assess the anatomic detail of the thoracic and lumbar spine and determine if vertebral compression is present. The area of mineral content is then compared with age-corrected peers in order to obtain a Z-value and then with an ideal peak bone mass in a normal individual not corrected for age to obtain a T-value. The precision of DEXA is approximately 2% in the spine and a little over 3% in the hip. It is important to recognize that the presence of scoliosis or arthritis can artificially elevate DEXA scores in the spine. The precision of q-CT is less than that of DEXA and the radiation exposure is greater. Peripheral bone mass evaluations have only a 70% correlation with the hip and the spine. The World Health Organization has defined a normal bone density as a T-value of less than 1 standard deviation compared to normals.19 Osteopenia is defined as bone mass between 1 standard deviation and 2.4 standard deviations below normal. Osteoporosis is classified as a bone deficiency of 2.5 or more standard deviations. The risk of fracture is increased twofold in the spine and 2.5 times in the hip for each standard deviation below peak bone mass.20 In recent protocols, however, these values are used for epidemiological and demographic studies, and treatment protocols call for an earlier intervention with far less bone deficiency. The Z-value becomes critical in differentiating primary osteoporosis from secondary forms of osteoporosis. In particular, Z values of 1.5 or worse should be evaluated for secondary forms of osteoporosis. Strength of bone is also related to turnover and other aspects of bone quality. While the fracture rate is greatest in those individuals with the lowest bone mass, there are individuals with apparently relatively normal levels of bone mass who in fact have vertebral and hip fractures. Patients with hip or vertebral fractures are more likely to experience future fractures than are patients with no fractures. The risk of vertebral fracture rises rapidly with age for both men and women. In the United States women are two to three times more likely to experience a vertebral fracture than men, but when corrected for age, this disparity disappears.
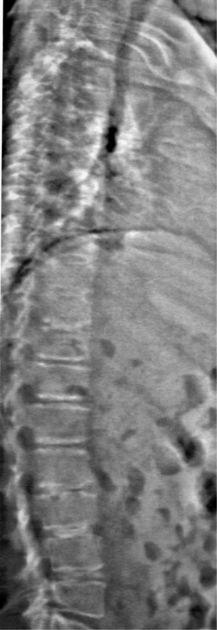
Fig. 39.2 An intervertebral assessment of the spine can be obtained during DEXA scanning to provide additional anatomic detail of the thoracic and lumbar spine and allow for the identification of vertebral compression fractures. The film is from the same patient as in Figure 39.1.
Biochemical markers have been introduced to evaluate and differentiate between high- and low-turnover osteoporosis. Bone resorption markers include collagen breakdown products – most notably, the N-telopeptides and C-telopeptides in the pyridinoline collagen cross-link products. These components are not degraded by the body and can be detected in both the serum and the urine. Bone formation markers are more popularly directed toward bone-specific alkaline phosphatase and osteocalcin. Bone resorption markers appear to have better correlation with fracture risk. The bone formation markers are disease specific and often used to test the efficacy of treatment interventions. The overall risk for a low-energy fracture is related to high-turnover states and low bone mass. Additional independent risk factors include low body weight, recent loss of body weight, personal history of fragility fracture, a first-degree relative with a fragility fracture, and smoking.21