CHAPTER 15 Osteochondral Lesions of the Talar Dome
New Horizons in Cartilage Replacement
Osteochondral lesions of the talar dome larger than 2.5 to 3.0 cm2 pose a special problem in the young and middle-aged population (Figs. 15-1 to 15-3). These lesions affect the articular cartilage of the talar dome and the underlying subchondral bone.1 If encountered acutely or if a large fragment exists, some of these lesions can be stabilized and internally fixed with metallic or bioabsorbable implants. Success rates of 78% (range, 40% to 100%) have been reported with reduction and fixation of the osteochondral fragments.2 Most of the acute lesions suitable for internal fixation are laterally located and are usually anterior on the talar dome, making them relatively easy to access using a small, anterolateral arthrotomy incision or arthroscopic techniques.
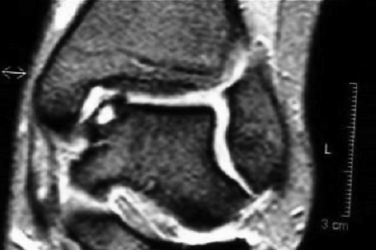
FIGURE 15-2 Anteroposterior magnetic resonance imaging shows an osteochondral lesion of the medial talus.
Unfortunately, patients with these lesions represent a small proportion of those who present for treatment of symptomatic osteochondral lesions of the talar dome. Most have chronic, medial lesions, which tend to occur more posteriorly on the talar dome. They are more difficult to access and usually require a medial malleolar osteotomy for open exposure. The posteromedial lesions more commonly manifest as chronic lesions that have greater depth than their lateral counterparts and that demonstrate degenerated articular cartilage with necrotic supporting subchondral bone.
COLLAGEN-COVERED AUTOLOGOUS CHONDROCYTE IMPLANTATION
In a prospective study presented at the ICRS meeting in 2004, Steinwachs17 described 163 patients treated for chondral defects in the knee with ACI using a periosteal flap or the Chondro-Gide membrane instead of the periosteal patch. At approximately 3 years of follow-up, 78% of patients in the periosteal group reported good or excellent results, and 88% of patients in the Chondro-Gide group reported good or excellent results. Statistical significance was not discussed by the investigator. There were no cases of membrane hypertrophy.17
MATRIX/MEMBRANE-INDUCED AUTOLOGOUS CHONDROCYTE IMPLANTATION
Matrix/membrane-induced autologous chondrocyte implantation (MACI) is a second-generation chondrocyte implantation process. MACI is a new biotechnology in which cultured autologous chondrocytes are impregnated onto a highly purified, porcine collagen I/III membrane (Verigen AG, Genzyme Corp., Cambridge, MA) (Fig. 15-4). The MACI implant can be fixed to the chondral defect by fibrin glue (with little or no suture necessary), suture, or bioabsorbable pins or tacks. The procedure can be performed arthroscopically or by mini- arthrotomy. No periosteal graft is needed.
Open Technique
Chondrocytes are harvested arthroscopically from a non–weight-bearing area of the ipsilateral knee (200 to 300 mg of healthy cartilage). The chondrocytes are then cultured and expanded in vitro (3 to 5 weeks) and then impregnated on an absorbable, three-dimensional, bilayered, purified, porcine collagen I/III membrane. The bilayer structure has a smooth side that acts as a natural barrier and faces the joint. Chondrocytes are seeded on the porous side of the matrix. The membrane is tear resistant and can be templated and cut to shape. The membrane is nonantigenic (i.e., telopeptides are split during the manufacturing process), and it is bioabsorbable. The bioabsorbable membrane can be fixed to the ankle cartilage defect with fibrin glue, pins, or suture.
The talar dome lesion may be approached using a simple arthrotomy for an anterior lesion, or a malleolar osteotomy may be required to expose a middle to posterior talar lesion. The osteochondral defect is débrided, and the base is curetted to remove the calcified cartilage layer (Fig. 15-5). A stable cartilage rim with sharp vertical walls of healthy cartilage is created, and the chondral defect is templated for size and shape (Fig. 15-6). The MACI membrane is cut to the proper shape with a scalpel or scissors (Fig. 15-7). The membrane is then fixed with fibrin glue (Tisucol, Baxter, Spain) (Fig. 15-8). Suture or bioabsorbable pins, or both, may be used, but fibrin glue by itself is usually all that is needed (Figs. 15-9 and 15-10).18,19
Postoperatively, the patient is placed into a soft dressing, and continuous passive motion is initiated for 8 weeks, during which time the patient does not bear weight on the extremity. Patients with larger and more central lesions are not allowed to bear weight for 12 weeks.
Arthroscopic Technique
Placement of the graft is performed using a dry scope technique, which is done without fluid in the joint. Two small anchors with 5-0 absorbable sutures are then placed at opposite sides of the periphery of the cartilage lesion (i.e., 3 and 9 o’clock or 12 and 6 o’clock). The sutures are then passed through the MACI membrane outside the joint at points corresponding to the cartilage lesion (Figs. 15-11 to 15-13).