CHAPTER 10 Nonsteroidal Antiinflammatory Drugs
INTRODUCTION
The effective management of acute postoperative pain is important for both humanitarian and psychological reasons. Despite the advances in analgesic agents and delivery systems over recent years, postoperative pain is often undertreated.1,2
The undertreatment of acute postoperative pain has important implications for both patients and the healthcare system. Undertreated postoperative pain prevents sleep, which causes fatigue and delays mobilization.3 It prolongs patient suffering and is associated with a number of undesirable outcomes such as delayed healing, extended hospitalization, and the development of chronic pain conditions and depressive disorders.4–6 Its overall negative effect on patient function increases healthcare utilization and costs.7
Multimodal analgesia is currently recognized as the best postoperative method to reduce pain and analgesia requirements.8 Reduced pain improves recovery, reduces postoperative morbidity, and lowers costs.9–11 The multimodal approach uses a combination of drugs and delivery techniques. For example, the combination of drugs such as opioids, non-specific nonsteroidal antiinflammatory drugs (NSAIDs), cyclooxygenase-2 (COX-2)-specific inhibitors, and local anesthetics may decrease adverse effects and better optimize pain control. Delivery techniques such as patient-controlled analgesia, epidural blocks, and regional blocks are often better at optimizing pain control with fewer adverse effects than single-dose delivery.
Although opioids and non-specific NSAIDs are effective analgesic agents, their use is limited by their adverse effect profiles. Opioids can cause respiratory depression, nausea, vomiting, constipation, pruritis, urinary retention, hypotension, tolerance, and dependency.12 Non-specific NSAIDs can cause gastrointestinal toxicity, renal problems, platelet dysfunction, and bleeding complications, the latter of which may be particularly problematic in the surgical setting.13–15 The adverse effects of non-specific NSAIDs arise as a result of their inhibitory effect on COX-1.16,17 COX-2-specific inhibitors were developed to provide the beneficial antiinflammatory and analgesic effects of non-specific NSAIDs while sparing the protective functions mediated by COX-1.
EPIDEMIOLOGY AND UNDERTREATMENT OF POSTOPERATIVE PAIN
Postoperative pain is one of the most common types of acute pain. Based on data from the National Hospital Discharge Survey, 42.5 million procedures were performed on hospital inpatients during 2002 in the United States alone.18 The number of outpatient procedures is difficult to quantify, but probably exceeds this number.7 Up to 88% of patients may report postoperative pain, and of those, 34–62% experience moderate to severe postoperative pain.1,2
Although postoperative pain is treatable, over the last 50 years its undertreatment has been well documented in the literature.19 The reasons for this are not completely understood, but are probably fostered by the notion that pain is an inevitable consequence of surgery.2,20 Other reasons may include a lack of formal education in pain management, physicians’ concerns regarding potential opioid addiction and drug tolerance, and difficulties in assessing pain severity.21 Furthermore, patients themselves may fail to request or use analgesia because of fears about adverse effects or dependency.
PRACTICE GUIDELINES FOR PAIN MANAGEMENT IN THE PERIOPERATIVE SETTING
Several organizations have published treatment standards for the management of pain. The World Health Organization has produced guidelines for cancer pain relief,22 and the American Pain Society has developed guidelines for the management of acute and cancer pain (Table 10.1).23 The publication of the Joint Commission on Accreditation of Healthcare Organizations’ pain standards has been instrumental in promoting the increased acceptance of pain as the fifth vital sign.24,25
Table 10.1 American Pain Society Quality Improvement Guidelines for the Management of Acute and Cancer Pain23
Recognize and treat pain promptly |
Chart and display the patient’s self-report of pain |
Commit to continue improvement of one or several outcome variables |
Document outcomes based on data and provide prompt feedback |
Make information about analgesic drugs readily available |
Promise the patient attentive analgesic care |
Define explicit policies for the use of advanced analgesic technologies |
Examine the process and outcomes of pain management with the goal of continuous improvement |
Reprinted from JAMA, vol. 274, American Pain Society Quality of Care Committee, quality improvement guidelines for the treatment of acute pain and cancer pain, pp. 1874–1888, 1995, with permission from the American Medical Association.
PATHOPHYSIOLOGY OF POSTOPERATIVE PAIN
Nociception and inflammatory pain
Nociceptive input is initiated by tissue damage and prolonged by inflammation. In response to tissue injury, specialized peripheral nociceptors promote the release of inflammatory mediators, neurotransmitters, and growth factors from traumatized tissue and sequestrated intravascular cells.26,27 The resulting sensory input is conveyed by visceral and somatic neurons to the dorsal horns and ascending spinal pathways to the thalamic, limbic, and cortical structures involved in affective and sensory-discriminative responses. Although the sensory input is extensively modulated both peripherally and centrally, the end result is the subjective awareness of pain.28
This inflammatory pain is characterized by hypersensitivity to stimuli both at the site of injury (primary hyperalgesia) and at sites distant to the site of injury (secondary hyperalgesia).26,29 While primary hyperalgesia is the result of peripheral pain mechanisms, secondary hyperalgesia involves central modulatory systems, including central sensitization.29 Fortunately, the hypersensitivity caused by inflammation usually normalizes if the disease process is controlled.26
Peripheral and central sensitization
Peripheral sensitization or heterosensitization is caused by exposure of nociceptor terminals to sensitizing agents. Released by damaged tissue or by inflammatory cells, these sensitizing agents include the inflammatory mediators, prostaglandin E2, bradykinin, and serotonin as well as various nerve growth factors.26,30
By activating intracellular signaling cascades, increased peripheral input into the central nervous system increases neuronal synaptic responses and decreases neuronal inhibition. If this amplification of sensory input is prolonged, both nocuous and innocuous neuronal inputs cause activation of spinal cord cells. The result is central sensitization.26,31 Prolonged amplification results in anatomic and neurochemical changes leading to neural plasticity in primary sensory neurons and is categorized as activation, modulation, and modification (Fig. 10.1).26
Prostaglandin production and the expression of COX-1 and COX-2
The synthesis of prostanoids is essential for the generation of inflammatory pain.32 Arachidonic acid is released from phospholipids in the cell membrane by phospholipid A2 enzymes and is converted to prostaglandin G2 and then prostaglandin H2 in a 2-step reaction catalyzed by COX (Fig. 10.2).32 Prostaglandin H2 is converted into the prostaglandin isoforms prostaglandin E2, prostaglandin D2 or prostaglandin F2a, prostacyclin, or thromboxane A2 through the action of tissue-specific isomerases.32,33 Differential expression and induction patterns of these enzymes and prostanoid receptors have important roles in the cellular effects of the prostanoids.
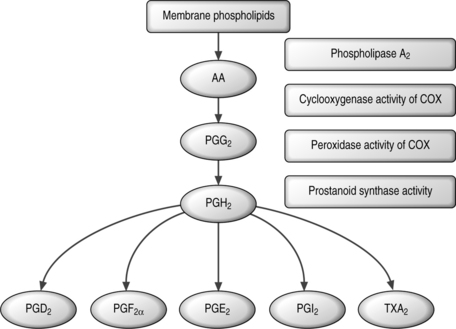
Fig. 10.2 Prostanoid biosynthetic pathway.32
Reprinted from Trends in Molecular Medicine, vol 8, Samad TA, Sapirstein A, and Woolf CJ, Prostanoids and pain: unraveling mechanisms and revealing therapeutic targets, pp. 390–396, 2002, with permission from Elsevier.
COX exists as two isoforms: COX-1, which is responsible for homeostatic prostanoid synthesis, and COX-2, which is responsible for proinflammatory prostanoid production.34 COX-1 is constitutive within platelets and is associated with the production of thromboxane, which strongly promotes platelet aggregation, and both COX-1 and COX-2 are constitutively expressed in the central nervous system, dorsal root ganglia, and kidney.35–38 In addition, COX-2 expression can be induced by several factors, including neurotransmitters and proinflammatory cytokines,39 and the role of inducible COX-2 in central nervous system responses to inflammation is well established. Its expression is upregulated within the central nervous system in response to interleukin-1β, leading to elevations in central prostanoid production.40
Several animal studies have assessed the relative contributions of COX-1 and COX-2 to prostaglandin release in the spinal cord. The selective COX-1 inhibitor SC560 significantly reduced nociceptive behavior and abolished spinal prostaglandin E2 release in a rat model, while celecoxib had no effect on either parameter.41 These findings indicated that nociception-evoked prostaglandin release was primarily caused by COX-1 and was independent of COX-2, and suggested that the efficacy of celecoxib in early injury-evoked pain may be lower than that of non-specific NSAIDs. In contrast, another study in a rat model suggested that COX-2, but not COX-1, mediated antihyperalgesic activity and release of spinal prostaglandin E2.42 In rats with chronic indwelling catheters, acute thermal hyperalgesia evoked by spinal delivery of substance P or N-methyl-D-aspartate and thermal hyperalgesia induced by carrageenan was suppressed by intrathecal and systemic COX-2 inhibitors, while systemic but not spinal administration of a COX-1 inhibitor reduced carrageenan-evoked thermal hyperalgesia, and neither systemic nor spinal COX-1 inhibition had an effect on spinal hyperalgesia evoked by substance P. Intrathecal substance P enhanced prostaglandin E2 release, and this effect was diminished by systemic delivery of non-specific NSAIDs and COX-2-specific inhibitors, but not by a COX-1-specific inhibitor. Therefore, constitutive spinal COX-2, but not COX-1, mediates acute antihyperalgesic effects of both spinal and systemic COX-2 inhibitors.
More recently, inhibition of constitutively expressed spinal COX-2 immediately following peripheral tissue injury has been found to reduce injury-induced activation of primary afferent neurons and spinal neurons and mechanical and thermal hyperalgesia prior to any measurable upregulation of COX-2 protein.36 This early research has suggested that constitutive spinal COX-2 may play a role in the development of hyperalgesia following peripheral tissue injury, and that blockade of this constitutive expression prior to injury may lessen the peripheral and central sensitization occurring after tissue injury. Considering the intrathecal potency of COX-2 inhibitors, the comparable efficacy of intrathecal and systemic COX-2 inhibitors in hyperalgesic states not associated with inflammation, and the onset of antihyperalgesic activity prior to COX-2 upregulation, it has been hypothesized that modulation of constitutive spinal COX-2 is a principal antihyperalgesic mechanism of COX-2 inhibitors.43
Role of COX-2 in peripheral sensitization
Inflammation induces the expression of COX-2, which in turn promotes the release of prostanoids. The resulting sensitization of peripheral nociceptor terminals causes primary hyperalgesia. Secondary hyperalgesia due to increased neuronal excitability in the spinal cord causes central sensitization.26,29
Role of COX-2 in central sensitization
Inflammation induces COX-2 expression in spinal cord neurons and other regions of the central nervous system. This widespread induction causes an 80-fold increase in the levels of prostaglandin E2 in the cerebrospinal fluid.40 The major inducer of COX-2 upregulation is interleukin-1β,44,45 and its role in the regulation of central prostanoid production was confirmed by intraspinal administration of an interleukin-converting enzyme or COX-2-specific inhibitor. Intraspinal injection resulted in decreased inflammation-induced central prostaglandin E2 levels and reduced centrally generated inflammatory pain hypersensitivity.40
These experimental findings were confirmed in a study in 30 patients undergoing thoracotomy for lobectomy who were randomized to receive nimesulide 100 mg b.i.d. or ibuprofen 400 mg t.i.d.46 Cerebrospinal fluid samples were analyzed for 6-keto-prostaglandin F1 α, the principle metabolite of prostacyclin. COX-1 and COX-2 activity was determined by measuring serum thromboxane B2 and endotoxin-induced prostaglandin E2 generation in whole blood. Nimesulide was found to be selective for COX-2, while ibuprofen was COX nonselective. Mean levels of 6-keto-prostaglandin F1 α in the cerebrospinal fluid increased following surgery and were significantly suppressed by nimesulide, but not by ibuprofen. Patients receiving nimesulide also had significantly lower pain scores (p<;0.001), morphine requirement (p=0.0175), and falls in peak expiratory flow rate (p<;0.001). This study demonstrated that increases in spinal prostaglandin synthesis after thoracotomy were repressed by a COX-2-selective agent.
PHARMACOLOGIC AGENTS FOR MANAGEMENT OF POSTOPERATIVE PAIN
Simple analgesics
Simple analgesics such as acetaminophen are a common monotherapy for treating mild to moderate pain. While acetaminophen can effectively treat pain of relatively low intensity, it does not provide any therapeutic benefit to an underlying inflammatory process. Acetaminophen is useful for aspirin-sensitive asthmatics and individuals at risk of gastrointestinal complications in whom non-specific NSAIDs are contraindicated.47 Although acetaminophen is routinely used in combination with opioids such as codeine, tramadol, hydrocodone, and oxycodone to reduce opioid use,48 this combination can result in more adverse effects than acetaminophen alone.
In adults, acetaminophen is administered to a maximum daily dose of 500 mg, although this should be reduced in patients with a history of heavy alcohol intake. Long-term use in combination with alcohol can be complicated by severe and sometimes fatal liver damage.47
Opioids
Opioids are highly effective in the management of severe, acute postoperative pain. The agonist or ‘pure’ morphine-like drugs range in potency from ‘less potent’ agents such as oxycodone, which is suitable for moderate pain, to potent drugs such as fentanyl, which is often used intraoperatively. There are also drugs such as buprenorphine, which possess both agonist and antagonist actions. Although the agonist–antagonist opioids are the least effective opioid type, they have the lowest risk of adverse events and typically show a ceiling effect in both analgesic activity and effect on respiratory depression.6,12 Finally, there are opioid antagonists such a naloxone that are pure antagonists without any analgesic effect.6
Although effective, opioid use is associated with multiple adverse effects, including sedation, hypotension, respiratory depression, emesis, paralytic ileus, urinary retention, pruritis, and dependence.49–51 These effects can complicate and lengthen the postoperative recovery period, and concerns about poor tolerability and perceived dependency can result in inadequate dosing. Controlling the variability in plasma concentration by the use of patient-assisted devices may reduce these adverse effects.
Even though opioids can provide a sustained analgesic effect and improved function in selected, well-monitored patients without the development of tolerance or significant toxicity,12 the use of adjunctive agents in combination with opioids is still often desirable. The synergistic coadministration of other analgesics reduces opioid doses and decreases adverse effects. In addition, opioids are ineffective when used alone for the management of movement-related pain, and supplementation with other types of medication is needed during postoperative mobilization.
Non-specific NSAIDs
Non-specific NSAIDs are often effective for mild to moderate pain following outpatient surgery,52 although their analgesic efficacy and adverse effects show marked variation among individuals. Non-specific NSAIDs may also be used as adjuncts to opioids for the management of more severe postoperative pain.
Monotherapy
Ketorolac tromethamine is the only parenteral non-specific NSAID approved for use in the United States and is the current standard for comparison. Although ketorolac is useful in the management of postoperative pain, it is a reversible inhibitor of platelet aggregation.53,54 Theoretically, platelet inhibitors should increase the risk of postoperative bleeding. However, a postmarketing surveillance study of patients receiving 10 272 courses of parenteral ketorolac therapy reported only a small increase in operative-site bleeding, which was limited to elderly patients and those receiving high-dose ketorolac or more than 5 days of treatment.55 Other studies, however, have reported an increase in operative and postoperative bleeding,56–59 including cases of severe and near-fatal postoperative bleeding.59 Studies in healthy volunteers have found single-dose ketorolac to prolong bleeding time significantly,60 with one study noting a 50% increase in bleeding time 4 hours after a single intramuscular dose.61 Consequently, ketorolac is not routinely used before surgery in which operative and postoperative bleeding may be problematic.
Ketoprofen is a commonly used non-specific NSAID that provides effective analgesia for mild to moderate pain. It is also used concurrently with opioids in the treatment of severe pain, and several studies have investigated its efficacy as a monotherapy for postoperative pain relief. A study in patients with moderate or severe pain following dental surgery reported that ketoprofen provided a superior level of meaningful pain relief at 6 hours compared with liquigel ibuprofen and acetaminophen. Ibuprofen, however, provided faster relief and superior overall efficacy.62 In a further study following dental surgery, ketoprofen demonstrated lower pain intensity from 2 to 6 hours after first drug intake and decreased swelling compared with acetaminophen.63
Following minor pediatric surgery, ketoprofen had an earlier onset and longer duration of analgesia than acetaminophen.64 Although the data were from too small a study to assess the effects of ketoprofen on hemorrhage rate, an anesthetic induction dose of ketoprofen in patients undergoing adenoidectomy did not increase the rate of blood loss.65
A meta-analysis of 7 randomized, double-blind tonsillectomy trials (n=505) showed that postoperative use of non-specific NSAIDs, such as ketorolac, ibuprofen, or ketoprofen, increased both the risk of postoperative bleeding requiring treatment and the risk of reoperation for hemostasis.66 The authors of the meta-analaysis searched PubMed® and the Cochrane Controlled Trials Register for randomized, double-blind studies published between January 1966 and May 2001. Trials were required to meet quality criteria for inclusion and were selected if they reported data on postoperative bleeding in patients treated with non-specific NSAIDs after tonsillectomy with or without adenoidectomy. In the 7 trials combined in the meta-analysis, the incidence of postoperative bleeding treated medically or surgically was 7.3%. The most common consequence of this bleeding was admission to the emergency department.
Adjunctive therapy
In the early postoperative period, a combination of opioids and non-specific NSAIDs can improve analgesic efficacy compared with either agent alone.12 The combined use of ketoprofen and opioids in patients with severe pain lowers opioid doses and reduces the incidence of opioid-related adverse events.67 Other studies have also shown that combination therapy lowers opioid requirements and decreases opioid-induced nausea, constipation, somnolence, and respiratory depression.52 The resulting increase in gastrointestinal motility may permit an earlier return to oral nutrition.68 Although the use of ketorolac and diclofenac has been reported to reduce morphine consumption by 30–40% following abdominal and orthopedic surgery,69,70 non-specific NSAIDs increase the risk of postoperative bleeding.13 For this reason, non-specific NSAIDs should probably not be used following hip arthroplasty. In addition, the common use of anticoagulation therapy with heparin following total knee surgery to prevent deep vein thrombosis also increases the risk of postoperative bleeding.
A study in patients receiving short-term ketorolac infusion following laparoscopic day surgery reported that, although ketorolac had an opioid-sparing effect over 36 hours of postoperative administration, it was not associated with continued benefits after this time.71 Although patients required significantly less fentanyl in the recovery ward and significantly less codeine prior to and following discharge, patients’ description of discomfort on performing common activities did not indicate any beneficial effect of ketorolac.
Adverse effects of non-specific NSAIDs
Although non-specific NSAIDs do not share many of the adverse effects associated with opioid use, non-specific COX inhibition can cause gastrointestinal ulceration and bleeding, platelet dysfunction, prolonged bleeding time, elevated blood pressure, renal toxicity, and hepatic dysfunction.13–15,55 These events may range from mild to potentially life-threatening complications.
The gastrointestinal adverse effects of non-specific NSAIDs are caused by inhibition of COX-1. In the gastrointestinal tract, COX-1 produces prostaglandin E2, which has a protective effect on the gastrointestinal mucosa by limiting acid damage and promoting mucus secretion, bicarbonate release, and adequate blood flow.16 The resulting gastrointestinal damage caused by COX-1 inhibition increases the incidence of ulceration, perforation, and potentially fatal gastrointestinal bleeding.15
Non-specific NSAIDs also inhibit normal platelet function and aggregation. Inhibition of COX-1 reduces platelet aggregation by blocking the production of thromboxane A2. Because COX-2 normally inhibits prostacyclin, a protein that increases platelet aggregation, normal platelet aggregation is further reduced.60
Although prostaglandins do not play a major role in the maintenance of basal renal function under normal physiologic conditions, prostaglandins produced by constitutive renal COX-1 and COX-2 may be vital to the maintenance of renal perfusion and glomerular filtration rate.72,73 In patients with compromised renal hemodynamics, such as the elderly or those with preexisting renal dysfunction or congestive heart failure, NSAID-mediated COX-1 inhibition can result in acute renal failure.74
COX-2-specific inhibitors
Because of the many adverse effects associated with opioids and non-specific NSAIDs, alternative analgesic agents are needed. The antiinflammatory and analgesic benefits of NSAIDs are due to the inhibition of COX-2 at the site of inflammation.34,39,75 The belief that selective inhibition of COX-2 would produce the beneficial effects of NSAIDs, but avoid disruption of COX-1-mediated protective functions, led to the development of COX-2-specific inhibitors. The indications for the currently available COX-2-specific inhibitors in the United States and Europe are presented in Table 10.2.
Table 10.2 Indications for Currently Available COX-2-Specific Inhibitors in the United States and Europe
Analgesic efficacy of COX-2-specific inhibitors
Studies of COX-2-specific inhibitors in the perioperative setting demonstrate that they provide effective analgesia while reducing opioid consumption in the range of 20–70%.7
Parenteral COX-2-specific inhibitors
The analgesic efficacy and opioid-sparing effects of parecoxib have been demonstrated in a number of studies of postoperative pain (Table 10.3). Following total hip arthroplasty, the use of parecoxib 20 mg or 40 mg significantly reduced the total amount of morphine used over 36 hours compared with placebo (p<0.01).76 Patients treated with parecoxib also reported significantly greater maximum pain relief and earlier discontinuation of morphine therapy. Parecoxib 20 mg or 40 mg has also demonstrated opioid-sparing effects and significant analgesic efficacy compared with morphine alone (p<0.05) following total knee replacement surgery.77 Another study compared parecoxib 20 mg and 40 mg, morphine 4 mg, and ketorolac 30 mg following unilateral total knee replacement surgery. Although the onset of analgesia in all groups was similar, the level and duration of analgesia with parecoxib 40 mg was significantly superior to morphine 4 mg.78
Table 10.3 Studies Investigating the Use of Parecoxib in the Management of Preoperative and Postoperative Pain
Study | Treatment and Model | Results |
---|---|---|
Barton et al.81 |
Single-dose parecoxib 20 mg and 40 mg i.m. and i.v. have been compared with ketorolac 60 mg following oral surgery.79 The analgesic efficacy of both parecoxib dosing regimens was comparable, but time to use of rescue medication was longer following intramuscular administration. Parecoxib 40 mg was found to be comparable to ketorolac in most measures of analgesia, but had a longer duration of action. In another oral surgery study, single-dose parecoxib 20–100 mg and ketorolac 30 mg demonstrated a similarly rapid onset of analgesia (within 11 minutes) and comparable analgesic efficacy.80 Dose-proportional increases in the duration of analgesia were observed with parecoxib 50 mg and 100 mg, showing a significantly longer duration of analgesia than ketorolac.
Following gynecologic laparotomy surgery, parecoxib 20 mg or 40 mg demonstrated analgesic efficacy similar to that of intravenous ketorolac 30 mg and a similarly rapid time to onset of analgesia (10–23 min).81 Parecoxib also demonstrated similar analgesic efficacy and a longer duration of action than morphine 12 mg i.m. in this model.82 The use of preoperative single-dose parecoxib 40 mg followed by a single oral dose of valdecoxib 40 mg following laparoscopic cholecystectomy confirmed the opioid-sparing effects and analgesic efficacy of parecoxib compared with placebo.83
Parecoxib was well tolerated in all of these trials,77,78,80,82 and the adverse events reported in these studies (such as nausea and dizziness) are typical of those seen after surgery.84 Importantly, parecoxib showed no evidence of treatment-related serious adverse events such as wound bleeding, renal dysfunction, or upper gastrointestinal bleeding.78
Oral COX-2-specific inhibitors
The efficacy of the oral COX-2-specific inhibitors celecoxib and valdecoxib for the treatment of preoperative and postoperative pain is summarized in Table 10.4.
Table 10.4 Studies Investigating the use of Oral COX-2-Specific Inhibitors in the Management of Preoperative and Postoperative Pain
Study | Treatment and Model | Results |
---|---|---|
Celecoxib | ||
Gimbel et al.85 |