CHAPTER 126 On-the-field Assessment of the Cervical Spine-Injured Athlete
INTRODUCTION
Physicians caring for athletes during sports competition must be prepared to evaluate and treat injuries to the cervical spine. While most injuries are mild and self-limiting, injury to the spinal cord resulting in temporary or permanent neurologic injury is a rare but potentially catastrophic event. The medical team at sporting events must be prepared to assess, stabilize, and transport athletes with suspected cervical spine injuries.
Cervical spine trauma is most common in contact or collision sports such as American football, rugby, and ice hockey.1–6 Other sports such as gymnastics, skiing, wrestling, and diving also carry a higher risk of injury.1,7,8 American football has provided the largest population of athletes to follow with serious cervical spine injuries. The National Center for Catastrophic Sports Injury Research reported a total of 223 spinal cord injuries in American football between 1977 and 2001, with an incidence of cervical spinal cord injury in high school football of 0.52/100 000 participants, 1.55/100 000 participants in college football, and 14/100 000 participants in professional football.9 Cervical spinal cord injuries were also the most common catastrophic football injury and the second leading cause of death attributable to football.9
A NOTE ON ATHLETIC BRAIN INJURY AND CONCUSSION
Concussion is the most common sports-related head injury and is defined as a transient post-traumatic alteration in neural functioning. Concussive symptoms include confusion, dizziness, headache, visual disturbance, nausea, equilibrium disturbance, difficulty concentrating, post-traumatic amnesia, and loss of consciousness. Many classifications of concussion have attempted to grade the severity of concussion based on clinical presentation and duration of symptoms.10,11 Recent research involving neuropsychological testing pre- and post-concussion has better delineated which symptoms correlate with severity and the time needed for full recovery.12 Collins et al.12 showed that the presence of amnesia, not loss of consciousness, is the best predictor of concussion severity and neurocognitive deficits. For the sideline evaluation, no athlete with a concussion should be allowed to return to play if still symptomatic. Several references are available which summarize recent advances and recommendations in the management of athletic concussion.13–15
MECHANISM OF INJURY
Axial loading
The mechanism of injury to the cervical spine and associated anatomic risk factors that lead to serious neurologic injury have been the subject of active interest and debate within the sports medicine and spine surgery communities. Early research suggested that serious cervical spine injuries occurred from forced hyperflexion2–7,16 or hyperextension.17–20 However, more recently axial loading has been defined as the most likely mechanism for catastrophic injury to the cervical spine during sports competition.
Axial loading occurs when a player strikes another player with the top of the head as the point of initial contact (so-called ‘spear tackling’). Axial loading places the cervical spine at a biomechanical disadvantage and makes it particularly susceptible to severe injury. Typically, most forces from a traumatic load to the cervical spine are dissipated through controlled spinal motion, the paravertebral muscles, and the intervertebral discs. When the top or crown of the helmet is used for initial contact, the neck is typically flexed approximately 30° and loses its normal cervical lordosis and ability to properly distribute force (Fig. 126.1). In this position the cervical spine assumes the characteristics of a segmented column and forces are transmitted along the longitudinal axis of the spine (Fig. 126.2).21
In athletes with cervical spinal stenosis, axial loading followed by forced hyperextension or hyperflexion can cause transient or permanent sensory and motor changes. Penning19 described a ‘pincer mechanism’ with compression of the spinal cord between a vertebral body and the spinolaminar line of an adjacent vertebra. Compression of the spinal cord occurs in hyperextension when the distance between the posteroinferior margin of the superior vertebral body and the anterosuperior aspect of the spinolaminar line of the subjacent vertebra decreases (Fig. 126.3). With hyperflexion, the anterosuperior aspect of the spinolaminar line of the superior vertebra and the posterosuperior margin of the inferior vertebra approximate. Eismont et al.20 also noted that hyperextension causes narrowing of the anteroposterior diameter of the spinal canal and can compress the spinal cord. Torg et al.22 described an axial load input with subsequent hyperextension and indentation of the ligamentum flavum with compression of the spinal cord. In each of these mechanisms, the anteroposterior diameter of the spinal canal decreases, resulting in compression of the spinal cord. Transient or permanent cervical cord injury can occur and usually involves a contusion of the spinal cord with temporary restriction of blood flow and a focal area of ischemia.8
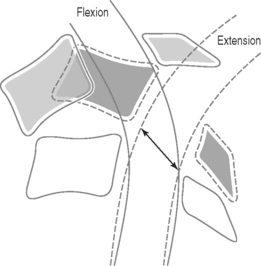
Fig. 126.3 Hyperextension ‘pincer mechanism’ as described by Penning.19 During hyperextension, the distance between the posteroinferior aspect of the superior vertebral body and the anterosuperior aspect of the spinal laminar line of the subjacent vertebra decreases, and the cord is pinched between the two vertebral bodies.
While advances in helmet materials and equipment used in American football lead to a decrease in head injuries, it also contributed to the development of dangerous playing techniques using the top of the helmet as the initial point of contact. Torg et al.23 compared the total number of head and neck injuries in the National Football League between 1971 and 1975 with previous work done by Schneider24 analyzing injuries between 1959 and 1963. The major difference between these time periods was the type and quality of helmet worn during competition. The study found a reduction in intracranial hemorrhages of 66% and deaths due to intracranial injury of 42%. However, the study also found a 204% increase in cervical spine fractures, subluxations, and dislocations and a 116% increase in the number of cases of permanent quadriplegia. The majority of cases of permanent quadriplegia that occurred were due to direct compression when a player struck another player with the top of his helmet.
Delineation of the axial load mechanism as the major cause of catastrophic cervical spine injury in American football resulted in rule changes that caused a sustained decrease in the rate of cervical spine injuries and quadriplegia. In 1976 the National Collegiate Athletic Association banned ‘spearing,’ defined as intentionally striking an opponent with the crown of the helmet, as well as other tackling techniques in which the helmet is used as the initial point of contact. In 1976, the rate of cervical spine fracture, subluxation, and dislocation was 7.72/100 000 and 30.66/100 000 in high school and college athletes, respectively. In 1987, this had decreased to 2.31/100 000 and 10.66/100 000 serious injuries to the cervical spine in high school and college athletes, respectively.21 Injuries resulting in permanent quadriplegia also significantly decreased as a result of the rule changes. In 1976, the rate of quadriplegia was 2.24/100 000 and 10.66/100 000 in high school and college athletes, respectively. In 1977, only 1 year after the rule changes, these rates decreased to 1.30/100 000 and 2.66/100 000 in high school and college athletes, respectively, and have continued to remain low.21
In 1990 Torg and colleagues25 further confirmed axial loading as the mechanism in catastrophic cervical spine injury by review of actual game films and videotapes. Analysis of films allowed an accurate determination of the mechanism of injury in 85% of cases, and axial loading was found to be the mechanism in every case.
INJURIES TO THE NECK AND SPINAL CORD
Stingers
‘Stingers’ or ‘burners’ are descriptive terms used to characterize transient unilateral upper extremity pain and paresthesias following a blow to the neck or shoulders. Because of confusion with a potentially more serious central cord injury called ‘burning hands syndrome,’26 use of the term ‘stinger’ rather than ‘burner’ is encouraged. Stingers are very common and are reported in up to 50–65% of college football players.27,28
Stingers usually result from one of two mechanisms: tensile overload or compression overload.29 While either mechanism is possible in any athlete, the mechanism of injury is more likely depending on the age of the athlete. In most high school athletes, the mechanism involves a tensile or stretch injury when the involved arm and neck are stretched in opposite directions. This occurs as the neck is forcibly stretched away while the ipsilateral shoulder is depressed (Fig. 126.4). In the older college or professional athlete with a higher likelihood of degenerative changes of the cervical spine, a compressive mechanism is more likely. This involves a compression or ‘pinch’ of the cervical nerve root within the neural foramen as the neck is forcibly flexed in a posterolateral direction towards the symptomatic upper extremity (see Fig. 126.4).30
Recurrent stingers are more common in the presence of cervical degenerative disc disease. Levitz et al.30 studied 55 athletes with recurrent stingers. The mechanism of injury was extension combined with ipsilateral compression in 83% of patients, and 70% had a positive Spurling’s sign. Eighty-seven percent of patients had evidence of disc disease by magnetic resonance imagimg (MRI), and 93% had disc disease or narrowing of the intervertebral foramina. The authors concluded that nerve root compression in the intervertebral foramina secondary to degenerative disc disease was the likely cause of recurrent or chronic stinger syndromes in collegiate and professional athletes. Athletes with more than one stinger can be placed in a protective neck collar that limits lateral neck flexion and hyperextension.
Rarely, more significant nerve injury and axonotmesis occurs causing chronic symptoms. Patients usually demonstrate persistent weakness in the distribution of the upper trunk of the brachial plexus, and muscle atrophy may be present in severe cases. Electromyography will assess the distribution and degree of injury and should be performed if symptoms have lasted more than 3 weeks. Most cases of axonotmesis have a high likelihood of recovering within 1 year.27
Cervical cord neurapraxia and transient quadriplegia
Injury to the spinal cord can be complete or be neurapraxic – a reversible injury with motor and sensory function returning within 24–48 hours. Neurapraxia of the cervical spinal cord is characterized by an acute, transient sensory and/or motor change to more than one extremity. Symptoms include burning pain, numbness, tingling and loss of sensation with or without motor changes of paresis or complete paralysis.31 Several other terms have been used to describe cervical cord neurapraxia and have been used interchangeably, including ‘transient quadriplegia,’31 ‘central cervical spinal cord syndrome,’32 and ‘spinal cord concussion.’33
The most typical pattern of incomplete spinal cord injury is the central cord syndrome. This involves weakness of the upper extremities in excess of lower extremity findings secondary to the lamination of the corticospinal tracts and selective damage to fibers serving arm and hand function. ‘Burning hands syndrome’ described by Maroon26 is characterized by burning dysesthesias of the hands and associated weakness of the upper extremities and is a variant of central cord syndrome. Transient quadriplegia is a temporary paralysis characterized by loss of motor function, with or without sensory disturbances.31
Episodes of cervical cord neurapraxia are transient by definition and complete recovery usually occurs in 10–15 minutes, although in some patients gradual resolution occurs over 1–2 days. Except for burning paresthesia, the athlete does not experience neck pain, and there is complete return of motor function and full, pain-free range of motion of the cervical spine. Torg et al.34 studied 110 patients with transient cervical cord neurapraxia and found that 40% presented with plegia (complete motor weakness), 25% with paresis (incomplete motor weakness), and 35% with only paresthesia and sensory symptoms. The neurapraxia resolved in 74% of patients within 15 minutes and lasted more than 24 hours in 11% of patients. Eighty percent of patients had symptoms in all 4 extremities, 15% in the upper extremities only, 2% in lower extremities only, and 3% with symptoms in the ipsilateral upper and lower extremities. Of 110 patients, 109 had a complete neurologic recovery, with one patient having a residual hemiplegia following operative intervention. For those athletes who did return to football, the recurrence rate for transient cervical cord neurapraxia was 56% with no athlete experiencing permanent neurologic injury, although the follow-up period was only 3 years and some athletes chose to retire from collision sports.
Cervical spinal stenosis
The method to best determine spinal stenosis in an athlete has been the subject of considerable debate. Early definitions used the absolute anteroposterior canal diameter to detect spinal stenosis – measured from the posterior aspect of the vertebral body to the most anterior point on the spinolaminar line. In general, a canal was considered normal if the diameter between C3 and C7 was >15 mm, and spinal stenosis was present if the diameter was <12 mm.35
Torg et al.31 and Pavlov et al.36 described a measurement to detect spinal stenosis using a spinal canal to vertebral body ratio (known as the ‘Torg ratio’). The ratio uses the anteroposterior diameter of the spinal canal and the anteroposterior width of the midpoint of the vertebral body (Fig. 126.5). The measurements are made at the level of the third through the sixth vertebral body on a routine lateral radiograph of the cervical spine. The ratio method eliminates error caused by magnification factors or differences in target-to-film distances. A spinal canal-vertebral body ratio of <0.8 indicates significant spinal stenosis. In Torg’s study,31 a ratio of <0.8 was recorded at one or more levels in 24 athletes with cervical cord neurapraxia, compared to a ratio of 1.0 or more in 49 age-matched controls with no neurologic complaints.
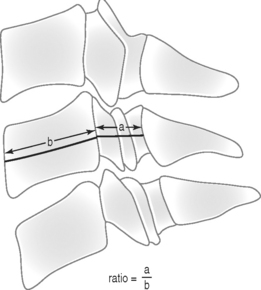
Fig. 126.5 The Torg ratio is the distance from the midpoint of the posterior aspect of the vertebral body to the nearest point on the spinolaminar line (a) divided by the anteroposterior width of the vertebral body (b).31
Eismont et al.20 also observed that a smaller-diameter spinal canal measured by plain film significantly correlated with neurologic injury in 98 patients with cervical spine traumatic fracture-dislocations. Others studies have further documented the association of a small sagittal diameter of the spinal canal with the severity of neurologic injury following cervical spine trauma.37,38
The Torg ratio has been the subject of considerable scrutiny, and its usefulness in sports and spine medicine questioned. Herzog et al.39 found the ratio to have a high sensitivity (92%) but a low positive predictive value (12%) for detecting cervical spinal stenosis in a cohort of professional football players. In their study, 49% of professional football players had a Torg ratio <0.8 at one or more levels, but only 13% had true spinal stenosis documented on advanced imaging. Herzog et al. concluded that the Torg ratio was a poor predictor of true functional spinal stenosis and may yield many false-positive results, and the low positive predictive value of the ratio precludes its use as a screening tool for determining eligibility of an athlete for participation in contact sports.
Several problems exist which limit the usefulness of the Torg ratio in determining spinal stenosis. Most concerning is that the ratio does not account for the size of the spinal cord and define the anatomic relationship of the spinal cord within the spinal canal. In addition, the ratio does not identify soft tissue pathology that may be impinging on the spinal canal. Resnick40 stated that roentgenography alone failed to assess the width of the cord and account for stenosis which results from ligamentous hypertrophy or disc protrusion. Cantu41 also argued that spinal stenosis cannot be defined by bony measurements alone and that normal canal size on a lateral radiograph does not rule out the possibility of ‘functional spinal stenosis.’ He defines ‘functional spinal stenosis’ as a loss of cerebrospinal fluid (CSF) around the cord or deformation of the spinal cord, as documented on computed tomography (CT), MRI, or myelography.42 The term ‘functional’ refers to the functional reserve or protective cushion of the CSF around the spinal cord.
While the Torg ratio has its limitations, Torg and colleagues31,36 should be credited with their efforts to define a measure used to assess cervical spinal stenosis and the risk of cervical cord injury. In addition, it should be noted that the Torg ratio was created at a time when MRI was not widely available. In the setting of an athlete with a history of cervical cord neurapraxia or transient quadriplegia, an evaluation with MRI, myelogram, or contrast-positive CT should be performed. If the Torg ratio is measured early in the diagnostic evaluation, an abnormal ratio is a strong indicator to pursue advanced imaging.41
Whether congenital or acquired, the relationship of cervical spinal stenosis to serious neurologic injury is well established. The National Center for Catastrophic Injury Research has reported cases of quadriplegia in the absence of a spine fracture only when functional spinal stenosis is present, and conversely reported cases of complete neurologic recovery after spine fracture or dislocation only in the absence of functional spinal stenosis.42 In addition, the presence of cervical spinal stenosis places the athlete at higher risk to require surgery after a cervical disc herniation, for potential paralysis without a fracture-dislocation, and to develop paralysis and a greater degree of paralysis after fracture-dislocation.20,42–46
Return to play after cervical cord neurapraxia
Return to play after an episode of cervical cord neurapraxia is a frequently discussed and controversial area in sports medicine and spine surgery. Torg et al.47 compared cohorts of asymptomatic college and professional football players with no history of transient neurapraxia, with a cohort of 45 high school, college, and professional athletes with an episode of transient neurapraxia, and 77 patients with permanent quadriplegia as a result of high school or college football. The Torg ratio was found to have a sensitivity of 93% for the detection of transient neurapraxia, but a low specificity and low positive predictive value. None of the 77 patients with permanent quadriplegia had an episode of transient neurapraxia before catastrophic injury to the spinal cord, and none of the 45 athletes with transient neurapraxia became quadriplegic. Developmental narrowing of the spinal canal in the quadriplegic group was also absent. The authors concluded that the occurrence of transient neurapraxia of the spinal cord and permanent quadriplegia were unrelated, and that developmental narrowing of the spinal canal in the absence of instability is not a predisposing factor for permanent neurologic injury. Therefore, congenital cervical spinal stenosis should not preclude an athlete from participation in contact sports.
Torg et al.34 further characterized the relationship of the spinal cord to the spinal canal by analyzing MR images in 110 athletes with cervical cord neurapraxia. The disc-level canal diameter was measured as the shortest distance between the intervertebral disc and the osseous posterior elements and the transverse cord diameter determined. The MRI measurements were compared to the spinal canal–vertebral body ratio measured on lateral radiograph. The study found using both methods of measurement that the probability of recurrent cervical cord neurapraxia was inversely correlated with spinal canal size. Using logistic regression analysis, plots were generated to calculate the risk of recurrent neurapraxia that could be used in counseling athletes with a history of cervical cord neurapraxia about their individual risk of a recurrent episode. The authors concluded that: (1) cervical cord neurapraxia is a transient neurological phenomenon and individuals with uncomplicated neurapraxia may be permitted to return to their previous sport without an increased risk of permanent neurological injury; (2) congenital or degenerative narrowing of the sagittal diameter of the cervical canal is a causative factor; (3) the overall recurrence rate after return to play is 56%; and (4) the risk of recurrence is strongly and inversely correlated with sagittal canal diameter and is useful in the prediction of future episodes of cervical cord neurapraxia. Although controversial, Torg et al.21 do not believe that athletes who experience an episode of uncomplicated transient cervical cord are at risk of incurring permanent neurologic sequelae and should not be disqualified from participation in contact or collision sports.
Other experts strongly disagree. Cantu41 contends that cervical spinal stenosis increases the risk of permanent neurologic injury and asserts that a history of transient quadriplegia and functional spinal stenosis is an absolute contraindication to return to contact or collision sports. Table 126.1 contrasts return to play recommendations following cervical cord neurapraxia by Torg et al.21 and Cantu.41
Table 126.1 Guidelines for Return to Play After Transient Cervical Cord Neurapraxia
Torg21 | Cantu41 | |
---|---|---|
No restriction | No history of CCN and spinal canal–vertebral body ratio ≥0.8 | One episode of TQ with full recovery and normal work-up without functional spinal stenosis |
Relative restriction |
CCN, cervical cord neurapraxia; TQ, transient quadriplegia.
Several reported cases draw support of Cantu’s concept of functional spinal stenosis and raise concern regarding Torg’s recommendations. Firooznia et al.43 reported three cases of permanent quadriplegia in patients with marked spinal stenosis after only minor trauma. Matsuura et al.38 also described the association of cervical canal stenosis and neurologic injury and found that the shape of the bony spinal canal as measured by thin-cut CT was critical in determining residual neurologic injury following traumatic spinal cord injury. In addition, Cantu41 reported a case of a high school football player with transient quadriplegia who had abnormal Torg ratios by lateral radiograph, but was allowed to return to play and later suffered a permanent spinal cord injury with resultant spastic quadriplegia. After the second injury, CT and MRI revealed functional cervical stenosis, a posterior disc herniation of C3–4 with displacement of the cord and thecal sac, and edema within the spinal cord from C2 to C5. Brigham and Adamson33 also reported a case of permanent neurologic injury in a professional football player with congenital spinal stenosis. This player presented with tingling in all four extremities upon neck flexion. Initial MRI demonstrated congenital cervical spinal stenosis without degenerative changes. Two years later he sustained an axial load to the neck and experienced immediate numbness in all four extremities, severe burning, and painful dysesthesias in both arms. MRI immediately after this injury was normal, but 3 months later repeat MRI demonstrated a focus of increased signal within the spinal cord at C5 likely representing an area of diffuse axonal injury. The patient continues to experience mild, bilateral upper extremity dysesthesias.
In athletes who experience transient cervical cord neurapraxia, the criteria for return to play are controversial and must be individualized. The reported cases of permanent neurologic injury following cervical cord neurapraxia in the setting of functional spinal stenosis are concerning.33,41 This author believes that the presence of functional cervical spinal stenosis in an athlete with a history of cervical cord neurapraxia is an absolute contraindication for the athlete to return to play in contact or collision sports. Mild disc bulging without functional spinal stenosis in an athlete with a history of transient cervical cord neurapraxia is a relative contraindication to return to play.
Spear-tackler’s spine
Torg and colleagues22 described a group of athletes who are at a particularly high risk for the development of cervical spinal cord injuries because of developmental narrowing of the cervical canal and the use of spear tackling or head-first playing techniques. In 15 patients who used spear-tackling techniques, permanent neurologic injury occurred in four patients from axial loading. Each of the four patients with permanent injuries had the following combination of findings on cervical spine radiographs: (1) developmental cervical canal stenosis (defined by a spinal canal–vertebral body ratio of <0.8); (2) straightening or reversal of cervical lordosis; and (3) preexisting minor post-traumatic evidence of bone or ligamentous injury. Some experts regard spear-tackler’s spine as an absolute contraindication to participation in collision sports.21 Other experts believe that return to collision sports is permitted if normal cervical lordosis is restored and the athlete refrains from further spear-tackling techniques.41
Fractures and dislocations
Axial loading to a straightened cervical spine results in compressive deformation and subsequent failure in a flexion mode with the potential for fracture, subluxation, or facet dislocation.25 The axial load ‘teardrop’ fracture is characterized by a triangular fracture fragment at the anteroinferior corner of the cervical vertebral body with a sagittal fracture through the vertebral body and the posterior arch (Fig. 126.6). These fractures occur most commonly at C5 but can also occur at C4 and C6. In a series of 55 patients with this injury from the National Football Head and Neck Injury Registry, 49 patients had a three-part, two-plane fracture including a sagittal fracture of the vertebral body, with resultant quadriplegia in 90% of patients.48 Isolated fracture of the anteroinferior corner occurred in 11% of cases and usually without neurologic sequelae.
Anterior subluxation and unilateral and bilateral facet dislocations are infrequent but severe injuries with a high risk of permanent quadriplegia. Torg et al.49 identified the C3–4 level as the most common dislocation in football resulting in quadriplegia. Anterior subluxation usually involves rupture of the posterior longitudinal ligament and ligamentum flavum. Prompt recognition and reduction in a controlled environment have led to more favorable results. Contrast-enhanced CT or MRI of the cervical spine should be obtained before fracture reduction to rule out the presence of a retropulsed interveterbral disc.41 Other potential injuries include a Jefferson fracture of the ring of C1, ‘Hangman’s fracture’ involving bilateral fractures of the pars interarticularis (Fig. 126.7), zygapophyseal (facet) fractures, and rupture of the atlantoaxial transverse ligament with atlantoaxial dislocation. Most fracture-dislocations of the cervical spine require surgical stabilization.
Some fractures such as isolated spinous process fractures require minimal treatment with short-term immobilization in a cervical collar. In general, stable fractures that have healed completely allow the athlete to return to play the following season. These fractures include chip, minor wedge, isolated laminar, and spinous process fractures. Athletes with cervical spine fractures or dislocations that require reduction and surgical stabilization are not considered safe to return to contact sports. Even after fracture healing, these athletes are thought to have insufficient spinal strength and altered biomechanics and motion in surrounding spinal segments and are at high risk of future sports-related injury.41,50 Thus, any injury requiring internal stabilization is suspect in its ability to withstand further stresses from contact or collision sports. Cervical fusions at the C4 level or above and multilevel fusions are also a contraindication to return to contact or collision sports.
ON-THE-FIELD EVALUATION
Adequate preparation is required for the assessment of the cervical spine-injured athlete. Anticipation of required personnel and equipment and a well-designed emergency response are critical to the management of catastrophic neck injuries. The medical team should develop and review emergency plans including predetermination of who will be on the field (i.e. certified athletic trainer and team physician), who will conduct the initial evaluation, what special equipment should be brought onto the field, and when the emergency medical system (EMS) should be contacted. Emergency equipment such as a spine board, cervical collar, and devices used to remove protective gear safely and quickly should be readily available. In 2001, the Inter-Association Task Force for Appropriate Care of the Spine-Injured Athlete established guidelines for the prehospital care of the spine-injured athlete.44 Experts from a range of disciplines including athletic training, sports medicine, rehabilitation medicine, orthopedic surgery, neurosurgery, and emergency medical technicians contributed to the recommendations, and the guidelines should be regarded as the standard of care in the evaluation of a spine-injured athlete.
Initial assessment
Once adequate ventilation and circulation are established, the level of consciousness should be further assessed. The conscious athlete is questioned regarding person, place, situation, the presence of neck pain, and sensory or motor disturbances in any body part. A screening neurologic examination is performed to assess sensation and strength in all four extremities. In the unconscious athlete or athlete with altered mental status, the Glasgow Coma Scale may be a useful prognostic indicator of cerebral function (Table 126.2).10
Response | Points | |
---|---|---|
Eyes open | Spontaneously | 4 |
To verbal commands | 3 | |
To pain | 2 | |
No response | 1 | |
Best motor response | Obeys commands | 6 |
Localizes pain | 5 | |
Withdrawal to pain | 4 | |
Flexion (decorticate) to pain | 3 | |
Extension (decerebrate) to pain | 2 | |
No response | 1 | |
Best verbal response | Oriented | 5 |
Confused | 4 | |
Inappropriate words | 3 | |
Incomprehensible sounds | 2 | |
No response | 1 |
The Glasgow Coma Scale is scored between 3 and 15 (3 being the worst and 15 the best). A score of 13 or higher correlates with mild brain injury; a score of 9 to 12 correlates with moderate brain injury; and a score of 8 or less represents severe brain injury.
Equipment management
Protective equipment used in sports such as football and hockey can make the management of a spine-injured athlete more difficult. Each member of the medical team should be familiar and practiced in the operation of emergency equipment. Practice with the tools required for face mask removal of the catastrophically injured athlete is essential. The face mask should be removed as soon as possible before transportation and regardless of respiratory status, even if the athlete is conscious.44 In football, the face mask is secured to the helmet with four or more plastic loop-straps that can be cut or removed. Several tools for removing the loop-straps have been described including screw drivers and various cutting tools such as knives, scissors, pruning shears, the Trainer’s Angel (Fig. 126.8), and the Face Mask Extractor.51–53 Because moisture can rust the screws making screwdrivers unreliable, and injuries to other rescuers have been documented while using cutting instruments such as knives, scalpels, or box cutters, these tools are not recommended as the primary tool for loop-strap removal.54
The most widely used tool specifically made to remove loop-straps is the Trainer’s Angel. However, this tool may cause more head movement than other available devices. The face mask removal should be performed in the shortest time possible with the least amount of head and neck movement. The anvil pruner, commonly used for gardening, has been shown repeatedly to be the most efficient tool for removal of the loop-straps and is recommended by the Inter-Association Task Force.44,54 Athletic trainers or another designated member of the responding medical team should have the appropriate removal equipment available and be familiar with its use before an emergency occurs.
Football helmets and chin straps should be left in place with few exceptions. If the athlete is wearing shoulder pads, football helmets hold the head in a position of neutral spinal alignment. If the helmet is removed from a down player wearing shoulder pads, the athlete’s head will hyperextend and may result in secondary injury to the cervical spine. The Inter-Association Task Force recommends removal of a helmet on the field under the following circumstances: (1) if the face mask cannot be removed in a reasonable period of time to gain access to the airway; (2) if after removal of the face mask the airway and ventilation cannot be adequately controlled; (3) if the helmet and chin straps do not hold the head securely such that immobilization of the helmet does not also immobilize the head; and (4) if the helmet prevents immobilization in an appropriate position.44
If removal of the helmet or shoulder pads is required, the helmet and shoulder pads should always be removed simultaneously or neutral spine alignment will be lost.55,56 Football shoulder pads elevate the torso of a supine athlete to the same height as a helmeted head. The front of the shoulder pads can be opened to allow access to cardiopulmonary resuscitation and defibrillation if needed without actually removing the shoulder pads. Indications to remove the shoulder pads include: (1) the helmet is being removed; (2) injuries require full access to the shoulder area; and (3) ill-fitting shoulder pads preclude adequate spinal immobilization.44 To remove football shoulder pads, cut the jersey and other shirts from neck to waist and from midline to the end of each sleeve, cut all straps used to secure the pads to the torso, and cut the laces or straps over the sternum. Cervical stabilization is maintained by placing one’s forearms on the athlete’s chest while holding the maxilla and occiput. With assistance on both sides, the patient is lifted and the shoulder pads removed by spreading apart the front panels and pulling them around the head.44
Immobilization and transportation
Manual stabilization of the head, neck, and shoulders should be performed as the athlete is being assessed. If transport is indicated, the athlete will need to be immobilized to an appropriate immobilization device, usually a standard spine board. To transfer a supine athlete to a spine board, the Inter-Association Task Force recommends a six-plus-person lift technique of the athlete onto a rigid long spine board.44 One person is responsible for stabilization of the head and neck and will lead the team through all commands to lift the athlete and then lower onto the backboard. The rescuer’s hands should be placed palm-up underneath the shoulder pads, with the athlete’s head resting between the rescuer’s forearms.
To transfer an athlete who is prone or face down, a log-rolling technique is recommended (Fig. 126.9). This is performed with a minimum of four persons with one designated to maintain stabilization of the head and neck. If the prone athlete is not breathing, the athlete should be log-rolled immediately. The athlete can later be lifted a second time onto the spine board for immobilization as described above. If the athlete is conscious, the log-roll should be delayed until the backboard is available.
Prevention of secondary injury
The pathophysiology of irreversible spinal cord injury involves not only the primary injury but also a cascade of secondary injury including neuronal edema, hypoxia, and aberration of cell membrane potential. Spinal cord resuscitation in an attempt to prevent secondary injury is recommended and supported by the National Acute Spinal Cord Injury Study.57,58 This includes respiratory and hemodynamic support to facilitate spinal cord perfusion, prompt relief of spinal cord deformation through controlled realignment and stabilization, and intravenous administration of corticosteroids.57,58 Intravenous methylprednisolone is given with a loading dose of 30 mg/kg body weight over 1 hour and is recommended as soon as possible, preferably within the first 4 hours after injury. This is followed by a subsequent dose of 5.6mg/kg body weight administered over the next 23 hours.
1 Cantu RC, Mueller FO. Fatalities and catastrophic injuries in high school and college sports. Phys Sports Med. 1999;27:35-50.
2 Funk FF, Wells RE. Injuries to the cervical spine in football. Clin Orthop. 1975;109:50-58.
3 Carvell JE, Fuller DJ, Duthie RB, et al. Rugby football injuries to the cervical spine. Br Med J (Clin Res Ed). 1983;286:49-50.
4 Silver JR. Injuries to the spine sustained in rugby. Br Med J (Clin Res Ed). 1984;288:37-43.
5 McCoy GF, Piggot J, Macafee AL, et al. Injuries of the cervical spine in schoolboy rugby football. J Bone Joint Surg [Br]. 1984;66:500-503.
6 Tator CH, Edmonds VE. National survey of spinal injuries to hockey players. Can Med Assoc J. 1984;130:875-880.
7 Wu WQ, Lewis RC. Injuries to the cervical spine in high school wrestling. Surg Neurol. 1985;23:143-147.
8 Ghiselli G, Schaadt G, McAllister DR. On-the-field evaluation of an athlete with a head or neck injury. Clin Sports Med. 2003;22:445-465.
9 Cantu RC, Mueller FO. Catastrophic spine injuries in American football. 1977–2001. Neurosurgery. 2003;53:358-362.
10 Cantu RC. Return to play guidelines after head injury. Clin Sports Med. 1998;17:45-59.
11 Quality Standards Subcommittee. Practice parameter: the management of concussion in sports (summary statement). Report of the Quality Standards Subcommittee. Neurology. 1997;48:581-585.
12 Collins MW, Iverson GL, Lovell MR, et al. On-field predictors of neuropsychological and symptom deficit following sports-related concussion. Clin J Sport Med. 2003;13:222-229.
13 Collins MW, Hawn KL. The clinical management of sports concussion. Curr Sports Med Rep. 2002;1:12-22.
14 Lovell MR, Collins MW. New developments in the evaluation of sports-related concussion. Curr Sports Med Rep. 2002;1:287-292.
15 McCrea M, Guskiewicz KM, Marshall SW, et al. Acute effects and recovery time following concussion in collegiate football players: the NCAA Concussion Study. JAMA. 2003;290:2556-2563.
16 Schneider RC. Serious and fatal neurosurgical football injuries. Clin Neurosurg. 1964;12:226-236.
17 Burke DC. Hyperextension injuries of the spine. J Bone Joint Surg [Br]. 1971;53:3-12.
18 Edeiken-Monroe B, Wagner LK, Harris JHJr. Hyperextension dislocation of the cervical spine. Am J Roentgenol. 1986;146:803-808.
19 Penning L. Some aspects of plain radiography of the cervical spine in chronic myelopathy. Neurology. 1962;12:513-519.
20 Eismont FJ, Clifford S, Goldberg M, et al. Cervical sagittal spinal canal size in spine injury. Spine. 1984;9:663-666.
21 Torg JS, Guille JT, Jaffe S. Injuries to the cervical spine in American football players: current concepts review. J Bone Joint Surg [Am]. 2002;84:112-122.
22 Torg JS, Sennett B, Pavlov H, et al. Spear tackler’s spine. An entity precluding participation in tackle football and collision activities that expose the cervical spine to axial energy inputs. Am J Sports Med. 1993;21:640-649.
23 Torg JS, Quedenfeld TC, Burstein A, et al. National football head and neck injury registry: report on cervical quadriplegia, 1971 to 1975. Am J Sports Med. 1979;7:127-132.
24 Schneider RC. Head and neck injuries in football. Mechanisms, treatment, and prevention. Baltimore: Williams and Wilkins, 1973.
25 Torg JS, Vegso JJ, O’Neill MJ, et al. The epidemiologic, pathologic, biomechanical, cinematographic analysis of football-induced cervical spine trauma. Am J Sports Med. 1990;18:50-57.
26 Maroon JC. ‘Burning hands’ in football spinal cord injuries. JAMA. 1977;238:2049-2051.
27 Clancy WG, Brand RL, Bergfeld JA. Upper trunk brachial plexus injuries in contact sports. Am J Sports Med. 1997;5:209-215.
28 Sallis RE, Jones K, Knopp W. Burners. Offensive strategy in an underreported injury. Phys Sports Med. 1992;20:47-55.
29 Watkins RL. Nerve injuries in football players. Clin Sports Med. 1986;5:215-246.
30 Levitz CL, Reilly PJ, Torg JS. The pathomechanics of chronic, recurrent cervical nerve root neurapraxia. The chronic burner syndrome. Am J Sports Med. 1997;25:73-76.
31 Torg JS, Pavlov H, Genuario SE, et al. Neurapraxia of the cervical spinal cord with transient quadriplegia. J Bone Joint Surg [Am]. 1986;68:1354-1370.
32 Schneider RC, Reifel E, Crisler HO, et al. Serious and fatal football injuries involving the head and spinal cord. JAMA. 1961;177:362-367.
33 Brigham CD, Adamson TE. Permanent partial cervical spinal cord injury in a professional football player who had only congenital stenosis. A case report. J Bone Joint Surg [Am]. 2003;85A:1553-1556.
34 Torg JS, Corcoran TA, Thibault LE, et al. Cervical cord neurapraxia: classification, pathomechanics, morbidity, and management guidelines. J Neurosurg. 1997;87:843-850.
35 Wolfe BS, Khnilnani M, Malis L. The sagittal diameter of the bony cervical spinal canal and its significance in cervical spondylosis. J M Sinai Hosp. 1956;23:283-292.
36 Pavlov H, Torg JS, Robie B, et al. Cervical spinal stenosis: determination with vertebral body ratio method. Radiology. 1987;164:771-775.
37 Kang JD, Figgie MP, Bohlman HH. Sagittal measurements of the cervical spine in subaxial fractures and dislocations. An analysis of two hundred and eighty-eight patients with and without neurological deficits. J Bone Joint Surg [Am]. 1994;76:1617-1628.
38 Matsuura P, Waters RL, Adkins RH, et al. Comparison of computerized tomography parameters of the cervical spine in normal control subjects and spinal cord-injured patients. J Bone Joint Surg [Am]. 1989;71:183-188.
39 Herzog RJ, Wiens JJ, Dillingham MF, et al. Normal cervical spine morphometry and cervical spinal stenosis in asymptomatic professional football players. Plain film radiography, multiplanar computed tomography, and magnetic resonance imaging. Spine. 1991;16(6Suppl):S178-S186.
40 Resnick D. Degenerative disease of the spine. In: Diagnosis of bone and joint disorders. Philadelphia: WB Saunders; 1981:1408-1415.
41 Cantu RC. Cervical spine injuries in the athlete. Semin Neurol. 2000;20:173-178.
42 Cantu RC. Functional cervical spinal stenosis: a contraindication to participation in contact sports. Med Sci Sports Exerc. 1993;25:1082-1083.
43 Firooznia H, Ahn JH, Rafii M, et al. Sudden quadriplegia after a minor trauma. The role of preexisting spinal stenosis. Surg Neurol. 1985;23:165-168.
44 The Inter-Association Task Force for Appropriate Care of the Spine-Injured Athlete. Prehospital care of the spine-injured athlete. Dallas, TX: National Athletic Trainers Association, March 2001.
45 Cantu RV, Cantu RC. Guidelines for return to contact sports after transient quadriplegia. J Neurosurgery. 1994;80:592-594.
46 Cantu RC. Transient quadriplegia: to play or not to play. Sports Med Digest. 1994;16:1-4.
47 Torg JS, Naranja RJJr, Palov H, et al. The relationship of developmental narrowing of the cervical spinal canal to reversible and irreversible injury of the cervical spinal cord in football players. J Bone Joint Surg [Am]. 1996;78:1308-1314.
48 Torg JS, Pavlov H, O’Neill MJ, et al. The axial load teardrop fracture. A biomechanical, clinical, and roentgenographic analysis. Am J Sports Med. 1991;19:355-364.
49 Torg JS, Truex RCJr, Marshall J, et al. Spinal injury at the level of the third and fourth cervical vertebra from football. J Bone Joint Surg [Am]. 1977;59:1015-1019.
50 Bailes JE, Hadley MN, Quigley MR, et al. Management of athletic injuries of the cervical spine and spinal cord. Neurosurgery. 1991;29:491-497.
51 Swartz EE, Norkus SA, Armstrong CW, et al. Face-mask removal: movement and time associated with cutting of the loop straps. J Athl Train. 2003;38:120-125.
52 Angotti DD, Hoenshel RW, Kleiner DM. The most efficient technique to using the Face Mask Extractor. J Athl Train. 2000;35(Suppl 2):S60.
53 Kleiner DM, Knox KE. An evaluation of techniques used by athletic trainers when removing a facemask with the Trainers Angel. J Athl Train. 1995;30(Suppl 2):7.
54 Knox K, Kleiner DM. The efficiency of tools used to retract a football helmet face mask. J Athl Train. 1997;32:211-215.
55 Swenson TM, Lauerman WC, Blanc RO, et al. Cervical spine alignment in the immobilized football player: radiographic analysis before and after helmet removal. Am J Sports Med. 1997;25:226-230.
56 Gastel JA, Palumbo MA, Hulstyn MJ, et al. Emergency removal of football equipment: a cadaveric cervical spine injury model. Ann Emerg Med. 1998;32:411-417.
57 Bracken MB, Shepard MJ, Collins WF, et al. A randomized, controlled trial of methylprednisolone or naloxone in the treatment of acute spinal-cord injury. Results of the Second National Acute Spinal Cord Injury Study. N Engl J Med. 1990;322:1405-1411.
58 Bracken MB, Shepard MJ, Holford TR, et al. Administration of methylprednisolone for 24 or 48 hours or tirilazad mesylate for 48 hours in the treatment of acute spinal cord injury. Results of the Third National Acute Spinal Cord Injury Randomized Controlled Trial. National Acute Spinal Cord Injury Study. JAMA. 1997;277:1597-1604.