CHAPTER 30 Oral cavity
The mouth or oral cavity extends from the lips and cheeks externally to the anterior pillars of the fauces internally, where it continues into the oropharynx (Fig. 30.1). The mouth can be subdivided into the vestibule external to the teeth and the oral cavity proper internal to the teeth. The palate forms the roof of the mouth and separates the oral and nasal cavities. The floor of the mouth is formed by the mylohyoid muscles and is occupied mainly by the tongue. The lateral walls of the mouth are defined by the cheeks and retromolar regions. Three pairs of major salivary glands (parotid, submandibular and sublingual) and numerous minor salivary glands (labial, buccal, palatal, lingual) open into the mouth. The muscles in the oral cavity are associated with the lips, cheeks, floor of the mouth and tongue. The muscles of the lips and cheeks are described with the face in Chapter 29. The muscles of the soft palate are described with the pharynx in Chapter 33.
CHEEKS
The external features of the cheeks are described in Chapter 29. Internally, the mucosa of the cheek is tightly adherent to buccinator and is thus stretched when the mouth is opened and wrinkled when closed. Ectopic sebaceous glands may be evident as yellow patches (Fordyce’s spots). Their numbers increase in puberty and in later life.
LIPS
The external features of the lips are described in Chapter 29. The central part of the lips contains orbicularis oris. Internally, the labial mucosa is smooth and shiny and shows small elevations caused by underlying mucous glands.
The position and activity of the lips are important in controlling the degree of protrusion of the incisors. With normal (competent) lips, the tips of the maxillary incisors lie below the upper border of the lower lip, and this arrangement helps to maintain the ‘normal’ inclination of the incisors. When the lips are incompetent, the maxillary incisors may not be so controlled and the lower lip may even lie behind them, thus producing an exaggerated proclination of these teeth. A tight, or overactive, lip musculature may be associated with retroclined maxillary incisors. The lips are kept moist both by tongue deposition of saliva and by numerous minor salivary glands within them. These glands are liable to trauma by the teeth, particularly in the lower lip: this can produce a mucocele as a result of either extravasation of saliva into the submucosal tissues or retention of saliva within the gland or its duct.
ORAL VESTIBULE
The oral vestibule is a slit-like space between the lips or cheeks on one side and the teeth on the other. When the teeth occlude, the vestibule is a closed space that only communicates with the oral cavity proper in the retromolar regions behind the last molar tooth on each side. Where the mucosa that covers the alveolus of the jaw is reflected onto the lips and cheeks, a trough or sulcus is formed which is called the fornix vestibuli. A variable number of sickle-shaped folds containing loose connective tissue run across the fornix vestibuli. In the midline these are the upper and lower labial frena (or frenula). Other folds may traverse the fornix near the canines or premolars. The folds in the lower fornix are said to be more pronounced than those in the upper fornix (Fig. 30.2).
ORAL MUCOSA
MASTICATORY MUCOSA AND THE GINGIVAE
Vascular supply and lymphatic drainage
The lymph vessels of the labial and buccal gingivae of the maxillary and mandibular teeth unite to drain into the submandibular nodes, though in the labial region of the mandibular incisors they may drain into the submental lymph nodes. The lingual and palatal gingivae drain into the jugulodigastric group of nodes, either directly or indirectly through the submandibular nodes.
Innervation
The nerves supplying the gingivae in the upper jaw come from the maxillary nerve via its greater palatine, nasopalatine, and anterior, middle and posterior superior alveolar branches (see Table 30.2). Surgical division of the nasopalatine nerve, for example during the removal of an ectopic canine tooth, causes no obvious sensory deficit in the anterior part of the palate, which suggests that the territory of the greater palatine nerve reaches as far forwards as the gingivae lingual to the incisor teeth or the nerve has large regenerative potential. The mandibular nerve innervates the gingivae in the lower jaw by its inferior alveolar, lingual and buccal branches.
OROPHARYNGEAL ISTHMUS
The oropharyngeal isthmus lies between the soft palate and the dorsum of the tongue, and is bounded on both sides by the palatoglossal arches. Each palatoglossal arch runs downwards, laterally and forwards, from the soft palate to the side of the tongue and consists of palatoglossus and its covering mucous membrane (Fig. 30.3). The approximation of the arches shuts off the mouth from the oropharynx, and is essential to deglutition (see Ch. 33).
FLOOR OF THE MOUTH
The floor of the mouth is a small horseshoe-shaped region situated beneath the movable part of the tongue and above the muscular diaphragm formed by the mylohyoid muscles (Fig. 30.4, see Fig. 30.7). A fold of tissue, the lingual frenulum, extends onto the inferior surface of the tongue from near the base of the tongue. It occasionally extends across the floor of the mouth to be attached onto the mandibular alveolus, known colloquially as a ‘tongue tie’; historically, this has been removed to aid speech, but the evidence for this is scanty. The submandibular salivary ducts open into the mouth at the sublingual papilla (caruncle), which is a large centrally positioned protuberance at the base of the tongue.
The sublingual folds lie on either side of the sublingual papilla and cover the underlying submandibular ducts and sublingual salivary glands. The blood supply of the floor of the mouth is described with the blood supply of the tongue (see p. 505). The main muscle forming the floor of the mouth is mylohyoid, with geniohyoid lying immediately above it.
Mylohyoid
Mylohyoid lies superior to the anterior belly of digastric and, with its contralateral fellow, forms a muscular floor for the oral cavity. It is a flat, triangular sheet attached to the whole length of the mylohyoid line of the mandible (Fig. 30.4A, see Fig. 30.7). The mylohyoid line is of variable length, sometimes ending before the lower third molar (wisdom) tooth. The posterior fibres of mylohyoid pass medially and slightly downwards to the front of the body of the hyoid bone near its lower border. The middle and anterior fibres from each side decussate in a median fibrous raphe that stretches from the symphysis menti to the hyoid bone. The median raphe is sometimes absent, in which case the two muscles form a continuous sheet, or it may be fused with the anterior belly of digastric. In about one-third of subjects there is a hiatus in the muscle through which a process of the sublingual gland protrudes.
Geniohyoid
Geniohyoid is a narrow muscle which lies above the medial part of mylohyoid (see Figs 30.6, 30.10). It arises from the inferior mental spine (genial tubercle) on the back of the symphysis menti, and runs backwards and slightly downwards to attach to the anterior surface of the body of the hyoid bone. The paired muscles are contiguous and may occasionally fuse with each other or with genioglossus.
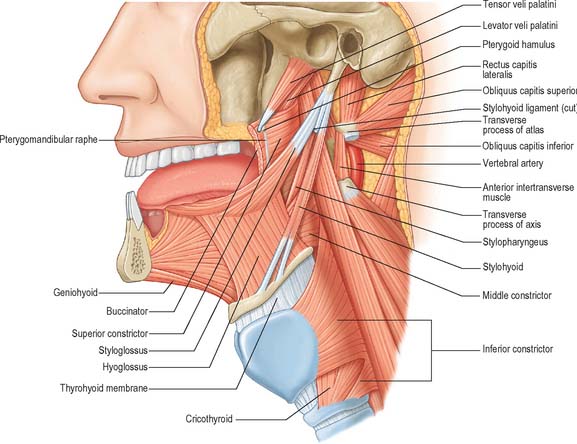
Fig. 30.6 Muscles of the tongue and pharynx. Palatoglossus is not shown here, but is depicted in Fig. 33.4.
PALATE
HARD PALATE
The hard palate is formed by the palatine processes of the maxillae and the horizontal plates of the palatine bones (see Fig. 30.14A). The hard palate is bounded in front and at the sides by the tooth-bearing alveolus of the upper jaw and is continuous posteriorly with the soft palate. It is covered by a thick mucosa bound tightly to the underlying periosteum. In its more lateral regions it also possesses a submucosa containing the main neurovascular bundle. The mucosa is covered by keratinized stratified squamous epithelium which shows regional variations and may be ortho- or parakeratinized.
The submucosa in the posterior half of the hard palate contains minor mucous-type salivary glands (Fig. 30.3). They secrete via numerous small ducts which often drain into a larger duct that opens bilaterally at the paired palatine foveae. These depressions, sometimes a few millimetres deep, flank the midline raphe at the posterior border of the hard palate. They provide a useful landmark for the extent of an upper denture; if not observed during construction of the denture they cause the denture to become unstable when the soft palate moves during deglutition and mastication. The upper surface of the hard palate is the floor of the nasal cavity and is covered by ciliated respiratory epithelium.
Vascular supply and lymphatic drainage of the hard palate
The veins of the hard palate accompany the arteries and drain largely to the pterygoid plexus.
Innervation of the hard palate
The sensory nerves of the hard palate are the greater palatine and nasopalatine branches of the maxillary nerve, which all pass through the pterygopalatine ganglion. The greater palatine nerve descends through the greater palatine canal, emerges on the hard palate from the greater palatine foramen, runs forwards in a groove on the inferior surface of the bony palate almost to the incisor teeth and supplies the gums and the mucosa and glands of the hard palate (Fig. 30.3). It also communicates with the terminal filaments of the nasopalatine nerve. As it leaves the greater palatine canal, it supplies palatine branches to both surfaces of the soft palate. The lesser (middle and posterior) palatine nerves, which are much smaller, descend through the greater palatine canal and emerge through the lesser palatine foramina in the tubercle of the palatine bone to supply the uvula, tonsil and soft palate. The nasopalatine nerves enter the palate at the incisive foramen and are branches of the maxillary nerve which pass through the pterygopalatine ganglion to supply the anterior part of the hard palate behind the incisor teeth.
TONGUE
ORAL (PRESULCAL) PART
The presulcal part of the tongue is located in the floor of the oral cavity. It has an apex touching the incisor teeth, a margin in contact with the gums and teeth, and a superior surface (dorsum) related to the hard and soft palates. On each side, in front of the palatoglossal arch, there are four or five vertical folds, the foliate papillae, which represent vestiges of larger papillae found in many other mammals. The dorsal mucosa has a longitudinal median sulcus and is covered by filiform, fungiform and circumvallate papillae (Fig. 30.5). The mucosa on the inferior (ventral) surface is smooth, purplish and reflected onto the oral floor and gums: it is connected to the oral floor anteriorly by the lingual frenulum. The deep lingual vein, which is visible, lies lateral to the frenulum on either side. The plica fimbriata (fimbriated fold), a fringed mucosal ridge directed anteromedially towards the apex of the tongue, lies lateral to the vein. This part of the tongue develops from the lingual swellings of the mandibular arch and from the tuberculum impar, and this embryological derivation explains its sensory innervation.
MUSCLES OF THE TONGUE
Genioglossus
Genioglossus is triangular in sagittal section, lying near and parallel to the midline. It arises from a short tendon attached to the superior genial tubercle behind the mandibular symphysis, above the origin of geniohyoid. From this point it fans out backwards and upwards (Fig. 30.6). The inferior fibres of genioglossus are attached by a thin aponeurosis to the upper anterior surface of the hyoid body near the midline (a few fasciculi passing between hyoglossus and chondroglossus to blend with the middle constrictor of the pharynx). Intermediate fibres pass backwards into the posterior part of the tongue, and superior fibres ascend forwards to enter the whole length of the ventral surface of the tongue from root to apex, intermingling with the intrinsic muscles. The muscles of opposite sides are separated posteriorly by the lingual septum. Anteriorly they are variably blended by decussation of fasciculi across the midline. The attachment of the genioglossi to the genial tubercles prevents the tongue from sinking back and obstructing respiration, therefore anaesthetists pull the mandible forward to obtain the full benefit of this connection.
Hyoglossus
Hyoglossus is thin and quadrilateral, and arises from the whole length of the greater cornu and the front of the body of the hyoid bone (Fig. 30.6). It passes vertically up to enter the side of the tongue between styloglossus laterally and the inferior longitudinal muscle medially. Fibres arising from the body of the hyoid overlap those from the greater cornu.
Styloglossus
Styloglossus is the shortest and smallest of the three styloid muscles (Fig. 30.6). It arises from the anterolateral aspect of the styloid process near its apex, and from the styloid end of the stylomandibular ligament. Passing downwards and forwards, it divides at the side of the tongue into a longitudinal part, which enters the tongue dorsolaterally to blend with the inferior longitudinal muscle in front of hyoglossus, and an oblique part, overlapping hyoglossus and decussating with it.
Stylohyoid ligament
The stylohyoid ligament is a fibrous cord which extends from the tip of the styloid process to the lesser cornu of the hyoid bone (Fig. 30.6). It gives attachment to some fibres of styloglossus and the middle constrictor of the pharynx and is closely related to the lateral wall of the oropharynx. Below it is overlapped by hyoglossus. The ligament is derived embryologically from the second branchial arch. It may be partially calcified.
Palatoglossus
Palatoglossus is closely associated with the soft palate in function and innervation, and is described with the other palatal muscles (see Ch. 33).
VASCULAR SUPPLY AND LYMPHATIC DRAINAGE OF THE TONGUE
Lingual artery
The tongue and the floor of the mouth are supplied chiefly by the lingual artery, which arises from the anterior surface of the external carotid artery. It passes between hyoglossus and the middle constrictor of the pharynx to reach the floor of the mouth accompanied by the lingual veins and the glossopharyngeal nerve. At the anterior border of hyoglossus, the lingual artery bends sharply upwards (Fig. 30.8). It is covered by the mucosa of the tongue and lies between genioglossus medially and the inferior longitudinal muscle laterally. Near the tip of the tongue, it anastomoses with its contralateral fellow; this contribution is important in maintaining the blood supply to the tongue in any surgical resection of the tongue. The branches of the lingual artery form a rich anastomotic network, which supplies the musculature of the tongue, and a very dense submucosal plexus. Named branches of the lingual artery in the floor of the mouth are the dorsal lingual, sublingual and deep lingual arteries.
Lymphatic drainage
The mucosa of the pharyngeal part of the dorsal surface of the tongue contains many lymphoid follicles aggregated into dome-shaped groups, the lingual tonsils. Each group is arranged around a central deep crypt, or invagination, which opens onto the surface epithelium. The ducts of mucous glands open into the bases of the crypts. Small isolated follicles also occur beneath the lingual mucosa. The lymphatic drainage of the tongue can be divided into three main regions, marginal, central and dorsal. The anterior region of the tongue drains into marginal and central vessels, and the posterior part of the tongue behind the circumvallate papillae drains into the dorsal lymph vessels. The more central regions may drain bilaterally, and this must be borne in mind when planning to remove malignant tumours of the tongue that are approaching the midline. If the tumour has a propensity for lymphatic spread, both cervical chains may be involved.
Marginal vessels
Vessels from the lateral margin of the tongue cross the sublingual gland, pierce mylohyoid and end in the submandibular nodes. Others end in the jugulodigastric or jugulo-omohyoid nodes. Vessels from the posterior part of the lingual margin traverse the pharyngeal wall to the jugulodigastric lymph nodes (Fig. 30.9).
INNERVATION OF THE TONGUE
The muscles of the tongue, with the exception of palatoglossus, are supplied by the hypoglossal nerve. Palatoglossus is supplied via the pharyngeal plexus (see Ch. 33). The pathways for proprioception associated with the tongue musculature are unknown, but presumably may involve the lingual, glossopharyngeal or hypoglossal nerves, and the cervical spinal nerves which communicate with the hypoglossal nerve.
Lingual nerve
The lingual nerve arises from the posterior trunk of the mandibular nerve in the infratemporal fossa (see Fig. 31.13A) where it is joined by the chorda tympani branch of the facial nerve and often by a branch of the inferior alveolar nerve. It then passes below the mandibular attachment of the superior pharyngeal constrictor and pterygomandibular raphe, closely applied to the periosteum of the medial surface of the mandible, until it lies opposite the distal (posterior) root of the third molar tooth, where it is covered only by the gingival mucoperiosteum. At this point it can contact the lingual cortical plate and may be at the level of, or higher than, the alveolar bone crest. It next passes medial to the mandibular attachment of mylohyoid, which carries it progressively away from the mandible, and separates it from the alveolar bone covering the mesial root of the third molar tooth, and then passes downward and forward on the deep surface of mylohyoid (i.e. the surface nearer the mucosa covering the floor of the mouth), crossing the lingual sulcus beneath the mucosa. In this position it lies on the deep portion of the submandibular gland which bulges over the top of the posterior border of mylohyoid. It passes below the submandibular duct which crosses it from medial to lateral, and curves upward, forward and medially to enter the tongue (Figs 30.8, 30.10). Within the tongue the lingual nerve lies first on styloglossus and then the lateral surface of hyoglossus and genioglossus, before dividing into terminal branches that supply the overlying lingual mucosa. The lingual nerve is connected to the submandibular ganglion (see Fig. 31.15) by two or three branches, and also forms connecting loops with twigs of the hypoglossal nerve at the anterior margin of hyoglossus.
The lingual nerve is at risk during surgical removal of (impacted) lower third molars, and, after such operations, up to 0.8% of patients may develop lingual sensory disturbance, which may persist in 0.3% (Robinson & Smith 1996). The nerve is also at risk during operations to remove the submandibular salivary gland, because the duct must be dissected from the lingual nerve, and because its connection to the submandibular ganglion pulls it into the operating field.
Glossopharyngeal nerve
The glossopharyngeal nerve is distributed to the posterior one-third of the tongue and the circumvallate papillae. It communicates with the lingual nerve. The course of the glossopharyngeal nerve in the neck is described in Chapter 28.
Hypoglossal nerve
The course of the hypoglossal nerve in the neck is described in Chapter 28. After crossing the loop of the lingual artery a little above the tip of the greater cornu of the hyoid, it inclines upwards and forwards on hyoglossus, passing deep to stylohyoid, the tendon of digastric and the posterior border of mylohyoid. Between mylohyoid and hyoglossus the hypoglossal nerve lies below the deep part of the submandibular gland, the submandibular duct and the lingual nerve, with which it communicates. It then passes onto the lateral aspect of genioglossus, continuing forwards in its substance as far as the tip of the tongue (Fig. 30.8). It distributes fibres to styloglossus, hyoglossus and genioglossus and to the intrinsic muscles of the tongue. If the nerve suffers either iatrogenic or pathological damage, the tongue, on protrusion, will deviate towards the unaffected side and there may be wasting of the affected side.
Special sensory innervation of the tongue
Dorsal lingual mucosa
Lingual papillae
Lingual papillae are projections of the mucosa covering the dorsal surface of the tongue (Fig. 30.5). They are limited to the presulcal part of the tongue, produce its characteristic roughness and increase the area of contact between the tongue and the contents of the mouth. There are four principal types, named filiform, fungiform, foliate and circumvallate papillae, and all except the filiform papillae bear taste buds. Papillae are more visible in the living when the tongue is dry.
Filiform papillae
Filiform papillae are minute, conical or cylindrical projections which cover most of the presulcal dorsal area, and are arranged in diagonal rows that extend anterolaterally, parallel with the sulcus terminalis, except at the lingual apex, where they are transverse. They have irregular cores of connective tissue and their epithelium, which is keratinized, may split into whitish fine secondary processes (Fig. 30.11). They appear to function to increase the friction between the tongue and food, and facilitate the movement of particles by the tongue within the oral cavity.
Fungiform papillae
Fungiform papillae occur mainly on the lingual margin but also irregularly on the dorsal surface, where they may occasionally be numerous (Fig. 30.11). They differ from filiform papillae because they are larger, rounded and deep red in colour, this last reflecting their thin, non-keratinized epithelium and highly vascular connective tissue core. Each usually bears one or more taste buds on its apical surface.
Circumvallate papillae
Circumvallate papillae are large cylindrical structures, varying in number from 8 to 12, which form a V-shaped row immediately in front of the sulcus terminalis on the dorsal surface of the tongue. Each papilla, 1–2 mm in diameter, is surrounded by a slight circular mucosal elevation (vallum or wall) which is separated from the papilla by a circular sulcus (Figs 30.12, 30.13). The papilla is narrower at its base than its apex and the entire structure is generally covered with non-keratinized stratified squamous epithelium. Numerous taste buds are scattered in both walls of the sulcus, and small serous glands (of von Ebner) open into the sulcal base.
Taste buds
Taste discrimination
Gustatory receptors detect four main categories of taste sensation, classified as salty, sweet, sour and bitter; other taste qualities have been suggested, including metallic and umami (Japanese: taste typified by monosodium glutamate). Although it is commonly stated that particular areas of the tongue are specialized to detect these different tastes, evidence indicates that all areas of the tongue are responsive to all taste stimuli. Each afferent nerve fibre is connected to widely separated taste buds and may respond to several different chemical stimuli. Some respond to all four classic categories, others to fewer or only one. Within a particular class of tastes, receptors are also differentially sensitive to a wide range of similar chemicals. Moreover, taste buds alone are able to detect only a rather restricted range of chemical substances in aqueous solution. It is difficult to separate the perceptions of taste and smell, because the oral and nasal cavities are continuous. Indeed, much of what is perceived as taste is the result of airborne odorants from the oral cavity which pass through the nasopharynx to the olfactory area above it.
TEETH
INTRODUCTION AND TERMINOLOGY
The terminology used to indicate tooth surfaces is shown in Fig. 30.14B. The aspect of teeth adjacent to the lips or cheeks is termed labial or buccal, that adjacent to the tongue being lingual (or palatal in the maxilla). Labial and lingual surfaces of an incisor meet medially at a mesial surface and laterally at a distal surface, terms which are also used to describe the equivalent surfaces of premolar and molar (postcanine) teeth. On account of the curvature of the dental arch, mesial surfaces of postcanine teeth are directed anteriorly and distal surfaces are directed posteriorly. Thus, the point of contact between the central incisors is the datum point for mesial and distal. The biting or occlusal surfaces of postcanine teeth are tuberculated by cusps which are separated by fissures forming a pattern characteristic of each tooth. The biting surface of an incisor is the incisal edge.
TOOTH MORPHOLOGY
There are two incisors, a central and a lateral, in each half jaw or quadrant (Figs 30.14, 30.15). In labial view, the crowns are trapezoid, the maxillary incisors (particularly the central) are larger than the mandibular. The biting or incisal edges initially have three tubercles or mamelons, which are rapidly removed by wear. In mesial or distal view, their labial profiles are convex while their lingual surfaces are concavo-convex (the convexity near the cervical margin is caused by a low ridge or cingulum, which is prominent only on upper incisors). The roots of incisors are single and rounded in maxillary teeth, but flattened mesiodistally in mandibular teeth. The upper lateral incisor may be congenitally absent or may have a reduced form (peg-shaped lateral incisor).
Behind each lateral incisor is a canine tooth with a single cusp (hence the American term cuspid) instead of an incisal edge. The maxillary canine is stouter and more pointed than the mandibular canine whose cusp tip is inclined lingually. The canine root, which is the longest of any tooth, produces a bulge (canine eminence) on the alveolar bone externally, particularly in the upper jaw. Although canines usually have single roots, those of the lower jaw may sometimes be bifid.
Deciduous teeth
The incisors, canine and premolars of the permanent dentition replace two deciduous incisors, a deciduous canine and two deciduous molars in each jaw quadrant (Figs 30.16, 30.17). The deciduous incisors and canine are shaped like their successors but are smaller and whiter and become extremely worn in older children. The deciduous second molars resemble the first permanent molars rather than their successors, the premolars. The upper first deciduous molar has a triangular occlusal surface (its rounded ‘apex’ is palatal) and a fissure separates a double buccal cusp from the palatal cusp. The lower first deciduous molar is long and narrow; its two buccal cusps are separated from its two lingual cusps by a zigzagging mesiodistal fissure. Both deciduous molars have large buccal protuberances on their mesial aspect. Upper deciduous molars have three roots (fusion of the palatal and distobuccal root is commonplace), and lower deciduous molars have two roots. These roots diverge more than those of permanent teeth because each developing premolar tooth crown is accommodated directly under the crown of its deciduous predecessor. The roots of deciduous teeth are progressively resorbed by osteoclast-like cells (odontoclasts) prior to being shed.
Eruption of teeth
Information on the sequence of development and eruption of teeth into the oral cavity (Fig. 30.18) is important in clinical practice and also in forensic medicine and archaeology. The tabulated data provided in Table 30.2 are largely based on European-derived populations and there is evidence of ethnic variation. When a permanent tooth erupts, about two-thirds of the root is formed and it takes about another 3 years for the root to be completed. For deciduous teeth, root completion is more rapid (Table 30.1). The developmental stages of initial calcification and crown completion are less affected by environmental influences than is eruption, the timing of which may be modified by several factors such as early tooth loss and severe malnutrition.
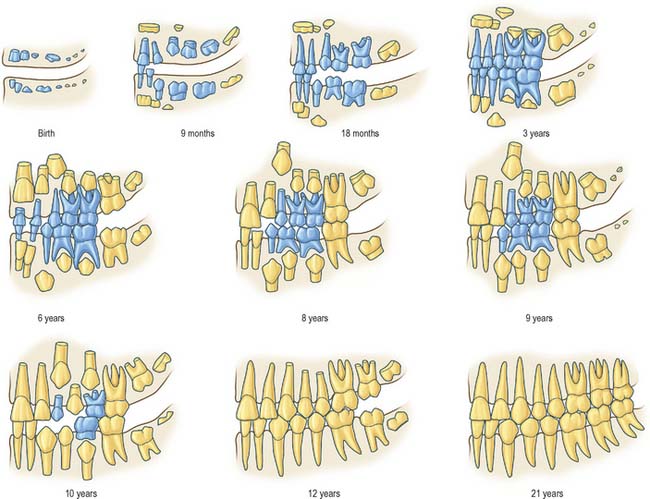
Fig. 30.18 Development of the deciduous (blue) and permanent (yellow) teeth.
(Modified with permission from Schour I, Massler M 1941 The development of the human dentition. J Am Dent Assoc 28: 1153–1160.)
Fig. 30.19 shows the panoramic appearance of the dentition seen with orthopantomograms at the time of birth, 2½, 6½, 10 and 16 years of age.
Dental alignment and occlusion
It is possible to bring the jaws together so that the teeth meet or occlude in many positions. When opposing occlusal surfaces meet with maximal ‘intercuspation’ (i.e. maximum contact), the teeth are said to be in centric occlusion. In this position the lower teeth are normally opposed symmetrically and lingually with respect to the upper. Some important features of centric occlusion in a normal (idealized) dentition may be noted. Each lower postcanine tooth is slightly in front of its upper equivalent and the lower canine occludes in front of the upper. Buccal cusps of the lower postcanine teeth lie between the buccal and palatal cusps of the upper teeth. Thus, the lower postcanine teeth are slightly lingual and mesial to their upper equivalents. Lower incisors bite against the palatal surfaces of upper incisors, the latter normally obscuring about one-third of the crowns of the lower. This vertical overlap of incisors in centric occlusion is the overbite. The extent to which upper incisors are anterior to lowers is termed the overjet. In the most habitual jaw position, the resting posture, the teeth are slightly apart, the gap between them being the freeway space or interocclusal clearance. During mastication, especially with lateral jaw movements, the food is comminuted, which facilitates the early stages of digestion.
General arrangement of dental tissues
A tooth consists of a crown covered by very hard translucent enamel and a root covered by yellowish bone-like cementum (Fig. 30.20A). These meet at the neck or cervical margin. A longitudinal ground section (Fig. 30.20B) reveals that the body of a tooth is mostly dentine (ivory) with an enamel covering up to about 2 mm thick, while the cementum is much thinner. The dentine surrounds a central pulp cavity, expanded at its coronal end into a pulp chamber and narrowed in the root as a pulp canal, opening at or near its tip by an apical foramen, occasionally multiple. The pulp is a connective tissue, continuous with the periodontal ligament via the apical foramen. It contains vessels for the support of the dentine and sensory nerves.
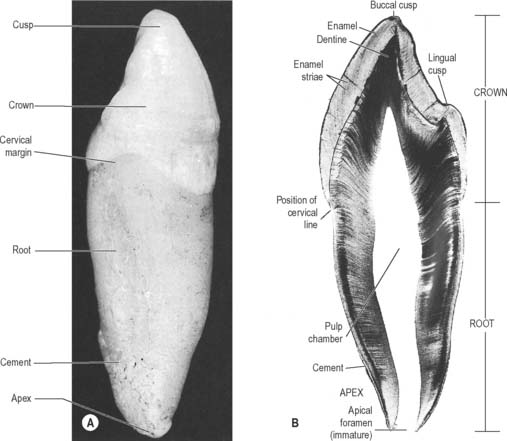
Fig. 30.20 The principal parts of a tooth. A, An extracted upper right canine tooth viewed from its mesial aspect. The root is covered by cement (partially removed), and the curved cervical margin is convex towards the cusp of the tooth. B, A ground section of a young (permanent) lower first premolar tooth sectioned in the buccolingual longitudinal plane, photographed with transmitted light. The enamel striae are incremental lines of enamel growth (compare with Fig. 30.24). Within the dentine the lines of the dentinal tubules are visible, forming S-shaped curves in the apical region but straighter in the root.
The root is surrounded by alveolar bone, its cementum separated from the osseous socket (alveolus) by the connective tissue of the periodontal ligament, approximately 0.2 mm thick (Fig. 30.21). Coarse bundles of collagen fibres, embedded at one end in cementum, cross the periodontal ligament to enter the osseous alveolar wall, these bundles of collagen being termed Sharpey’s fibres. Near the cervical margin, the tooth, periodontal ligament and adjacent bone are covered by the gingiva. On its internal surface the gingiva is attached to the tooth surface by the junctional epithelium, a zone of profound clinical importance because just above it is a slight recess, the gingival sulcus. As the sulcus is not necessarily self-cleansing, dental plaque may accumulate in it and this predisposes to periodontal disease.
Enamel
Enamel is an extremely hard and rigid material which covers the crowns of teeth. It is a heavily mineralized epithelial cell secretion, containing 95–96% by weight crystalline apatites (88% by volume) and less than 1% organic matrix. The organic matrix comprises mainly unique enamel proteins, amelogenins and non-amelogenins such as enamelins, tuftelins. Although comprising a very small percentage of the weight and volume of enamel, the organic matrix permeates the whole of enamel. As its formative cells are lost from the surface during tooth eruption, enamel is incapable of further growth. Repair is limited to the remineralization of minute carious lesions.
Enamel reaches a maximum thickness of 2.5 mm over cusps and thins at the cervical margins. It is composed of closely packed enamel prisms or rods. In longitudinal section, enamel prisms extend from close to the enamel–dentine junction to within 20 μm of the surface, where they are generally replaced by prismless (non-prismatic, aprismatic) enamel (Fig. 30.22A). In cross-section the prisms are mainly horseshoe-shaped and are arranged in rows that are staggered such that the tails of the prisms in one row lie between the heads of the prism in the row above (prism pattern 3) (Fig. 30.22B) and the tails are directed rootwards. The appearance of prism boundaries results from sudden changes in crystallite orientation. Prisms have a diameter of approximately 5 μm, and are packed with flattened hexagonal hydroxyapatite crystals, far larger than those found in collagen-based mineralized tissues.
Two types of incremental lines are visible in enamel, short-term and long-term. At intervals that average 4 μm – with a range of 2–3 μm at the enamel dentine junction and 4–6 μm in outer enamel – along its length, each prism is crossed by a line, probably reflecting diurnal swelling and shrinking in diameter during its growth. This short-term daily growth line is known as a cross-striation (Fig. 30.22C). The longer-term incremental lines pass from the enamel–dentine junction obliquely to the surface, where they end in shallow furrows, perikymata, visible on newly erupted teeth (Fig. 30.22D). Each line, known as an enamel stria of Retzius, represents a period that ranges between 6 and 12 days of enamel growth with a modal value of 9 days. This is known as an individual’s periodicity and it is constant in all teeth from the same individual but varies considerably between individuals (Reid & Dean 2006) (Fig. 30.22E). A prominent striation, the neonatal line, is formed in teeth whose mineralization spans birth. Neonatal lines are present in the enamel and dentine of teeth mineralizing at the time of birth (all the deciduous teeth and the first permanent molars; see Fig. 30.14) and are therefore of forensic importance, indicating that an infant has survived for a few days after birth. They reflect a disturbance in mineralization during the first few days after birth. Other accentuated lines can be observed throughout enamel growth; these are generally reflections in disturbances in ameloblast secretion caused by illness or nutritional deficiencies. In serious cases these disturbances will manifest as hypoplasia (enamel thinning on the tooth surface).
Dentine
Dentine is a yellowish avascular tissue which forms the bulk of a tooth. It is a tough and compliant composite material, with a mineral content of 70% dry weight (largely crystalline hydroxyapatite with some calcium carbonate) and 20% organic matrix (type I collagen, glycosaminoglycans and phosphoproteins). Its conspicuous feature is the regular pattern of microscopic dentinal tubules, 3 μm in diameter, which extend from the pulpal surface to the enamel–dentine junction. The tubules show lateral and terminal branching near the enamel–dentine junction (Fig. 30.23A) and may project a short distance into the enamel (enamel spindles). Each tubule encloses a single cytoplasmic process of an odontoblast whose cell body lies in a pseudostratified layer which lines the pulpal surface. Processes are believed to extend the full thickness of dentine in newly erupted teeth, but in older teeth they may be partly withdrawn and occupy only the pulpal third, while the outer regions contain probably only extracellular fluid. The diameter of the dentine tubule is narrowed by deposition of peritubular dentine. This is different from normal dentine (intertubular dentine) because it is more highly mineralized and lacks a collagenous matrix. Peritubular dentine can therefore be identified by microradiography (Fig. 30.23B). In time, it may completely fill the tubule, a process which gives rise to translucent dentine and which commences in the apical region of the root.
Dentine is formed slowly throughout life, and so there is always an unmineralized zone of predentine at the surface of the mineralized dentine, adjacent to the odontoblast layer at the periphery of the pulp. Biochemical changes within the mineralizing matrix mean that preentine stains differently to the matrix of the mineralized dentine. The predentine–dentine border is generally scalloped, because dentine mineralizes both linearly and as microscopic spherical aggregates of crystals (calcospherites). Dentine, like enamel, is deposited incrementally, and exhibits both short- and long-term incremental lines. Long-term lines are known as Andresen lines and in the mid-axial region are approximately 20 μm apart: they represent increments of about 6–12 days (Fig. 30.23C). Daily incremental lines (von Ebner lines) in the mid-axial plane are typically 4 μm apart; they are considerably closer together (1–2 μm apart) at the enamel–dentine junction and in root dentine. Where mineralization spans birth (i.e. all deciduous teeth and usually the first permanent molars) a neonatal line is formed in dentine similar to that which is seen in enamel, and it signals the abrupt change in both environment and nutrition which occurs at birth. As with enamel, disturbances in growth of dentine will exhibit as accentuated lines, commonly called contour lines of Owen.
Primary dentine formation proceeds at a steady but declining rate as first the crown and then the root is completed. A slow and intermittent deposition of dentine (regular secondary dentine) continues throughout life and further reduces the size of the pulp chamber; it is distinguished from primary dentine by the reduction and haphazard arrangement of dentinal tubules within it. The presence of the odontoblast process means that dentine is a vital tissue. It responds to adverse external stimuli – such as rapidly advancing caries, excessive wear or tooth breakage – by forming poorly mineralized dead tracts, in which the odontoblasts of the affected region die and the tubules remain empty (tertiary dentine). A dead tract may be sealed from the pulp by a thin zone of sclerosed dentine and the deposition of irregular (tertiary) dentine by newly differentiated pulp cells (Fig. 30.24).
Dental pulp
Dental pulp provides the nutritive support for the synthetic activity of the odontoblast layer. It is a well-vascularized, loose connective tissue, enclosed by dentine and continuous with the periodontal ligament via apical and accessory foramina. Several thin-walled arterioles enter by the apical foramen and run longitudinally within the pulp to an extensive subodontoblastic plexus. Blood flow rate per unit volume of tissue is greater in the pulp than in other oral tissues, and tissue fluid pressures within the pulp appear to be unusually high.
Dental pulp is extensively innervated by unmyelinated postganglionic vasoconstrictor sympathetic nerve fibres from the superior cervical ganglion, which enter with the arterioles, and by myelinated (Aδ) and unmyelinated (C) sensory nerve fibres from the trigeminal ganglion, which traverse the pulp longitudinally and ramify as a plexus (Raschkow’s plexus) beneath the odontoblast layer (Figs 30.25, 30.26). Here, any myelinated nerve fibres lose their myelin sheaths and continue into the odontoblast layer, and some enter the dentinal tubules, especially the region beneath the cusps. Stimulation of dentine, whether by thermal, mechanical or osmotic means, evokes a pain response. Pulp nerves release numerous neuropeptides.
Cementum
Like bone, cementum is perforated by Sharpey’s fibres, which represent the attachment bundles of collagen fibres in the periodontal ligament (extrinsic fibres). New layers of cementum, which are deposited incrementally throughout life to compensate for tooth movements, incorporate new Sharpey’s fibres. Incremental lines are irregularly spaced. The first cement to be formed is thin (up to 200 μm), acellular and contains only extrinsic fibres. Cementum formed later towards the root apex is produced more rapidly and contains cementocytes lying in lacunae joined by canaliculi. The latter are mainly directed towards their source of nutrients from the periodontal ligament. This cementum contains both extrinsic fibres derived from the periodontal ligament and intrinsic fibres of cementoblastic origin which lie parallel to the surface. Varying arrangements of layering between cellular and acellular cement occur. With increasing age, cellular cement may reach a thickness of a millimetre or more around the apices and at the branching of the roots, where it compensates for the loss of enamel by attrition. Cementum is not remodelled but small areas of resorption with evidence of repair may be seen.
Forensic anatomy of teeth
If teeth have been restored, extracted or replaced by a denture, an individual will have a virtually unique dentition which may have been recorded by the dentist in the form of charts, radiographs or plaster casts. Teeth are the most indestructible bodily structures and can provide identification when trauma or fire has rendered the face unrecognizable. Moreover, the chronology of crown development, eruption and root formation can be used to estimate age until the third molar is completed at about 21 years. The method is even applicable to the fetus because the weight of mineralized tissue in teeth is closely related to age from about 22 weeks’ gestation until birth. The time taken for a crown to form can be calculated from ground sections with considerable accuracy by counting the number of daily cross-striations from the neonatal line. For permanent teeth, the time taken for the crown to form can be calculated by counting the number of the enamel striae and multiplying by the individual’s periodicity. The age of adult teeth can be estimated from factors such as wear of the crown, reduction in size of the pulp and increase in thickness of cement in the apical half of the root. However, probably the most useful single characteristic is the amount of translucent dentine in the root, which is proportional to age. Such estimations are within 5–7 years of the chronological age and likely to be closer to the true age than those derived from skeletal changes.
Periodontal ligament
The majority of collagen fibres of the periodontal ligament are arranged as variously oriented dense fibre bundles that connect alveolar bone and cementum and which may help to resist movement in specific directions (Fig. 30.27). About 80% of the collagen in the periodontal ligament is type I, most of the remainder is type III. The rate of turnover of collagen is probably the highest of any site in the body, for reasons which are as yet unclear. A very small volume of fibres are oxytalan fibres.
Alveolar bone
That part of the maxilla or mandible which supports and protects the teeth is known as alveolar bone. An arbitrary boundary at the level of the root apices of the teeth separates the alveolar processes from the body of the mandible or the maxilla (Fig. 30.28). Like bone in other sites, alveolar bone functions as a mineralized supporting tissue, gives attachment to muscles, provides a framework for bone marrow and acts as a reservoir for ions, especially calcium. It is dependent on the presence of teeth for its development and maintenance, and requires functional stimuli to maintain bone mass. Where teeth are congenitally absent, as for example in anodontia, it is poorly developed, and it atrophies after tooth extraction, which can cause difficulties in the prosthodontic rehabilitation of patients.
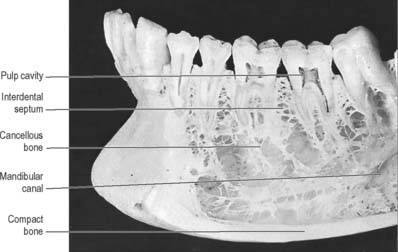
Fig. 30.28 Anterior part of the left side of the mandible, with superficial bone removed on the buccal side to show roots of a number of teeth, some of which have also been sectioned vertically. Note the cortical plate of compact bone lining the sockets of the teeth (the lamina dura of radiographs: see Fig. 30.30), and the flat table of bone surmounting the interdental bone septa. In this specimen the mandibular canal is widely separated from the roots of the teeth, a variable condition.
The alveolar tooth-bearing portion of the jaws consists of outer and inner alveolar plates. The individual sockets are separated by plates of bone termed the interdental septa, while the roots of multi-rooted teeth are divided by interradicular septa. The compact layer of bone which lines the tooth socket has been called either the cribriform plate, on account of its content of vascular (Volkmann’s) canals which pass from the alveolar bone into the periodontal ligament, or bundle bone, because numerous bundles of Sharpey’s fibres pass into it from the periodontal ligament (Fig. 30.29).
In clinical radiographs, the bone lining the alveolus commonly appears as a continuous dense white line about 0.5–1 mm thick, the lamina dura (Fig. 30.30). However, this appearance gives a misleading impression of the density of alveolar bone: the X-ray beam passes tangentially through the socket wall, and so the radiopacity of the lamina dura is an indication of the quantity of bone the beam has passed through, rather than the degree of mineralization of the bone. Superimposition also obscures the Volkmann’s canals. Chronic infections of the dental pulp spread into the periodontal ligament, which leads to resorption of the lamina dura around the root apex. The presence of a continuous lamina dura around the apex of a tooth therefore usually indicates a healthy apical region (except in acute infections where resorption of bone has not yet begun).
VASCULAR SUPPLY AND LYMPHATIC DRAINAGE OF THE TEETH AND SUPPORTING STRUCTURES
INNERVATION OF THE TEETH
The regional innervation of the teeth and gingivae is shown in Table 30.2. The teeth in the upper jaw are supplied by the superior alveolar nerves, while those in the lower jaw are supplied by the inferior alveolar nerve.
Inferior alveolar (dental) nerve
The course of the inferior alveolar nerve in the infratemporal fossa is described in Chapter 31. Just before entering the mandibular canal the inferior alveolar nerve gives off a small mylohyoid branch which pierces the sphenomandibular ligament and enters a shallow groove on the medial surface of the mandible, following a course roughly parallel to its parent nerve. It passes below the origin of mylohyoid to lie on the superficial surface of the muscle, between it and the anterior belly of digastric, both of which it supplies. It gives a few filaments to supply the skin over the point of the chin.
Local analgesia
In the case of the mandible, the anterior teeth can be anaesthetized by simple diffusion techniques as the bone is relatively thin. However, this is not adequate for the cheek teeth due to the increased thickness of the bone. In this case, the inferior alveolar nerve has to be anaesthetized before it enters the inferior alveolar canal. The needle has to be placed within the pterygomandibular space to achieve a successful inferior alveolar nerve block. The lingual nerve is also usually blocked, as it lies close to the inferior alveolar nerve. Because of the other structures within the infratemporal fossa, it is vitally important that the operator has a detailed knowledge of the anatomy in this region to understand, and therefore try to avoid, the complications that may arise. Any damage to blood vessels in the infratemporal fossa, generally the pterygoid venous plexus, can lead to haematoma formation. In extreme cases, bleeding can track through the inferior orbital fissure resulting in a retrobulbar haematoma, which can result in loss of visual acuity or blindness. Intravascular injection of local anaesthetic solution (which usually contains adrenaline [epinephrine]) can have profound systemic effects and for this reason an aspirating syringe is always used to check that the needle has not entered a vessel prior to injection (vessels in this area that theoretically may be entered include the maxillary and internal carotid arteries). If the needle is placed too medially, it may enter medial pterygoid, while if directed too laterally, it may penetrate temporalis. In either case, there will be lack of anaesthesia followed later by trismus. If the needle is placed too deeply, anaesthetic solution may cause a temporary facial nerve palsy due to loss of conduction from the facial nerve in the region of the parotid gland.
SALIVARY GLANDS
Salivary glands are compound, tubuloacinar exocrine glands whose ducts open into the oral cavity. They secrete saliva, a fluid which lubricates food to assist deglutition, moistens the buccal mucosa, which is important for speech, and provides an aqueous solvent necessary for taste and a fluid seal for sucking and suckling. They also secrete digestive enzymes, e.g. salivary amylase, and antimicrobial agents, e.g. immunoglobulin A (IgA), lysozyme and lactoferrin, into saliva. Conditions where there is a significant decrease in the production of saliva (xerostomia) may result in periodontal inflammation and dental caries. An illustration of the position of the major salivary glands and their ducts is shown in Fig. 30.31.
PAROTID GLAND
The parotid gland is the largest salivary gland. It is almost entirely serous. The parotid duct runs through the cheek and drains into the mouth opposite the maxillary second permanent molar tooth. The parotid gland is situated in front of the external ear and is described in detail in relation to the face (see Ch. 29).
SUBMANDIBULAR SALIVARY GLAND
Superficial part of the submandibular gland
The medial surface is related anteriorly to mylohyoid, from which it is separated by the mylohyoid nerve and vessels and branches of the submental vessels. More posteriorly, it is related to styloglossus, the stylohyoid ligament and the glossopharyngeal nerve, which separate it from the pharynx. In its intermediate part the medial surface is related to hyoglossus, from which it is separated by styloglossus, the lingual nerve, submandibular ganglion, hypoglossal nerve and deep lingual vein (sequentially from above down). Below, the medial surface is related to the stylohyoid muscle and the posterior belly of digastric.
Deep part of the submandibular gland
The deep part of the gland extends forwards to the posterior end of the sublingual gland. It lies between mylohyoid inferolaterally, hyoglossus and styloglossus medially, the lingual nerve superiorly, and the hypoglossal nerve and deep lingual vein inferiorly (Fig. 30.10).
Submandibular duct
The submandibular duct is usually 5 cm long and has a thinner wall than the parotid duct. It begins from numerous tributaries in the superficial part of the gland and emerges from the medial surface of this part of the gland behind the posterior border of mylohyoid. It traverses the deep part of the gland, and then passes at first up and slightly back for approximately 5 mm, this sharp bend over the free edge of mylohyoid being known as the genu of the duct. It then runs forwards between mylohyoid and hyoglossus passing between the sublingual gland and genioglossus to open in the floor of the mouth on the summit of the sublingual papilla at the side of the frenulum of the tongue (Figs 30.4, 30.10). It lies between the lingual and hypoglossal nerves on hyoglossus, but, at the anterior border of the muscle, it is crossed laterally by the lingual nerve, terminal branches of which ascend on its medial side. As the duct traverses the deep part of the gland it receives small tributaries draining this part of the gland. It has been suggested previously that the genu of the duct predisposes to the stasis of saliva and thereby encourages salivary stone (sialolith) formation, but this is somewhat controversial and largely unproven.
Like the parotid gland, the duct system of the submandibular gland can be visualized by sialography (Fig. 30.32).
SUBLINGUAL SALIVARY GLAND
The sublingual gland is the smallest of the main salivary glands: each gland is narrow, flat, shaped like an almond, and weighs approximately 4 g. The sublingual gland lies on mylohyoid, and is covered by the mucosa of the floor of the mouth, which is raised as a sublingual fold (Fig. 30.4). The anterior end of the contralateral sublingual gland lies in front, and the deep part of the submandibular gland lies behind. The mandible above the anterior part of the mylohyoid line, the sublingual fossa, is lateral, and genioglossus is medial, separated from the gland by the lingual nerve and submandibular duct.
The sublingual glands are seromucous, but predominantly mucous.
Sublingual ducts
The sublingual gland has 8–20 excretory ducts (Fig. 30.10). Smaller sublingual ducts open, usually separately, from the posterior part of the gland onto the summit of the sublingual fold (a few sometimes open into the submandibular duct). Small rami from the anterior part of the gland sometimes form a major sublingual duct (Bartholin’s duct), which opens with, or near to, the orifice of the submandibular duct. This duct may be visualized occasionally in a submandibular sialogram (Fig. 30.32).
Ranula
A ranula usually presents as a large tense bluish swelling anteriorly in the floor of the mouth just to one side of the midline, which often displaces the tongue. Occasionally the developing retention cyst herniates through a midline dehiscence where the two mylohyoid muscles fail to meet in the midline anteriorly, and then the ranula may present as a submental swelling or as a combined submental and floor of mouth swelling, a ‘plunging’ ranula. The treatment of a ranula is excision of the sublingual gland responsible.
MICROSTRUCTURE
Salivary glands have numerous lobes composed of smaller lobules separated by dense connective tissue which is continuous with the capsule of the gland, and contains excretory (collecting) ducts, blood vessels, lymph vessels, nerve fibres and small ganglia. Each lobule has a single duct, whose branches terminate at dilated secretory ‘endpieces’, which are tubular or acinar in shape (Fig. 30.33). Their primary secretion is modified as it flows through intercalated, striated and excretory ducts into one or more main ducts which discharge saliva into the oral cavity. They contain a variable amount of intralobular adipose tissue: adipocytes are particularly numerous in the parotid gland.
The secretory endpieces of the human parotid gland are almost exclusively serous acini (Fig. 30.34A): mucous tubules or acini are rare. In the submandibular gland, secretory units are predominantly serous acini, with some mucous tubules and acini (Fig. 30.34B). Mucous tubules are often associated with groups of serous cells at their blind ends, appearing as crescent-shaped serous demilunes in routine histological preparations. However, this appears to be a fixation artefact, as tissue prepared by rapid freezing methods lacks serous demilunes and the serous secretory cells align with mucous cells around a common lumen. In the sublingual gland, mucous tubules and acini predominate (Fig. 30.34C), but serous cells also occur, as acini or as serous demilunes.
Ducts
Intercalated, striated (both intralobular) and extralobular collecting ducts lead consecutively from the secretory endpieces. The lining cells of intercalated ducts are flat nearest the secretory endpiece, but become cuboidal. Intercalated ducts function primarily as a conduit for saliva but, together with the striated ducts, may also modify its content of electrolytes and secrete IgA. Striated ducts are lined by a low columnar epithelium and are so called because their lining cells have characteristic basal striations. The latter are regions of highly infolded basal plasma membrane, between which lie columns of vertically aligned mitochondria. The nuclei are consequently displaced by the basal striations from a typical ductal basal position to a central or even apical location (Fig. 30.34). Infolding of the basal plasma membrane, and local abundance of mitochondria, are typical features of epithelial cells which actively transport electrolytes. Here, the cells transport potassium and bicarbonate into saliva: they produce a hypotonic saliva by reabsorbing sodium and chloride ions in excess of water. Striated ducts modify electrolyte composition and secrete IgA, lysozyme and kallikrein. IgA is produced by subepithelial plasma cells and transported transcytotically across the epithelial barrier to be secreted, once it has been dimerized by epithelial secretory component, into the saliva. This is also a function of serous acinar cells and other secretory epithelia, notably the lactating breast (see Ch. 54). The intralobular ductal system of the sublingual gland is less well developed than that of the parotid and submandibular glands.
Myoepithelial cells
Myoepithelial cells (Fig. 30.33) are contractile cells (see Ch. 2) associated with secretory endpieces and with much of the ductal system. They lie between the basal lamina and the epithelial cells proper. They extend numerous cytoplasmic processes around serous acini and are often termed basket cells. Myoepithelial cells associated with ducts are more fusiform in shape, and are aligned along the length of the duct. Their cytoplasm contains abundant actin microfilaments which mediate contraction under the control of both sympathetic and parasympathetic stimulation. The outflow of saliva is thus accelerated through reduction in the luminal volume of secretory endpieces and ducts, contributing to the secretory pressure.
CONTROL OF SALIVARY GLAND ACTIVITY
The typical pattern of innervation is shown in Fig. 30.33, but details vary in different glands, and with age. Only the more constant features are illustrated and described here. Cholinergic nerves often accompany ducts and arborize freely around secretory endpieces, but adrenergic nerves usually enter glands along arteries and ramify with them. The main secretomotor nerves are predominantly non-myelinated axons: the few myelinated axons that have been seen are presumably either preganglionic efferent or visceral afferent. Within the glands the nerve fibres intermingle, such that cholinergic and adrenergic axons often lie in adjacent invaginations of one Schwann cell. Secretion and vasoconstriction are mediated via separate sympathetic axons. A single parasympathetic axon may, through serial en passant terminals, induce vasodilatation, secretion and myoepithelial contraction.
TISSUE SPACES AROUND THE JAWS
The dissemination of infection in soft tissues is influenced by the natural barriers presented by bone, muscle and fascia. However, the tissue spaces around the jaws are primarily defined by muscles, principally mylohyoid, buccinator, masseter, medial pterygoid, superior constrictor and orbicularis oris (Fig. 30.35). None of these ‘spaces’ is actually empty and they should merely be regarded as potential spaces that are normally occupied by loose connective tissue. It is only when inflammatory products such as hyaluronase destroy the loose connective tissue that a definable space is produced.
The spaces are paired except for the submental, sublingual and palatal spaces.
POTENTIAL TISSUE SPACES AROUND THE LOWER JAW
The important potential tissue spaces of the lower jaw are the submental, submandibular, sublingual, buccal, submasseteric, parotid, pterygomandibular, peritonsillar, parapharyngeal and retropharyngeal spaces (Fig. 30.35).
The remaining tissue spaces are illustrated in Fig. 30.35B. The buccal space is located in the cheek, on the lateral side of buccinator. The submasseteric spaces are a series of spaces between the lateral surface of the ramus of the mandible and masseter: they are formed because the fibres of masseter have multiple insertions onto most of the lateral surface of the ramus. The pterygomandibular space lies between the medial surface of the ramus of the mandible and medial pterygoid, and the parotid space lies behind the ramus of the mandible, in and around the parotid gland. The parapharyngeal space is bounded by the superior constrictor of the pharynx and the medial surface of medial pterygoid. It is restricted to the infratemporal region of the head and the suprahyoid region of the neck and communicates with the retropharyngeal space, which itself extends into the retrovisceral space in the lower part of the neck (the tissue spaces of the neck are described in Chapter 28, and of the pharynx in Chapter 33). The peritonsillar space lies around the palatine tonsil between the pillars of the fauces, and is part of the intrapharyngeal space. It is bounded by the medial surface of the superior constrictor of the pharynx and its mucosa.