Chapter 15 Nuclear medicine techniques in hepatobiliary and pancreatic disease
Positron Emission Tomography
Physics
Some elements undergo radioactive decay by ejecting a positron from their nucleus (Table 15.1). A positron is a nuclear particle that has the same mass as an electron, but it has a positive charge. When ejected, the positron moves through surrounding tissues for 1 mm or so, until it eventually combines with a nearby electron, and the mass of both particles is converted to two photons of energy (γ-rays) of 511 keV, which are emitted 180 degrees apart. The PET camera detects these two photons.
Camera Issues
Dedicated Positron Emission Tomography Systems
PET machines are constructed using multiple rings of circularly arranged detectors comprising scintillator crystals, such as bismuth germanium oxide crystals, lutetium oxyorthosilicate, or gadolinium orthosilicate, usually without associated collimators. These cameras all work in coincidence, meaning that a true count is generated only when the two 511 keV γ-rays emitted during positron decay simultaneously strike a set of paired detectors (Fig. 15.1). These types of PET imaging devices have high sensitivity. A large amount of usable data is obtained from a given amount of material injected into a patient so that the camera is able to detect small accumulations of injected tracer. The resolution of these machines is defined as the smallest distance between two sources of radiation, whereby the imaging instrument is able to show two sources of radiation rather than one. This distance is currently around 5 mm for most clinical systems; animal imaging devices are now available that have 1-mm resolution (for 18F).
Computed Tomography/Positron Emission Tomography Hybrid Systems
γ-Rays originating in the center of the body have to pass through tissue for a considerable distance to reach the detector, and if one or more of the paired annihilation photons are absorbed, that decay is not recorded as a true count. An anatomically central source would be underrepresented if no attempt were made to correct for differing body tissue density and the depth of the origin of the γ-rays. One of the major advantages of PET imaging is that an accurate correction can be made for this process of attenuation, or loss of counts. Combination machines have been developed (Beyer, 2000) that contain a CT and a PET device in the same imaging gantry. Attenuation correction maps extrapolated from the CT acquisition overcome the attenuation of activity originating in the center of the body. In this way, the PET image becomes quantitative so that the process of computerized data reconstruction ultimately generates a map of the three-dimensional distribution of radioactivity. It is essential that attenuation be performed to obtain quality information from areas such as the porta hepatis, particularly if quantification of radioactivity content in the lesion is to be attempted.
Using PET CT enables coregistration of structural and functional information; this promises near-perfect anatomic localization of areas of functional changes. Some drawbacks with these machines are related to misregistration of the CT data used for attenuation correction in the region of the diaphragm and around the heart, related to cardiorespiratory motion (Beyer et al, 2003). At present there are multiple approaches to this issue but no universally accepted methodology of addressing it.
Biochemistry
The most common radiopharmaceutical used in PET imaging is FDG, a glucose analogue that crosses cell membranes, sharing the glucose transporter molecules used by glucose. Similar to glucose, it undergoes phosphorylation by the enzyme hexokinase. The resulting molecule, FDG-6-phosphate, is polar; it cannot cross cell membranes and is not a substrate for glucose-6-phosphate isomerase, which converts glucose-6-phosphate to fructose-6-phosphate. FDG-6-phosphate is not a substrate for glucose-6-phosphatase in any tissue except the normal hepatocyte. The net effect is a continuous accumulation and retention of FDG in cells (Fig. 15.2).
Warburg (1930) reported that cancer cells show increased rates of glycolysis compared with normal cells. This discovery has stood the test of time and now underpins the utility of FDG PET imaging in cancer. Glucose metabolism is the most efficient form of energy transfer, and all normal tissues have characteristic glucose metabolic rates. The brain has a high rate of glucose metabolism, and the lung and white fat have low rates. A clump of tumor cells is easier to detect in the lung or in fat than in the brain, because the target-to-background ratio is high in these tissues. The liver has an intermediate glucose metabolic rate. Active inflammatory cells exhibit an increased rate of glycolysis, and sites of active inflammation exhibit increased FDG uptake, although in general the rate of glucose metabolism is lower in inflammatory sites than in most tumors. Sarcoidosis in particular can be quite FDG avid and may have similar patterns and degrees of uptake as some malignancies, such as lymphoma and small cell lung cancer. In such cases, clinical judgment is called into play just as it is in the interpretation of opaque masses in the lung on CT scan.
FDG PET Imaging in Patients with Colorectal Cancer Metastatic to Liver (See Chapter 81A)
Historical Review
The earliest human study of FDG PET in colorectal cancer was performed by Yonekura and colleagues in 1982; this study of large hepatic metastases in three patients with colorectal cancer was successful and showed that these tumors were highly glucose avid. Numerous publications from multiple centers have established that colorectal cancer is readily detected on FDG PET, and colorectal cancer is a common reason for referral of patients for a scan. The value of FDG PET in colorectal cancer patients is based on the consistently accelerated levels of glycolysis seen in colorectal cancer, which results in high FDG uptakes on PET scanning.
Technique
FDG PET studies (Figs. 15.3 to 15.13) are typically performed as a screening procedure that obtains a whole-body image in an attempt to find as many sites of disease as possible. The usual FDG PET CT study of the whole body takes between 7 and 20 minutes, depending on the machine used. The amount of time spent imaging the liver alone is 2 to 4 minutes. If an acquisition of the liver were performed for 1 hour, the accuracy of PET would improve over the values reported in the literature. Nevertheless, FDG PET imaging performs remarkably well with an extraordinary safety profile, with no adverse effects reported in more than 81,000 doses administered over a 4-year period in 22 centers (Silberstein, 1998).
Staging
Preoperative imaging identifies sites of disease in relation to the vascular anatomy of the liver and excludes disease that renders the patient inoperable. Cross-sectional imaging with CT, MRI, and US are the methods of choice to elucidate the vascular anatomy of the liver (see Chapters 13, 16, and 17); nuclear medicine plays no direct role. A secondary role of preoperative imaging is in the demonstration of disease that renders the patient nonresectable. In the best of centers, many patients who are scheduled for hepatic resection are found intraoperatively to have disease that if shown initially would have led to a nonoperative treatment plan (Jarnagin et al, 1999). In a series reported by Scheele and colleagues in 1995, the rates of resection with curative intent steadily increased from 1960 to 1992. Among the many reasons for this increase are improved treatment options and better preoperative imaging that allows more accurate selection of patients with potentially resectable disease. Conventional cross-sectional imaging with helical CT in arterial and portal phases is the standard study for detection of colorectal metastases, and MRI may also be used in conjunction for preoperative evaluation. The previous standard, CT arterial portography, has been supplanted by helical CT, which has equivalent sensitivity for detection of hepatic metastases and results in fewer false positives (Valls et al, 1998).
Fluorodeoxyglucose Positron Emission Tomography for Detection of Hepatic Metastases
A head-to-head retrospective comparison of CT versus FDG PET clinical readings showed an advantage of FDG PET over CT (Table 15.2). The values reported were 23 with disease (n = 58) and FDG PET sensitivity of 95% and specificity of 100% compared with CT sensitivity of 74% and specificity of 85% (Ogunbiyi et al, 1997). The limitations of this study are its retrospective nature and the lack of information regarding the use of intravenous contrast material; in addition, the FDG PET scans were read with knowledge of the CT, whereas the converse may not have been true.
Another report of 34 patients with colorectal cancer that was potentially resectable, comparing CT with contrast and FDG PET, revealed FDG PET had a sensitivity of 89% and specificity of 67% compared with CT, with a sensitivity of 100% and specificity of 14%, giving an overall accuracy of 85% for FDG PET versus 82% for CT (Lai et al, 1996). A further series of 24 patients, 19 of whom had liver metastases, compared CT, computed tomography in arterial portography (CTAP), and FDG PET on a lesion-by-lesion basis. In terms of accuracy, FDG PET performed better than the radiologic methods: 93% compared with 76% for CT and CTAP. The reason the more sensitive CTAP performed worse than FDG PET is because the specificity of CTAP was only 9%. A separate group reported similar findings; FDG PET accuracy was 92% compared with CT arterial portography (CTAP) and CT accuracy of 80% and 78%, respectively. The low specificity of CTAP (5%) worked to its detriment (Delbeke et al, 1997). Many centers now combine a dedicated CT of the liver as part of the PET CT examination.
Fluorodeoxyglucose Positron Emission Tomography for Detection of Extrahepatic Disease
There has been a remarkable consistency in the rate that FDG PET alters the preoperative stage of a patient after standard-of-care preoperative diagnostic imaging (Table 15.3). In a wide variety of tumors, including colorectal cancers, about 30% of patients conventionally staged have their treatment plans altered as a result of FDG PET. The National Oncology PET Registry (NOPR) study of nearly 23,000 patients revealed a management rate of change of 36% (Hillner et al, 2008). A report of 34 patients with potentially resectable hepatic disease (Lai et al, 1996) found retroperitoneal nodal metastases in six patients, pulmonary metastases in three patients, and locoregional recurrences in two patients. One patient had unexpected disease in two sites, and FDG PET had an influence on clinical management in 10 of 34 patients (29%). The experience at Memorial Sloan-Kettering Cancer Center in the preoperative assessment of patients at high risk of recurrence after hepatic resection of colorectal cancer is similar. We found nine (23%) of 40 patients had their management radically altered owing to FDG PET findings of extensive unresectable disease, with management in another seven patients being influenced by the FDG PET findings confirming isolated, resectable extrahepatic disease, giving a total of 40% of patients in whom management was influenced.
Reference | No. Considered for Surgical Resection | Management Changed as a Result of FDG PET (%) |
---|---|---|
Ogunbiyi et al, 1997 | 23 | 43 |
Flamen et al, 1999 | 37 | 11 |
Lai et al, 1996 | 34 | 29 |
Vitola et al, 1996b | 24 | 25 |
Delbeke et al, 1997* | 61 | 28 |
Schiepers et al, 1995† | 76 | 26 |
Valk et al, 1998 | 38 | 32 |
FDG PET; fluorodeoxyglucose positron emission tomography.
* All patients in the study group included as potentially hepatic resection patients were not reported as a separate group.
† 25 unexpected lesions found in 20 patients.
A real strength of FDG PET is its ability to detect extrahepatic disease and alter the surgical plan for the patient. Most if not all lesions detected with FDG PET are within the resolution of conventional imaging modalities and theoretically should be detectable, but lesions either are not recognized as being abnormal (“missed”), or they appear normal. One reason why FDG PET performs so well is that the accelerated glucose metabolism of the tumors makes the tumor stand out against the background of normal tissue, just as the light of a match is easily discerned, even at a distance, on a dark night. The effectiveness of FDG PET is due to its ability to show lesions that mimic a normal structure, particularly intraperitoneal disease (see Figs. 15.4, 15.7, and 15.10), which mimics unopacified loops of bowel and normal-sized lymph nodes replaced by tumor. The influence of a finding of extrahepatic disease depends on the aggressiveness of the approach to disease the treating clinician and the patient wish to undertake. Local pelvic recurrence, if completely resectable, should not prevent a patient from undergoing hepatic resection. FDG PET is not a panacea, however, because an imaging study does not change the biology of a disease, and the fact that disease cannot be detected with FDG PET does not rule out micrometastatic disease. It is important in terms of informed consent that patients appreciate that a workup including FDG PET does not rule out the later appearance of a distant metastasis.
Evaluation of Patients After Treatment
There is a dearth of literature on the relative sensitivities of FDG PET and conventional imaging in the detection of recurrent intrahepatic disease after liver surgery (see Figs. 15.11 to 15.13). Some patients develop subphrenic abscesses after resection, which are difficult to differentiate from recurrent tumor; however, even in this situation, FDG PET seems to perform well. In an initial report of seven patients who had undergone resection previously, PET correctly differentiated recurrent disease from postsurgical change in all; CT misdiagnosed two of six, with one false-positive result and one false-negative result; CTAP misdiagnosed three of five patients, all false-positive results (Vitola et al, 1996b). In the assessment of the effectiveness of chemoembolization (see Chapter 83), FDG PET has been shown to be useful (Vitola et al, 1996a), although an increment over CT and tumor markers has yet to be shown.
Preliminary investigations suggest FDG PET performed within 24 hours after hepatic cryotherapy (see Chapter 85B) can predict sites of recurrent disease based on the pattern of FDG uptake (Akhurst et al, 1999). This is an exciting development, because it helps clinicians identify patients in whom cryotherapy has been unsuccessful (see Fig. 15.13). The early demonstration of potential treatment failure can assist in further treatment planning. We also have been using FDG PET to follow patients who are being treated with hepatic artery pump therapy (see Chapter 6). In two cases, the information derived from FDG PET would not have been obtainable from conventional imaging, because the effects of cryotherapy persist on CT and US, leading to an inability to differentiate posttreatment change from residual tumor.
Management of Patients with Hepatocellular Carcinoma (See Chapter 80)
Diagnosis
In general, the ease of lesion detection on imaging depends on the target/background ratio. The higher the target-to-background ratio, the easier it is to detect a lesion. A low-grade lesion is likely to have a metabolic profile similar to the surrounding normal tissues. From an imaging standpoint, the liver is a relatively homogeneous solid structure. Because hepatomas are derived from liver tissue, they share many imaging characteristics with a normal liver and are therefore difficult to detect (Schroder et al, 1998). A study aiming to evaluate FDG PET in the diagnosis of liver masses found all primary sarcomas and adenocarcinoma metastases and all cholangiocarcinomas had increased FDG uptake values, but that seven of 23 patients with hepatocellular carcinoma (HCC) had poor FDG uptake (Delbeke et al, 1998). A sound pathophysiologic basis underlies this reduced efficacy, as shown by Torizuka and colleagues (1995) in a study of HCC greater than 3 cm in size. They showed that undifferentiated HCC had a pattern of continual FDG uptake shared by most solid tumors, whereas a moderately differentiated HCC showed a pattern of uptake similar to the normal liver, with an initial rise and then a slow fall. The difference in pattern was due to marked differences in the activity of transporters carrying glucose into the cell and in hexokinase activity, but it may also be due to a similarity between normal liver and HCC in terms of the rate of dephosphorylation of FDG-6-phosphate.
Treatment Planning and Assessment of Prognosis
The survival rate of HCC patients seems to correlate with the degree of FDG uptake into the tumor, with patients with low FDG uptake showing a longer survival than patients with high FDG uptake (Enomoto et al, 1991). FDG uptake may correlate with the degree of expression of p53 (Schroder et al, 1998; Yoon et al, 1998), a known factor in hepatocellular carcinogenesis (Hui et al, 1998). In a similar fashion to other tumors, FDG PET has been shown to be effective in monitoring lipiodol chemoembolization in HCC (Torizuka et al, 1994).
Cholangiocarcinoma (See Chapter 50A, Chapter 50B, Chapter 50C, Chapter 50D )
The detection of cholangiocarcinoma in patients with primary sclerosing cholangitis can be difficult, because imaging findings of the underlying disease may mimic those of tumor. Preliminary reports indicate a potential role of FDG PET, because cholangiocarcinoma seems to be FDG avid (Delbeke et al, 1998; Keiding et al, 1998), although this may be limited to follow-up assessment (Berr et al, 1999). If active cholangitis is present, the mucosal inflammation may obscure small sites of carcinoma in situ. If strictures are stented, the resultant mucosal irritation also may make the diagnosis of malignancy more difficult. Screening for cholangiocarcinoma may require interrogation of a different metabolic pathway, such as that of protein synthesis or proliferation.
In an analysis of data at Memorial Sloan-Kettering Cancer Center, 82 patients with cholangiocarcinoma were studied; 24% of the 62 newly diagnosed patients had their management changed as a result of the detection of occult metastases. FDG PET detected 95% of the intrahepatic tumors and 69% of the extrahepatic tumors; this is not surprising, because central cholangiocarcinomas are more often clinically silent and present later in terms of size than extrahepatic tumors. In terms of the detection of metastases, 100% of metastases from intrahepatic tumors and 93% from the extrahepatic group were detected (Corvera et al, 2004). An example of a cholangiocarcinoma detected with FDG PET is shown in Figure 15.14.
Focal Liver Lesions
A focal liver lesion in a patient with a recent history of colorectal cancer and increasing carcinoembryonic antigen (CEA) is likely due to a metastasis; this partly explains the high success rates in detecting malignant disease reported by some groups (Delbeke et al, 1998). In a separate group, FDG PET was not found to be useful in the characterization of liver masses in patients with chronic hepatitis C infection (Schroder et al, 1998).
Diagnosis and Follow-up of Pancreatic Diseases
Pancreatic Cancer (See Chapter 58A, Chapter 58B )
The clinical presentation of painless jaundice in a middle-aged individual should immediately raise the clinical suspicion of the presence of a cancer obstructing the common bile duct; this is most commonly due to a cancer of the head of the pancreas. Classic approaches to imaging of masses of the head of the pancreas still have validity, with US being the least invasive test available. If surgical resection is to be considered, MRI is likely to give the best possible local soft-tissue discrimination, allowing for planning of a surgical approach and the best assessment of the pancreatic and biliary ducts. Most solid pancreatic adenocarcinomas are FDG avid (Fig. 15.15), allowing for the screening of patients for unexpected distant disease and for the possibility of assessment of the response of the pancreatic mass to induction therapy, if that is considered appropriate. The implementation of PET CT allows much greater confidence in the localization of focal epigastric FDG uptake to a particular anatomic structure; previously epigastric FDG uptake was described as a regional, rather than an organ-specific, phenomenon.
The mechanism of FDG uptake into cells is exactly the same as for unlabeled glucose, so according to the laws of mass action, a high serum concentration of unlabeled glucose (as seen in diabetics with insulin resistance) reduces the amount of FDG uptake into cancer cells, leading to a reduction in sensitivity. In a study of 106 patients with pancreatic masses, of which 32 were benign, PET showed an overall sensitivity of 85%, a specificity of 84%, a negative predictive value of 71%, and a positive predictive value of 93%. In a subgroup of patients with normal serum glucose levels (n = 72), the results were 98%, 84%, 96%, and 93%, respectively (Zimny et al, 1997). Insulin-sensitive patients also present an interesting problem because incorrect timing of the FDG injection in relation to the insulin injection may lead to much of the injected FDG being driven into muscle, reducing tumor sensitivity.
Cystic pancreatic masses (see Chapter 57) present a difficult imaging problem, because they consist of a thin layer of cells surrounding a large void (Fig. 15.16). A large report of 599 patients with cystic masses of the pancreas found 280 (46.8%) were pseudocysts, 102 (17%) were intraductal papillary mucinous tumors, 100 (16.7%) were serous tumors, 62 (10.4%) were mucinous tumors, 39 (6.5%) were mucinous cystadenocarcinoma, and 16 (2.7%) were solid papillary cystic tumors (Bassi et al, 2003). Clues that a cystic mass of the pancreas may be neoplastic, rather than due to a pseudocyst, include the absence of symptoms (Fernandez-del Castillo et al, 2003) and the presence of calcification in the wall of the cyst, rather than the entire pancreas, as is seen in chronic pancreatitis. Multiloculated cystic lesions favor a neoplastic origin, rather than the single cyst seen in a pseudocyst.
Because of the relatively low resolution of all nuclear medicine techniques and partial volume averaging, it is extremely problematic to attempt to characterize a cystic pancreatic mass. Two tumors with the same metabolic rate may have different standardized uptake value measures related solely to the thickness of the cyst wall (Fig. 15.17). If a portion of the mass is thick, characterization can be attempted, but most often this portion of a lesion is adjacent to normal tissues, making accurate characterization difficult. In addition, if inflammation or infection of the pancreatic mass is suspected, the specificity of focal FDG uptake as a predictor of malignancy is reduced dramatically. For this reason, a single FDG PET scan is unlikely to be completely successful in characterizing cystic pancreatic masses.
Follow-up of patients after resection typically is performed with CT or MRI, because these two modalities are very sensitive in the detection of small liver lesions. FDG PET usually is reserved as a modality to document unexplained increases in CEA or other tumor markers or to characterize indeterminate masses seen on other cross-sectional imaging. Novel tracers targeting the pancreas include radioiodinated phenylalanine derivatives, which seem to have preclinical promise in that they show higher affinity for pancreatic tumor xenografts in murine models than FDG with less uptake in inflammatory lesions. These compounds may lead to improved specificity in the diagnosis of pancreatic malignancy in patients with coexistent chronic pancreatitis (Hellwig et al, 2005).
Assessment of Pancreatic Reserve
Exocrine Pancreatic Function.
An experimental approach to the assessment of pancreatic exocrine function illustrates the wide-ranging potential of PET. 11C-methionine is a PET radiopharmaceutical that is taken up into normal pancreas and liver. Initial studies of pancreas involved the use of selenium 75-methionine in the 1960s; the production of 11C-methionine was described in 1976 (Comar et al, 1976). Good correlations of secretin-induced duodenal amylase and bicarbonate concentrations with the pancreas-to-liver 11C-methionine ratios 4.5 minutes after injection of the methionine have been reported (Takasu et al, 2001). The investigators found that a pancreas-to-liver 11C-methionine ratio at 4.5 minutes after injection of greater than 1.25 had 100% sensitivity and 86% specificity for the detection of chronic pancreatic impairment.
Endocrine Pancreatic Imaging.
Because of the 100-fold fewer β cells compared with exocrine cells, β cell imaging poses additional problems over and above imaging of exocrine pancreatic cells. It has been suggested that a successful β-cell imaging agent must have a β/exocrine cell affinity ratio of greater than 1000 : 1 (Sweet et al, 2004). Realization of this problem has focused efforts on specific β-cell surface markers. The use of the monoclonal antibody to image β cells successfully in mice has been described (Moore et al, 2001). Such approaches represent a proof of principle of one method of following up patients in experimental programs aimed at preserving β-cell function in the setting of diabetes and pancreatic transplantation.
Gastroenteropancreatic Neuroendocrine Tumors (See Chapter 81b)
Gastroenteropancreatic neuroendocrine tumor imaging and therapy is a developing field. The standard bearer of somatostatin imaging, 111In octreotide, which will be discussed later in this chapter, is increasingly being substituted with gallium 68 (68Ga) somatostatin receptor imaging. 68Ga, a generator-derived, 68-minute half-life positron emitter, has been coupled to a variety of somatostatin analogues; the only difference between the somatostatin analogue [DTPA0,Tyr3] octreotate and [DTPA0,Tyr3] octreotide is that the C-terminal threoninol is replaced with threonine. Octreotate binds with greater affinity to somatostatin receptor subtype II than octreotide, making this tracer particularly suitable for tumors of pancreatic origin. The advantages of using a PET camera over a SPECT camera are significant because of improved quantification and resolution. A parallel development in radiopeptide therapy has occurred, whereby the same somatostatin analogues have been bound using the same chelators to lutetium-177, a 6.71-day half-life radioisotope that has both a 208 keV γ-emission suitable for imaging and a β-emission with an average energy of 0.13 MeV, which provides therapeutic efficacy. Lutetium radiopeptide therapy has proved to be less nephrotoxic than yttrium-90 (90Y) radiopeptide therapy, particularly when administered under the cover of renoprotective amino acid infusions using lysine and arginine. Administration of 5-fluorouracil (5-FU) at a starting dose of 200mg/m2 for a 3-week period around the time of the lutetium-177 (177Lu) is well tolerated and has the radiosensitizing potential to increase the tumoricidal effects of the 177Lu (Hubble et al, 2010).
68Ga octreotate imaging is complementary to the use of FDG PET and cross-sectional imaging. Growing neuroendocrine tumors that are moderately to intensely FDG avid but 68Ga octreotate negative will not respond to radiopeptide therapy, and either local ablative or other systemic approaches should be evaluated. Growing neuroendocrine tumors that are concordantly 68Ga octreotate and FDG avid are quite likely to respond to radiopeptide therapy, and following radiopeptide therapy reassessment with imaging at 6 to 12 months after treatment, such tumors will often demonstrate an FDG-avid component of the tumor that has diminished with preservation of the 68Ga octreotate–avid component, reflecting the relative sensitivity of the clones of cells to the radiation treatment (Figs. 15.18 and 15.19). Retreatment leads to long-term tumor control (Kwekkeboom et al, 2008), but caution must be exercised, as cumulative doses greater than 100 GBq have been associated with preleukemic states.
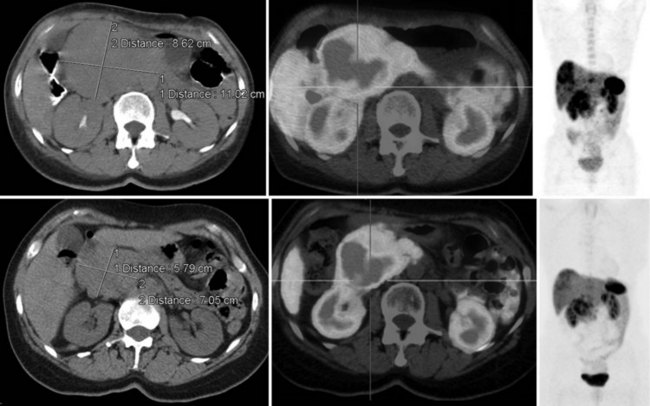
FIGURE 15.19 The same patient as in Figure 15.18, comprising in the top row a pretreatment computed tomography (CT) through the pancreatic mass, a fused 68Ga octreotate positron emission tomography (PET) CT image, and the whole-body 68Ga octreotate PET image performed at baseline. The same sequence is seen in the bottom row after nearly 3 years and five treatments of a total of 41 GBq of 177Lu octreotate therapy with concomitant 5-fluorouracil chemotherapy (200 mg/m2 given as a radiosensitizer). Note the reduction in size of the pancreatic mass that retains somatostatin receptor 2 expression, in spite of the near-complete resolution of FDG activity in the pancreatic mass shown in Figure 15.18.
Hepatobiliary Scintigraphy
Scintigraphic imaging of the hepatobiliary system aims to mimic the extraction of the precursor of bile from the blood, the formation of bile, and the excretion of bile into the bile ducts and gut. Iminodiacetic acid derivatives can be labeled with 99mTc. These compounds are extracted rapidly from the circulation by hepatocytes and are excreted into the biliary system without undergoing conjugation. The hepatic half-clearance time varies with the agent used (Table 15.4), and some renal excretion of iminodiacetic acid derivatives occurs with proportionally increased renal excretion in hepatocellular dysfunction (Fig. 15.20).
Name | Liver Half-Clearance Time (min) | Hepatic Uptake (%) |
---|---|---|
Mebrofenin | 17 | 98 |
Disofenin | 19 | 88 |
o-Diethyl | 37 | 82 |
o-Dimethyl | 42 | 84 |
p-Isopropyl | 59 | 85 |
p-n-Butyl | 107 | 94 |
Patient preparation is important in the performance and interpretation of hepatobiliary scintigraphy. Detailed clinical information is required for the proper interpretation of the studies. To image the gallbladder, the patient should be fasted for 2 to 24 hours. If a study is performed immediately postprandially, the gallbladder is contracted, leading to nonvisualization (Klingensmith et al, 1981). An overdistended gallbladder leads to nonvisualization in patients fasted for more than 24 hours (Larsen et al, 1982).
Normal Scan
A hepatobiliary study usually is acquired in many dynamic acquisitions; the immediate postinjection sequence is performed with rapid frame times (2 to 5 seconds) to assess perfusion (Fig. 15.21). The later images are acquired for a longer duration (2 to 5 min/frame) to assess the uptake and excretory phases (Fig. 15.22). Right lateral or oblique images often are needed to distinguish gallbladder activity from underlying renal pelvis or duodenal activity (Fig. 15.23).
After injection of the radiopharmaceutical tracer, a biphasic influx of the tracer to the liver is seen (see Fig. 15.21) that reflects delivery of tracer from the hepatic arterial and then the portal circulation. The hepatocytes are efficient at extracting the tracer, so uptake is rapid and leads to hepatic uptake quickly exceeding that of the cardiac blood pool. The hepatic activity reaches a peak at about 10 minutes after injection. The hepatocytes clear the tracer into the biliary canaliculi, and within 30 minutes, the right and left hepatic ducts and the common hepatic duct can be seen, although such detail may not be seen in very small infants. The gallbladder is seen in 90% of normal individuals within 30 minutes after injection, and 100% are seen within 1 hour. The bowel is seen in 80% of normal individuals within 60 minutes, and the remaining 20% eventually drain to the gut, with delayed drainage as a result of mild dehydration or mildly increased sphincter of Oddi dysfunction. In patients who have had a cholecystectomy, the gut usually can be seen by 20 minutes (Fig. 15.24; Sostre et al, 1992b), and bile reflux into the stomach often can be seen (Fig. 15.25; also see Fig. 15.22). If a patient has a contracted gallbladder, as is often seen in chronic cholecystitis, gallbladder filling may be delayed (Fig. 15.26).
Augmented Hepatobiliary Scintigraphy
Numerous drugs affect the function of the hepatobiliary tree and are used to improve the diagnostic efficacy of hepatobiliary scintigraphy. Phenobarbital is used to increase the production of bile in infants to improve the diagnostic accuracy of the test in cases of neonatal jaundice. Cholecystokinin infusion leads to increased bile flow, contraction of the gallbladder, and relaxation of the sphincter of Oddi. Nitroglycerin relaxes the sphincter of Oddi (Staritz, 1988), and morphine contracts it; this effect is promptly relieved by cholecystokinin or naloxone (Helm et al, 1988; Patch et al, 1991). The duration of increase of pancreatic cholecystokinin levels secreted in response to a fatty meal is much longer than the effects of infusion. A repeat dose of cholecystokinin given after a cholecystokinin infusion as premedication leads to an equivalent gallbladder ejection of bile. Patients who have had a prolonged fast can have one injection to empty the gallbladder to enable subsequent filling and a later injection to assess gallbladder ejection fraction in the same sitting, with no loss of diagnostic accuracy (Sostre et al, 1992a).
Right Upper Quadrant Pain
The differential diagnosis of right upper quadrant pain is beyond the scope of this chapter, but hepatobiliary scintigraphy has a role in the assessment of acute and chronic right upper quadrant pain (see Chapter 31). A 30% increase has been seen in the number of cholecystectomies performed since the 1990s. Another phenomenon is the change in the indications, with rates of operations for biliary dyskinesia, acute acalculous cholecystitis, and chronic acalculous cholecystitis increasing 348%, 139%, and 138%, respectively, in the same time frame (Johanning & Gruenberg, 1998). This study was a review of the International Classification of Diseases (9th revision) discharge codes, and the results may be due in part to a reporting bias, as these disease states become more widely appreciated; but they are more likely due to the reduced morbidity of the laparoscopic operative procedure. Preoperative testing with biliary scintigraphy predicts patients with acalculous biliary pain who are likely to benefit from cholecystectomy (Goncalves et al, 1998; Reed et al, 1993), and it should be performed in this patient group (Barron & Rubio, 1995).
Cholecystitis (See Chapter 30)
The diagnosis of acute cholecystitis is largely clinical, although ancillary tests are often performed to increase clinical confidence before surgery. The two competing imaging tests are US and hepatobiliary scintigraphy. The key finding on hepatobiliary imaging is nonvisualization of the gallbladder (see Fig. 15.25). Sensitivity rates of greater than 96% have been reported consistently by many groups (Freitas et al, 1982; Suarez et al, 1980; Szlabick et al, 1980; Velasco et al, 1982). An additional finding of a hot rim around the gallbladder fossa is strongly associated with a diagnosis of acute cholecystitis (Cawthon et al, 1984), but it is not 100% specific, as had previously been thought (Oates et al, 1996). The specificity of unaugmented hepatobiliary scintigraphy is lower, however, because prolonged fasting may lead to nonvisualization of the gallbladder. This finding has led to many investigators routinely using morphine augmentation (Choy et al, 1984) or cholecystokinin pretreatment (Eikman et al, 1975) to increase biliary tree pressure and show cystic duct patency. The addition of this step increases the specificity of hepatobiliary scintigraphy (Cabana et al, 1995; Chen et al, 1997; Choy et al, 1984; Fink-Bennett et al, 1991a) over and above delayed imaging (Kim et al, 1993). Morphine-augmented hepatobiliary scintigraphy in the detection of acute cholecystitis has sensitivity, specificity, positive predictive value, and negative predictive value of 95%, 99%, 97%, and 98%, respectively (Fink-Bennett et al, 1991a). These figures compare favorably with US, and hepatobiliary scintigraphy seems to be the diagnostic test of choice in the diagnosis of acute cholecystitis (Shea et al, 1994). US relies on a combination of sonographic findings, including the presence of stones, gallbladder wall thickening, perigallbladder fluid, and a positive Murphy sign over the gallbladder fossa rather than demonstration of cystic duct obstruction itself (Dykes et al, 1986; Lauritsen et al, 1988; Samuels et al, 1983; Soiva et al, 1986; van Weelde et al, 1986).
Chronic Cholecystitis
In patients with chronic cholecystitis, the crucial imaging finding is the presence of cholelithiasis, and this is best shown with US (Shea et al, 1994). Most patients with chronic cholecystitis show cystic duct patency. Delayed filling of the gallbladder is associated with chronic cholecystitis, but this is a nonspecific finding, because fasting may give the same appearance (see Fig. 15.26). US remains the diagnostic test of choice in the detection of chronic cholecystitis.
Chronic Acalculous Right Upper Quadrant Pain
Acalculous Cholecystitis and the Cystic Duct Syndrome.
Cystic duct syndrome is defined as fibrotic narrowing of the cystic duct lumen by 60%, kinking or adhesions of the duct seen at surgery, or both. Chronic cholecystitis is defined pathologically as hypertrophy of the gallbladder wall (>1.5 to 2 mm thick) and the presence of Rokitansky-Aschoff sinuses, a mononuclear infiltrate, foamy macrophages, diffuse muscularis hypertrophy, and yellow papillary nodules (Fink-Bennett et al, 1991b). Using a standardized protocol of cholecystokinin infusion (0.2 µg/kg administered over 3 minutes), a normal range for the ejection of bile from the gallbladder has been established. Fink-Bennett and colleagues (1991b) studied this group in detail. Patients with reduced gallbladder ejection fraction (<35% ejected in the first 20 minutes after cholecystokinin infusion) were considered abnormal. Of patients with an abnormal gallbladder ejection fraction, 108 (94%) of 115 had either cystic duct narrowing or chronic cholecystitis on pathology, with only 1 of 106 who had follow-up reporting no amelioration of symptoms; 69 of 78 (89%) who were medically managed had continued symptoms suggesting biliary colic. This study suggests the finding of reduced gallbladder ejection fraction in response to cholecystokinin is a strong indicator of the need for surgical intervention. Only seven (6%) of the 115 patients with histopathologic confirmation of disease had a gallbladder ejection fraction greater than 35%, a false-negative result. The negative predictive value of a normal gallbladder ejection fraction is 91%, with 130 of 143 patients with improvement after treatment for nongallbladder pathology having a gallbladder ejection fraction greater than 35% (Fink-Bennett et al, 1991b).
Postcholecystectomy Syndrome and Sphincter of Oddi Dysfunction (See Chapter 38).
The overall incidence of pancreatitis in patients undergoing endoscopic retrograde cholangiopancreatography (ERCP) manometry alone has been reported as 9.3%, with a 26% incidence if sphincterotomy is combined with manometry (Maldonado et al, 1999). The high rate of this side effect serves as a continued stimulus to investigate noninvasive methods for diagnosing SOD. Augmented hepatobiliary scintigraphy is a promising method in the noninvasive diagnosis of SOD. The most common abnormal finding with hepatobiliary scintigraphy is an obstructive pattern of biliary drainage, but some patients who have no symptoms at the time of the examination have a normal study. A variety of criteria for analysis have been developed using quantitative hepatobiliary scintigraphy (Roberts-Thomson et al, 1986). Augmentation techniques include an improvement in biliary drainage after amyl nitrate therapy (Madacsy et al, 1994) to exclude a fixed stenosis that would not respond to drug therapy and the development of an obstructive pattern with prostigmine-morphine administration observed by Madacsy and colleagues (1995), who suggested that only patients with prostigmine-morphine–induced obstruction should undergo manometry.
Biliary Tract Complications after Surgery (See Chapter 42A, Chapter 42B )
The complication rates of laparoscopic cholecystectomy decrease as the number of cases performed by a particular surgical group increases (Walker et al, 1993). Hepatobiliary scintigraphy is a powerful, noninvasive tool to detect bile leak and iatrogenic biliary obstruction (Reichelt, 1978). When there is clinical suspicion of bile duct injury after surgery, hepatobiliary imaging should be one of the first tests considered (Figs. 15.27 to 15.32). The degree of leak can be assessed, and this can assist in the triaging of patients with bile leak to conservative or operative repair. After bile drainage has been achieved, a follow-up scan can assist in the triaging of patients who require further intervention (Walker et al, 1992). Improved disease-free survival after hepatic resection of metastases has led to an increase in the complexity and aggressiveness of hepatic resection, including complex operations involving the biliary tree. Hepatobiliary scintigraphy can assess the efficacy of biliary drainage after these complex procedures.
Liver Transplantation (See Chapter 97A, Chapter 97B, Chapter 97C, Chapter 97D, Chapter 97E )
The major role of hepatobiliary imaging in liver transplant patients is in the postoperative assessment of the presence or absence of bile duct injury (Mochizuki et al, 1991; see Chapter 100). The noninvasive assessment of the integrity of a bile duct anastomosis is best done by hepatobiliary scintigraphy, because conventional imaging cannot characterize the nature of ascitic fluid. Bile duct stenoses do occur, and these are well shown with scintigraphy. Acute vascular rejection is an uncommon event in liver transplantation, and scintigraphy allows the assessment of perfusion noninvasively (Williams et al, 1985). Preservation injury or peritransplantation infarction is demonstrable on scintigraphy and may give the clinician a clue for the level of function expected from the graft (Karademir et al, 1994), but such information is unlikely to change posttransplant treatment. Cholestasis and rejection are two common posttransplantation problems, and the two overlap in terms of their scintigraphic appearance (Engeler et al, 1992). This problem had led to many units not including scintigraphy in the postoperative assessment. Attempts to further analyze the information within the hepatobiliary scan to assess transplant viability more accurately have been promoted by some authors but have not become widely used (Brown et al, 1988; Shah et al, 1995; Tagge et al, 1987). A normal study effectively excludes rejection or severe cholestasis, but this information is unlikely to be additive to other simpler tests. Nuclear medicine studies are useful in the assessment of immunosuppressed patients with pyrexia of unknown origin.
Pediatric Imaging
Neonatal Jaundice
The task of the physician faced with a newborn who is jaundiced is to determine the cause to decide whether intervention is required. Most cases of neonatal jaundice are due to physiologic causes, breast milk jaundice, and, less commonly, erythroblastosis fetalis. Idiopathic neonatal hepatitis is rare, as is biliary atresia (see Chapter 40). Both of these conditions result in either mixed or conjugated prolonged hyperbilirubinemia (direct bilirubin of >15%). Early diagnosis of biliary atresia is important, because it leads to the development of cirrhosis and liver failure with an average survival of 12 months if untreated (Emblem et al, 1994). The differentiation of neonatal hepatitis and biliary atresia is difficult on clinical grounds and occasionally at liver biopsy (Manolaki et al, 1983).
Biliary Atresia (See Chapter 40)
Differentiation of biliary atresia and neonatal hepatitis on histopathology depends on the presence of ductal proliferation and portal fibrosis, both of which may take some time to develop; this may reduce the accuracy of a biopsy performed too soon after birth. The diagnosis of biliary atresia using hepatobiliary scintigraphy is based primarily on nonvisualization of the gut at 24 hours, but a secondary criterion of rapid uptake of the tracer into the liver without biliary excretion also suggests the presence of biliary atresia. Idiopathic neonatal hepatitis is characterized by impaired uptake of the tracer by the liver with slow hepatic clearance of the tracer, which occurs in parallel to cardiac blood pool, owing to renal excretion (this finding is mimicked by biliary atresia as cirrhosis develops, leading to impaired hepatocyte extraction of the tracer). It is advisable to perform hepatobiliary imaging before 3 months of age to reduce the likelihood of the development of cirrhosis. Premedication with phenobarbital (5 mg/kg/day for 5 days) leads to increased bile flow and improved specificity of the test, as it reduces the number of cases in which the gut is not visualized. The differential diagnosis of nonvisualization of the gut at 24 hours includes biliary atresia, bile plug syndrome, cystic fibrosis, or severe hepatocellular dysfunction secondary to idiopathic neonatal hepatitis, total parenteral nutrition (Cooper et al, 1985), infectious hepatitis, and septooptic dysplasia (Kumura et al, 1987). Bile plug syndrome is seen in dehydrated, septic infants. The characteristic scintigraphic features of this syndrome are excretion of the tracer into a dilated proximal biliary tract without gut excretion.
The diagnosis of biliary atresia on US is based on numerous features, including inability to see a gallbladder, a hypoplastic gallbladder, no change in gallbladder size with food, and a new feature known as the triangular cord sign. Recently reported in many publications by a single group of researchers, the triangular cord sign appears to have high sensitivity, specificity, and accuracy (85%, 100%, and 95%, respectively) (Choi et al, 1996, 1998; Park et al, 1997). The reported efficacy of diisopropylacetanilido-iminodiacetic acid scanning (sensitivity 96%, specificity 35%, accuracy 56%) by this group is less than reported in most other literature; however, using these criteria alone, US sensitivity of 85% translates into 15% of infants with biliary atresia having a delayed surgical procedure; a combined approach would therefore seem appropriate as the authors suggest. US is a good initial test, and it is useful in ruling out the presence of a choledochal cyst, which also may manifest with neonatal jaundice. Hepatobiliary scintigraphy in such a case is useful, if it shows filling of the cyst with bile (Fig. 15.33).
Lin and colleagues (1997) directly compared US with hepatobiliary scintigraphy using premedication with phenobarbital and cholestyramine premedication in a group of 66 patients with neonatal jaundice. The diagnostic sensitivity, specificity, and accuracy of scintigraphy in differentiating biliary atresia from other forms of neonatal jaundice were 100%, 87.5%, and 90.5%, respectively, compared with US results of 86.7%, 77.1%, and 79.4%, respectively (Lin et al, 1997). Premedication with oral phenobarbital increases test accuracy by accelerating biliary excretion (Majd et al, 1981a, 1981b). Reduced specificity of hepatobiliary scintigraphy is seen if the infant’s birth weight is less than 2,200 g (Spivak et al, 1987). Hepatobiliary scintigraphy is the diagnostic test of choice in the diagnosis of cystic duct obstruction; it predicts response to cholecystectomy in patients with acalculous biliary pain and plays a vital role in the assessment of patients with biliary tract injury and neonatal jaundice.
Role Of General Nuclear Medicine In Assessment Of Adults With Malignant Involvement Of The Liver And Hepatobiliary Tree
Cardiac Preoperative Risk Assessment (See Chapter 22)
It is prudent to perform preoperative testing to attempt to risk-stratify patients before major surgery when risk of coronary disease is significant. Patients who have been recommended to undergo preoperative testing include those with two or more of the following: diabetes, prior congestive cardiac failure, prior myocardial infarction, Canadian class 1 or 2 angina, poor functional capacity (<4 metabolic equivalents on a treadmill exercise test), or a planned prolonged surgical procedure with large fluid shifts, blood loss, or both (Leppo & Dahlberg, 1998). Preoperative testing with myocardial perfusion scintigraphy with either thallium-201 (201Tl)- or 99mTc-based perfusion agents is a powerful predictor of perioperative events. The initial reports of thallium scintigraphy showed a high positive predictive value of the test on the order of 20%, but the positive predictive value has declined slowly over time (Leppo & Dahlberg, 1998). In part, this decline may be due to changes in scanning protocols (SPECT versus planar acquisition) or to physicians overreading the scans to avoid possible litigation, as the biology of the disease is unlikely to have changed significantly. A negative test remains highly predictive (>99%) of a cardiac event–free perioperative period.
Sulfur Colloid Imaging
Historical Background
In a normal patient, approximately 90% of the injected dose of 99mTc–sulfur colloid is taken up by the liver, with the spleen and bone marrow each accounting for 5% (Fig. 15.34). Sulfur colloid imaging has been used in the assessment of diffuse parenchymal disease of the liver, with ratios of liver to spleen and bone marrow, the colloid shift, being used to assess the degree of hepatocellular dysfunction. The finding of a hypertrophied caudate lobe raises strong suspicions of the presence of Budd-Chiari syndrome. The advent of high-speed CT and color Doppler US has reduced the demand for liver and spleen imaging for the establishment of this diagnosis.
Diagnosis of Malignancy
The typical appearance of a malignant deposit is a focal cold defect on 99mTc–sulfur colloid imaging, because metastases do not contain the phagocytic cells necessary for uptake of the radiopharmaceutical. “Cold spots” on colloid imaging are nonspecific findings; hepatic cysts, hemangioma, and hepatic adenoma all may appear cold, because they do not contain Kupffer cells (Table 15.5). A solid lesion identified on cross-sectional imaging that is not accompanied by a photopenic defect on sulfur colloid imaging is likely to be FNH (Sandler et al, 1980) or the rare hepatoblastoma, usually only seen in children (Tanasescu et al, 1991). Hepatic adenomas that take up sulfur colloid are quite rare (Low & Khangure, 1990) and usually are gallium avid.
Table 15.5 Cold Defects and Hot Lesions on Technetium 99m–Sulfur Colloid Imaging
Lesion | Explanation |
---|---|
Cold Defects | |
Hepatic pseudotumor | Hepatic regeneration or scar in cirrhotic patients, may or may not be hot |
Acute radiation injury | Radiosensitive reticuloendothelial cells |
Extrahepatic masses | Deformation of normal liver |
Simple cysts | No target cells in lesion |
Polycystic liver disease | No target cells in lesion |
Hemangioma | No target cells in lesion |
Adenoma | Usually cold; rare cases with uptake present have been reported |
Hamartoma | No target cells in lesion |
Hydatid cyst | No target cells in lesion |
FNH | Variably hot or cold because it may contain reticuloendothelial cells |
Hot Lesions | |
Budd-Chiari syndrome | Typically caudate lobe hypertrophy |
Cirrhosis | |
Hepatoma | |
Hemangioma | |
Hamartoma | |
Abscess | |
FNH |
FNH, focal nodular hyperplasia
Dual-Tracer Studies
The relatively low specificity of sulfur colloid imaging led to the development of a variety of dual-tracer studies involving gallium 67 (67Ga) citrate (Beihn et al, 1974; Rovekamp et al, 1983), 99mTc-labeled red blood cells, 111In-labeled white blood cells (Fawcett et al, 1985), and more recently 201Tl (Mochizuki et al, 1994). The principle of the dual-tracer studies was to use tracers of known physiologic processes to add specificity to the colloid findings. 201Tl acts as a potassium analogue when injected intravenously, and it accumulates in viable cells. It has been used for many years for myocardial scintigraphy, and after serendipitous uptake in a tumor, it was investigated as a tumor-seeking agent. Subtraction imaging with 201Tl-labeled and 99mTc-labeled colloids has been used in the assessment of HCC (Mochizuki et al, 1994). 67Ga citrate is a nonspecific tumor-seeking agent whose efficacy probably is related to shared metabolic pathways with iron. A lesion negative on colloidal imaging but positive on labeled white blood cell scan suggests abscess; a lesion positive on 67Ga and negative with colloid imaging is more likely to be a hepatoma (given the absence of fever and other signs of infection). Serial 67Ga studies can be a useful follow-up tool in patients after resection of HCC (Serafini et al, 1988). The improvements in the image achievable with US, CT, and MRI and the limits of resolution (1.5 to 2 cm) of the tracer techniques have led to the gradual replacement of colloid imaging with conventional radiologic investigations. If there are contraindications to biopsy or equivocal lesions, combined tracer studies remain a useful noninvasive technique.
Hemangioma Studies (See Chapter 79A)
A hepatic hemangioma is almost always negative on sulfur colloid imaging (Fig. 15.35). If a lesion suspicious for a hemangioma can be identified as a cold spot with sulfur colloid, a red blood cell study can add weight to the diagnosis of hemangioma. A red blood cell study involves the labeling of the patient’s red blood cells with 99mTc using a stannous chloride chelate, which bonds the 99mTc to the red blood cells (Royal et al, 1981). Because hemangiomas have an increased blood volume compared with normal tissues, they appear as a hot spot on a blood pool scan and may be visualized when other imaging modalities are negative (Krausz et al, 1997). Lesions 1.5 to 2 cm can be reliably detected with sensitivities of 89% (Guze & Hawkins, 1989; Siegel & Mazurek, 1997; Ziessman et al, 1991). Hypervascular metastases do occur but are rare (Farlow et al, 1993; Ginsberg et al, 1986; Shih et al, 1996; Swayne et al, 1991).
Neuroendocrine Receptor Imaging (See Chapters 61 and 81B)
Many neuroendorine tumors can be imaged using 111In-labeled octreotide, a somatostatin analogue, including carcinoids, gastrinomas, insulinomas, glucagonomas, medullary carcinoma of the thyroid, meningiomas, small cell lung cancer, and other tumors such as Hodgkin and non-Hodgkin lymphomas (Krenning et al, 1992). These agents can be used to characterize liver lesions in patients with the aforementioned underlying conditions. Uptake in the normal liver may obscure small lesions, so unless a lesion can be seen as a cold spot or a hot spot, there is limited diagnostic information in a scan showing homogeneous liver uptake of octreotide. The real advantage of somatostatin receptor imaging is in the demonstration of extrahepatic metastases that may not have been shown with the conventional imaging modalities, altering the stage of the patient. Most patients with neuroendocrine tumors should have an octreotide scan before an attempted curative resection for metastatic disease (Fig. 15.36). If available, 68Ga somatostatin receptor imaging should be performed in place of 111In octreotide because of the time savings to the patient and the improved imaging characteristics of the PET agent over the γ-camera agent (Fig. 15.37).
Hepatic Arterial Perfusion Studies (See Chapter 86)
Technetium-99m Macroaggregated Albumin
The radiopharmaceutical 99mTc–macroaggregated albumin (MAA) is available in kit form and is commonly used for pulmonary perfusion scintigraphy. A heating process makes an aggregate of the albumin, resulting in MAA particles, 90% of which are between 10 and 90 µm in diameter. The net effect is that this pharmaceutical embolizes into the first capillary bed it meets and does not pass on through the remainder of the circulation when injected. This preparation has been used to assess the perfusion of the liver by direct injection into the hepatic artery (Yang et al, 1982). The addition of 99mTc–sulfur colloid imaging provides information regarding the relative perfusion of the liver via the hepatic artery as assessed by 99mTc–MAA and the intrahepatic distribution of the hepatic metastases as shown by 99mTc–sulfur colloid imaging (Figs. 15.38 to 15.41; Ridge et al, 1987). Direct injection of chemotherapeutic agents into the hepatic artery has been shown to deliver 10 times the concentration of 5-fluorouracil (5-FU) into liver metastases than delivery via the intravenous route (Hohenberger et al, 1993).
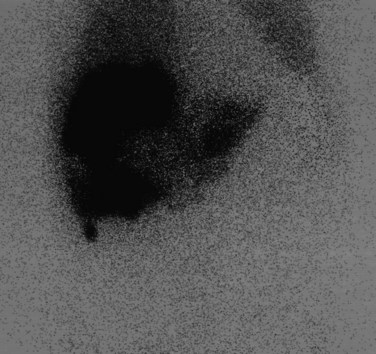
FIGURE 15.41 Same patient as in Figure 15.40. The window and level of display are “wound up,” showing hepatopulmonary shunting.
The advent of intrahepatic arterial drug therapy has led to increasing use of combined 99mTc–MAA and 99mTc–sulfur colloid imaging to exclude extrahepatic delivery of the radiopharmaceutical, which may necessitate catheter revision (Habbe et al, 1998). Inadvertent direct perfusion of the stomach, small intestine, or pancreas can cause local chemotoxicity, leading to localized inflammation that is often symptomatic (Figs. 15.42 and 15.43; Choplin et al, 1983; Chuang et al, 1981; Ku et al, 1998; Ottery et al, 1986). In some cases, the distribution of the tracer on scintigraphy has predicted the pattern of response of the metastases to the therapy (Borzutzky & Turbiner, 1985) and is a useful adjunct in the assessment of response to therapy (Savolaine et al, 1989). Hepatic artery scintigraphy also has been used to show the presence of arteriovenous shunting in hepatic tumors, which can modify therapy in an attempt to limit pulmonary toxicity (see Fig. 15.41; Leung et al, 1994).
Hepatic Arterial Therapy using Radiopharmaceuticals (See Chapter 84A)
An adaptation of the chemotherapy approach has been made by many groups, using therapeutic doses of unsealed radiotherapy. MAA imaging is also integral to the workup of a patient being considered for hepatic arterial embolization with radioactive microspheres, another approach to the palliation of liver tumors that share similar toxicity profiles when administered inaccurately. Hepatic delivery of β-particle–emitting radiopharmaceuticals, such as 90Yt- or 131In-labeled microspheres, has been associated with improved median survival and reductions in tumor markers (Lau et al, 1994).
Akhurst T, et al, 1999: Fluorodeoxyglucose (FDG) positron emission tomography (PET) immediately post hepatic cryotherapy predicts recurrence of tumor in the liver. Presented at the annual meeting of the American Society of Clinical Oncology, May 15-18, 1999, Atlanta, Ga.
Barron LG, Rubio PA. Importance of accurate preoperative diagnosis and role of advanced laparoscopic cholecystectomy in relieving chronic acalculous cholecystitis. J Laparoendosc Surg. 1995;5:357-361.
Bassi C, et al. Management of 100 consecutive cases of pancreatic serous cystadenoma: wait for symptoms and see at imaging or vice versa? World J Surg. 2003;27:319-323.
Beihn RM, et al. Subtraction technique for the detection of subphrenic abscesses using 67Ga and 99mTc. J Nucl Med. 1974;15:371-373.
Berr F, et al. Detection of cholangiocarcinoma in primary sclerosing cholangitis by positron emission tomography. Hepatology. 1999;29:611-613. (letter)
Beyer T, et al. Dual-modality PET/CT tomography for clinical oncology. Q J Nucl Med. 2000;46:24-34.
Beyer T, et al. Dual-modality PET/CT imaging: the effect of respiratory motion on combined image quality in clinical oncology. Eur J Nucl Med Mol Imaging. 2003;30:588-596.
Borzutzky CA, Turbiner EH. The predictive value of hepatic artery perfusion scintigraphy. J Nucl Med. 1985;26:1153-1156.
Brown PH, et al. Hepatocyte versus biliary disease: a distinction by deconvolutional analysis of technetium-99m IDA time–activity curves. J Nucl Med. 1988;29:623-630.
Cabana MD, et al. Morphine-augmented hepatobiliary scintigraphy: a meta-analysis. Nucl Med Commun. 1995;16:1068-1071.
Cawthon MA, et al. Biliary scintigraphy: the “hot rim” sign. Clin Nucl Med. 1984;9:619-621.
Chen CC, et al. Morphine augmentation increases gallbladder visualization in patients pretreated with cholecystokinin. J Nucl Med. 1997;38:644-647.
Choi SO, et al. “Triangular cord”: a sonographic finding applicable in the diagnosis of biliary atresia. J Pediatr Surg. 1996;31:363-366.
Choi SO, et al. Ultrasonographic “triangular cord”: the most definitive finding for noninvasive diagnosis of extrahepatic biliary atresia. Eur J Pediatr Surg. 1998;8:12-16.
Choplin RH, et al. Gastric perforation from hepatic artery infusion chemotherapy. Gastrointest Radiol. 1983;8:133-134.
Choy D, et al. Cholescintigraphy in acute cholecystitis: use of intravenous morphine. Radiology. 1984;151:203-207.
Chuang VP, et al. Hepatic artery infusion chemotherapy: gastroduodenal complications. Am J Roentgenol. 1981;137:347-350.
Comar D, et al. Labelling and metabolism of methionine-methyl-11 C. Eur J Nucl Med. 1976;1:11-14.
Cooper A, et al. Resolution of intractable cholestasis associated with total parenteral nutrition following biliary irrigation. J Pediatr Surg. 1985;20:772-774.
Corvera C, et al. F-18-FDG PET in the evaluation of patients with gallbladder cancer. Gastroenterology. 2004;126:A786.
Delbeke D, et al. Staging recurrent metastatic colorectal carcinoma with PET. J Nucl Med. 1997;38:1196-1201.
Delbeke D, et al. Evaluation of benign vs malignant hepatic lesions with positron emission tomography. Arch Surg. 1998;133:510-516.
Dykes EH, et al. The role of 99mTc HIDA cholescintigraphy in the diagnosis of acute gallbladder disease: comparison with oral cholecystography and ultrasonography. Scott Med J. 1986;31:170-173.
Eikman EA, et al. A test for patency of the cystic duct in acute cholecystitis. Ann Intern Med. 1975;82:318-322.
Emblem R, et al. [Better results with early surgical intervention in biliary atresia: icterus in infants older than 14 days should be investigated]. Tidsskr Nor Laegeforen. 1994;114:1946-1947.
Engeler CM, et al. Liver transplant rejection and cholestasis: comparison of technetium 99m-diisopropyl iminodiacetic acid hepatobiliary imaging with liver biopsy. Eur J Nucl Med. 1992;19:865-870.
Enomoto K, et al. [Can fluorodeoxyglucose positron-emission tomography evaluate the functional differentiation of hepatocellular carcinoma]. Kaku Igaku. 1991;28:1353-1356.
Farlow DC, et al. A case of metastatic malignancy masquerading as a hepatic hemangioma on labeled red blood cell scintigraphy. J Nucl Med. 1993;34:1172-1174.
Fawcett HD, et al. Indium-111 chloride for detecting suspected hepatomas in patients with focal defects on technetium-99m sulfur colloid liver imaging. Clin Nucl Med. 1985;10:410-412.
Fernandez-del Castillo C, et al. Incidental pancreatic cysts: clinicopathologic characteristics and comparison with symptomatic patients. Arch Surg. 2003;138:427-433.
Fink-Bennett D, et al. Morphine-augmented cholescintigraphy: its efficacy in detecting acute cholecystitis. J Nucl Med. 1991;32:1231-1233.
Fink-Bennett D, et al. Cholecystokinin cholescintigraphy: detection of abnormal gallbladder motor function in patients with chronic acalculous gallbladder disease. J Nucl Med. 1991;32:1695-1699.
Flamen P, et al. Additional value of whole-body positron emission tomography with fluorine-18-2-fluoro-2-deoxy-D-glucose in recurrent colorectal cancer. J Clin Oncol. 1999;17:894-901.
Freitas JE, et al. Suspected acute cholecystitis: comparison of hepatobiliary scintigraphy versus ultrasonography. Clin Nucl Med. 1982;7:364-367.
Ginsberg F, et al. Hepatic angiosarcoma: mimicking of angioma on three-phase technetium-99m red blood cell scintigraphy. J Nucl Med. 1986;27:1861-1863.
Goncalves RM, et al. Biliary dyskinesia: natural history and surgical results. Am Surg. 1998;64:493-498.
Guze BH, Hawkins RA. Utility of the SPECT Tc-99m labeled RBC blood pool scan in the detection of hepatic hemangiomas. Clin Nucl Med. 1989;14:817-818.
Habbe TG, et al. Complications and technical limitations of hepatic arterial infusion catheter placement for chemotherapy. J Vasc Interv Radiol. 1998;9:233-239.
Hellwig D, et al. Radioiodinated phenylalanine derivatives to image pancreatic cancer: a comparative study with [18F]fluoro-2-deoxy-D-glucose in human pancreatic carcinoma xenografts and in inflammation models. Nucl Med Biol. 2005;32:137-145.
Helm JF, et al. Effects of morphine on the human sphincter of Oddi. Gut. 1988;29:1402-1407.
Hillner BE, et al. Impact of positron emission tomography/computed tomography and positron emission tomography (PET) alone on expected management of patients with cancer: initial results from the National Oncologic PET Registry. J Clin Oncol. 2008;26:2155-2161.
Hohenberger P, et al. Perfusion of colorectal liver metastases and uptake of fluorouracil assessed by H2(15)O and [18F]uracil positron emission tomography (PET). Eur J Cancer. 1993;29A:1682-1686.
Hubble D, et al. 177)Lu-octreotate, alone or with radiosensitising chemotherapy, is safe in neuroendocrine tumour patients previously treated with high-activity (111)In-octreotide. Eur J Nucl Med Mol Imaging. 2010;37:1869-1875.
Hui AM, et al. Cell cycle regulators and human hepatocarcinogenesis. Hepatogastroenterology. 1998;45:1635-1642.
Jarnagin WR, et al. Liver resection for metastatic colorectal cancer: assessing the risk of occult irresectable disease. J Am Coll Surg. 1999;188:33-42.
Johanning JM, Gruenberg JC. The changing face of cholecystectomy. Am Surg. 1998;64:643-648.
Karademir S, et al. Role of 99mTc-labeled DISIDA scan in the assessment of marginal liver grafts after orthotopic transplantation. Clin Transplant. 1994;8:54-58.
Keiding S, et al. Detection of cholangiocarcinoma in primary sclerosing cholangitis by positron emission tomography. Hepatology. 1998;28:700-706.
Kim CK, et al. Cholescintigraphy in the diagnosis of acute cholecystitis: morphine augmentation is superior to delayed imaging. J Nucl Med. 1993;34:1866-1870.
Klingensmith WC, et al. The normal fasting and postprandial diisopropyl-IDA Tc-99m hepatobiliary study. Radiology. 1981;141:771-776.
Krausz Y, et al. Liver hemangioma: a perioperative Tc-99m RBC SPECT correlation. Clin Nucl Med. 1997;22:35-37.
Krenning EP, et al. 111In-octreotide scintigraphy in oncology. Metabolism. 1992;41:83-86.
Ku Y, et al. Percutaneous isolated liver chemoperfusion for treatment of unresectable malignant liver tumors: technique, pharmacokinetics, clinical results. Recent Results Cancer Res. 1998;147:67-82.
Kumura D, et al. Septo-optic dysplasia: recognition of causes of false-positive hepatobiliary scintigraphy in neonatal jaundice. J Nucl Med. 1987;28:966-972.
Kwekkeboom DJ, et al. Treatment with the radiolabeled somatostatin analog [177 Lu-DOTA 0,Tyr3] octreotate: toxicity, efficacy, and survival. J Clin Oncol. 2008;26:2124-2130.
Lai DT, et al. The role of whole-body positron emission tomography with [18F]fluorodeoxyglucose in identifying operable colorectal cancer metastases to the liver. Arch Surg. 1996;131:703-707.
Larsen MJ, et al. Radionuclide hepatobiliary imaging: nonvisualization of the gallbladder secondary to prolonged fasting. J Nucl Med. 1982;23:1003-1005.
Lau WY, et al. Treatment of inoperable hepatocellular carcinoma with intrahepatic arterial yttrium-90 microspheres: a phase I and II study. Br J Cancer. 1994;70:994-999.
Lauritsen KB, et al. Cholescintigraphy and ultrasonography in patients suspected of having acute cholecystitis. Scand J Gastroenterol. 1988;23:42-46.
Leppo JA, Dahlberg ST. The question: to test or not to test in preoperative cardiac risk evaluation? J Nucl Cardiol. 1998;5:332-342.
Leung WT, et al. Measuring lung shunting in hepatocellular carcinoma with intrahepatic-arterial technetium-99m macroaggregated albumin. J Nucl Med. 1994;35:70-73.
Lin WY, et al. Comparison of technetium Tc-99m disofenin cholescintigraphy with ultrasonography in the differentiation of biliary atresia from other forms of neonatal jaundice. Pediatr Surg Int. 1997;12:30-33.
Low V, Khangure MS. Hepatic adenoma and focal nodular hyperplasia: a diagnostic dilemma. Australas Radiol. 1990;34:124-130.
Madacsy L, et al. Differentiation between organic stenosis and functional dyskinesia of the sphincter of Oddi with amyl nitrite–augmented quantitative hepatobiliary scintigraphy. Eur J Nucl Med. 1994;21:203-208.
Madacsy L, et al. Evaluation of results of the prostigmine-morphine test with quantitative hepatobiliary scintigraphy: a new method for the diagnosis of sphincter of Oddi dyskinesia. Eur J Nucl Med. 1995;22:227-232.
Majd M, et al. Effect of phenobarbital on 99mTc-IDA scintigraphy in the evaluation of neonatal jaundice. Semin Nucl Med. 1981;11:194-204.
Majd M, et al. Hepatobiliary scintigraphy with 99mTc-PIPIDA in the evaluation of neonatal jaundice. Pediatrics. 1981;67:140-145.
Maldonado ME, et al. Incidence of pancreatitis in patients undergoing sphincter of Oddi manometry (SOM). Am J Gastroenterol. 1999;94:387-390.
Manolaki AG, et al. The prelaparotomy diagnosis of extrahepatic biliary atresia. Arch Dis Child. 1983;58:591-594.
Mochizuki T, et al. Detection of complications after liver transplantation by technetium-99m mebrofenin hepatobiliary scintigraphy. Ann Nucl Med. 1991;5:103-107.
Mochizuki T, et al. Thallium-201/technetium-99m-phytate (colloid) subtraction imaging of hepatocellular carcinoma. J Nucl Med. 1994;35:1134-1137.
Moore A, et al. Noninvasive in vivo measurement of beta-cell mass in mouse model of diabetes. Diabetes. 2001;50:2231-2236.
Oates E, et al. Gallbladder nonvisualization with pericholecystic rim sign: morphine augmentation optimizes diagnosis of acute cholecystitis. J Nucl Med. 1996;37:267-269.
Ogunbiyi OA, et al. Detection of recurrent and metastatic colorectal cancer: comparison of positron emission tomography and computed tomography. Ann Surg Oncol. 1997;4:613-620.
Ottery FD, et al. Chemical cholecystitis after intrahepatic chemotherapy: the case for prophylactic cholecystectomy during pump placement. Dis Colon Rectum. 1986;29:187-190.
Park WH, et al. A new diagnostic approach to biliary atresia with emphasis on the ultrasonographic triangular cord sign: comparison of ultrasonography, hepatobiliary scintigraphy, and liver needle biopsy in the evaluation of infantile cholestasis. J Pediatr Surg. 1997;32:1555-1559.
Patch GG, et al. Naloxone reverses pattern of obstruction of the distal common bile duct induced by analgesic narcotics in hepatobiliary imaging. J Nucl Med. 1991;32:1270-1272.
Reed DNJr, et al. Kinevac-assisted cholescintigraphy as an accurate predictor of chronic acalculous gallbladder disease and the likelihood of symptom relief with cholecystectomy. Am Surg. 1993;59:273-277.
Reichelt HG. [Hepatobiliary sequential scintigraphy: value of this nuclear-medicine diagnostic technic in the preoperative and postoperative stages following hepato-biliary tract injuries]. Chirurg. 1978;49:167-171.
Ridge JA, et al. Perfusion of colorectal hepatic metastases: relative distribution of flow from the hepatic artery and portal vein. Cancer. 1987;59:1547-1553.
Roberts-Thomson IC, et al. Assessment of bile flow by radioscintigraphy in patients with biliary-type pain after cholecystectomy. Aust N Z J Med. 1986;16:788-793.
Rovekamp MH, et al. Diagnosis of upper-abdominal infections by In-111 labeled leukocytes with Tc-99m colloid subtraction technique. J Nucl Med. 1983;24:212-216.
Royal HD, et al. Scintigraphy of hepatic hemangiomas: the value of Tc-99m–labeled red blood cells: concise communication. J Nucl Med. 1981;22:684-687.
Samuels BI, et al. A comparison of radionuclide hepatobiliary imaging and real-time ultrasound for the detection of acute cholecystitis. Radiology. 1983;147:207-210.
Sandler MA, et al. Ultrasonic features and radionuclide correlation in liver cell adenoma and focal nodular hyperplasia. Radiology. 1980;135:393-397.
Savolaine ER, et al. Role of scintigraphy in establishing optimal perfusion in hepatic arterial infusion pump chemotherapy. Am J Clin Oncol. 1989;12:68-74.
Scheele J, et al. Resection of colorectal liver metastases. World J Surg. 1995;19:59-71.
Schiepers C, et al. Contribution of PET in the diagnosis of recurrent colorectal cancer: comparison with conventional imaging. Eur J Surg Oncol. 1995;21:517-522.
Schroder O, et al. Limited value of fluorine-18-fluorodeoxyglucose PET for the differential diagnosis of focal liver lesions in patients with chronic hepatitis C virus infection. Nuklearmedizin. 1998;37:279-285.
Serafini AN, et al. Early recognition of recurrent hepatocellular carcinoma utilizing gallium-67 citrate scintigraphy. J Nucl Med. 1988;29:712-716.
Shah AN, et al. Role of nuclear medicine in liver transplantation. Semin Nucl Med. 1995;25:36-48.
Shea JA, et al. Revised estimates of diagnostic test sensitivity and specificity in suspected biliary tract disease. Arch Intern Med. 1994;154:2573-2581.
Shih WJ, et al. False-positive results for hepatic hemangioma on Tc-99m RBC SPECT caused by a liver metastasis from small-cell lung carcinoma. Clin Nucl Med. 1996;21:898-899.
Siegel A, Mazurek R. Early dynamic SPECT acquisition for the imaging of hepatic hemangiomas utilizing Tc-99m labeled red blood cells. Clin Nucl Med. 1997;22:745-748.
Silberstein EB. Prevalence of adverse reactions to positron-emitting radiopharmaceuticals in nuclear medicine. Pharmacopeia Committee of the Society of Nuclear Medicine. J Nucl Med. 1998;39:2190-2192.
Soiva M, et al. The value of routine sonography in clinically suspected acute cholecystitis. Scand J Gastroenterol. 1986;21:70-74.
Sostre S, et al. Gallbladder response to a second dose of cholecystokinin during the same imaging study. Eur J Nucl Med. 1992;19:964-965.
Sostre S, et al. A noninvasive test of sphincter of Oddi dysfunction in postcholecystectomy patients: the scintigraphic score. J Nucl Med. 1992;33:1216-1222.
Spivak W, et al. Diagnostic utility of hepatobiliary scintigraphy with 99mTc-DISIDA in neonatal cholestasis. J Pediatr. 1987;110:855-861.
Staritz M. Pharmacology of the sphincter of Oddi. Endoscopy. 1988;20(Suppl 1):171-174.
Suarez CA, et al. The role of HIDA/PIPIDA scanning in diagnosing cystic duct obstruction. Ann Surg. 1980;191:391-396.
Swayne LC, et al. False-positive hepatic blood pool scintigraphy in metastatic colon carcinoma. Clin Nucl Med. 1991;16:630-632.
Sweet IR, et al. Non-invasive imaging of beta cell mass: a quantitative analysis. Diabetes Technol Ther. 2004;5:652-659.
Szlabick RE, et al. Hepatobiliary scanning in the diagnosis of acute cholecystitis. Arch Surg. 1980;115:540-544.
Tagge EP, et al. Quantitative scintigraphy with deconvolutional analysis for the dynamic measurement of hepatic function. J Surg Res. 1987;42:605-612.
Takasu A, et al. [11C]methionine positron emission tomography for the evaluation of pancreatic exocrine function in chronic pancreatitis. Pancreas. 2001;22:203-209.
Tanasescu DE, et al. Scintigraphic findings mimicking focal nodular hyperplasia in a case of hepatoblastoma. Clin Nucl Med. 1991;16:236-238.
Torizuka T, et al. Value of fluorine-18-FDG-PET to monitor hepatocellular carcinoma after interventional therapy. J Nucl Med. 1994;35:1965-1969.
Torizuka T, et al. In vivo assessment of glucose metabolism in hepatocellular carcinoma with FDG–PET. J Nucl Med. 1995;36:1811-1817.
Valk PE, et al. Detection of recurrent colorectal cancer by FDG PET in patients with serum CEA elevation. J Nucl Med. 1998;39:135P.
Valls C, et al. Helical CT versus CT arterial portography in the detection of hepatic metastasis of colorectal carcinoma. Am J Roentgenol. 1998;170:1341-1347.
van Weelde BJ, et al. Ultrasonography of acute cholecystitis: clinical and histological correlation. Diagn Imaging Clin Med. 1986;55:190-195.
Velasco J, et al. Hepatobiliary scanning in cholecystitis. Eur J Nucl Med. 1982;7:11-13.
Vitola JV, et al. Positron emission tomography with F-18-fluorodeoxyglucose to evaluate the results of hepatic chemoembolization. Cancer. 1996;78:2216-2222.
Vitola JV, et al. Positron emission tomography to stage suspected metastatic colorectal carcinoma to the liver. Am J Surg. 1996;171:21-26.
Walker AT, et al. Bile duct disruption and biloma after laparoscopic cholecystectomy: imaging evaluation. Am J Roentgenol. 1992;158:785-789.
Walker AT, et al. Bile duct disruption after laparoscopic cholecystectomy. Semin Ultrasound CT MR. 1993;14:346-355.
Warburg O. Metabolism of Tumors. London: Constable; 1930.
Williams HC, et al. Vascular thrombosis in acute hepatic allograft rejection: scintigraphic appearance. J Nucl Med. 1985;26:478-481.
Yang PJ, et al. Perfusion scintigraphy (Tc-99m MAA) during surgery for placement of chemotherapy catheter in hepatic artery: concise communication. J Nucl Med. 1982;23:1066-1069.
Yonekura Y, et al. Increased accumulation of 2-deoxy-2-[18F]Fluoro-D-glucose in liver metastases from colon carcinoma. J Nucl Med. 1982;23:1133-1137.
Yoon DS, et al. Cell proliferation index and the expression of p53 and Bcl-2 in tumorous and non-tumorous lesions of hepatocellular carcinoma and metastatic liver cancer. Yonsei Med J. 1998;39:424-429.
Ziessman HA, et al. Improved detection of small cavernous hemangiomas of the liver with high-resolution three-headed SPECT. J Nucl Med. 1991;32:2086-2091.
Zimny M, et al. Fluorine-18 fluorodeoxyglucose positron emission tomography in the differential diagnosis of pancreatic carcinoma: a report of 106 cases. Eur J Nucl Med. 1997;24:678-682.