6 Non-Balloon Coronary Interventional Techniques and Devices Rotational Atherectomy, Thrombectomy, Cutting Balloons, and Embolic Protection Devices
Rotational Atherectomy
Indications and Contraindications
1. The treatment of fibrocalcific plaques with balloon angioplasty often results in incomplete lesion dilation, which subsequently increases the risk of restenosis or acute/subacute stent thrombosis. Aggressive attempts to dilate resistant lesions with either high-pressure inflations or the use of noncompliant balloons carries the risk of vessel wall injury (i.e., dissection or perforation).
2. Non-dilatable lesions often inhibit the passage of balloons and stents, thereby limiting options for definitive revascularization. In these clinical situations, initial plaque debulking with RA may improve initial procedural success by improving arterial compliance, thereby facilitating more uniform and symmetric stent deployment while potentially allowing for increases in postprocedure minimal lumen diameter. Finally, while treating bulky lesions at or near a large branch vessel, preemptive use of RA may help minimize plaque shifting (i.e., the “snow-plowing” phenomenon) and alleviate the need for subsequent side branch intervention.
Although RA may assist in the delivery and deployment of interventional equipment (i.e., balloons and stents), there are specific clinical situations where its use should either be used with great caution or avoided altogether (Table 6-1).
Table 6-1 Indications and Contraindications to Rotational Atherectomy
Indicated | High-Risk | Contraindicated |
---|---|---|
LV, left ventricular; LVEF, left ventricular ejection fraction; PCI, percutaneous coronary intervention.
Equipment
The Rotablator Rotational Atherectomy System (Boston Scientific Corporation, Natick, MA) is the only commercially available RA system (Figs. 6-1 and 6-2). The system consists of a nickel-plated brass elliptical burr (available in sizes of 1.25–2.5 mm in diameter) that is coated on its leading edge with diamonds that are 20 to 30 microns in diameter. The burr is attached to a long, flexible driveshaft that is inserted through a guide catheter (6–10 F; see Table 6-2) over a 0.009-inch stainless steel guidewire (i.e., RotaWire). The RotaWire is available in both extra-support and floppy grades of stiffness. Whereas the floppy RotaWire is used in the majority of cases, the extra-support version may assist in advancing the device to very distal lesions. Finally, the Rotablator driveshaft itself is contained in a 4.3 F Teflon sheath and is connected to a turbine driven by compressed nitrogen gas. A continuous infusion of pressurized emulsifier solution (i.e., Rotaglide) with saline is infused through the drive shaft to aid lubrication and heat dissipation.
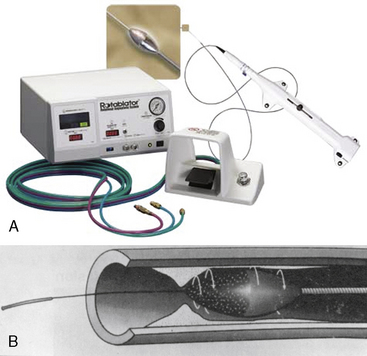
Figure 6-1 A, Rotablator Rotational Atherectomy System. B, Diagram of the rotational atherectomy burr.
(Courtesy of Boston Scientific Corp., Natick, MA.)
Table 6-2 Recommended Guide Catheter Sizes for Use With the Coronary Rotablator
Rotablator Burr Size (mm) | Recommended Guide Catheter Internal Diameter (inch) | Guide Size (French [F])* |
---|---|---|
1.25 | 0.053 | 6–8 |
1.50 | 0.063 | 6–8 |
1.75 | 0.073 | 6–8 |
2.00 | 0.083 | 7–9 |
2.15 | 0.089 | 7–9 |
2.25 | 0.093 | 7–9 |
2.50 | 0.102 | 9–10 |
* For a given size of catheter, the inside diameter varies from manufacturer to manufacturer. French sizes assume thin-wall (high-volume flow) catheters with side holes.
Technique and Technical Tips
Crossing of the lesion can be performed either with the RotaWire or with a standard 0.014-inch guidewire that can subsequently be exchanged for a RotaWire using either a tracking catheter or an over-the-wire balloon system. Although the RotaWire size (0.009-inch) often makes crossing lesions challenging, in cases where exceptionally severe lesions preclude the passage of either a tracking catheter or over-the-wire balloon catheter, direct wiring with the RotaWire may in fact be necessary. The infusion port on the drive shaft is connected to a pressurized bag of saline and lubricant mixture (i.e., Rotaglide—egg yolk/olive oil/EDTA mixture). A combination of verapamil (10 mg/L), nitroglycerin (4 mg/L), and heparin (2000 U/L) can also be added to the saline flush in order to minimize vessel spasm during RA. After loading onto the RotaWire, the burr’s speed is tested (i.e., platforming) prior to introduction into the guide catheter. The burr speed during platforming should range from 160,000 to 180,000 rpm, depending on the burr size (Table 6-3). Following successful platforming, the burr is advanced through the guide catheter and is positioned immediately proximal to the lesion. Advancement of the burr through the guide catheter around the aortic arch often requires the operator tasked with securing the back end of the RotaWire to provide additional back tension to both facilitate burr advancement and limit acquired tension. Although many operators will transiently activate the system inside the guide to further alleviate acquired tension within the system, activating the system in the vessel proximal to the lesion accomplishes the same goal of preventing the burr from “leaping forward” during the initial RA pass. Direct intracoronary administration of vasodilators prior to system activation can be performed at this time to combat coronary spasm potentially instigated by RA.
Of note, in particularly severely narrowed lesions with heavy calcium, the RotaWire may retract proximally during burr advancement. By ensuring coaxial guide catheter alignment and applying gentle forward pressure on the guide catheter, distal wire position can be maintained. Finally, if excessive decelerations (i.e., > 5000 rpm decrease) repeatedly occur during RA, it is recommended to downsize to a smaller burr. Figures 6-3 and 6-4 are case examples demonstrating the use of RA in various clinical settings.
No Reflow or Slow Reflow After Rotablator Ablation
No reflow or slow reflow is the occurrence of no blood flow (no reflow) or blood flow reduced by one angiographic thrombolysis in myocardial infarction (TIMI) study flow grade (slow reflow) in the treated artery despite the fact that the treated segment is patent. No reflow or slow reflow is believed to occur because of the transient increase in blood viscosity due to the presence of microparticles or vasospasm at the level of the distal microvasculature. No reflow or slow reflow has been observed in 6% to 7% of patients undergoing PTCA (see Fig. 6-2). No reflow and slow reflow can be minimized by the following actions:
2. Using a stepped burr approach (smaller, then larger) in long or calcified lesions
3. Using a “pecking” motion at the plaque to avoid blocking the arterial lumen
4. Maintaining maximum blood flow by repeated flushing with saline (bolus of 10–30 mL)
5. Using guide catheters with side holes
6. Maintaining the left ventricular filling pressures (and mean arterial pressure) by increasing the patient’s volume status appropriately
When performed in a controlled setting by an experienced operator, RA offers a safe and effective means of debulking plaque and preparing lesions for stent implantation. Additional technical tips on performing safe and successful RA are listed in Table 6-4.
Table 6-4 Technical Notes and Tips on Performing Rotational Atherectomy
Clinical Outcomes
Although prospective and retrospective studies have demonstrated the safety and efficacy of rotational atherectomy in treating complex coronary lesions with regard to immediate procedural success and postprocedure MLD when compared to either balloon angioplasty or balloon angioplasty followed by stenting, the long-term data supporting the use of RA to reduce restenosis or TVR for treatment of both de novo coronary lesions (including chronic total occlusions) and in-stent restenosis remain less conclusive (Table 6-5).
Mechanical Thrombectomy
Indications
Angiographically, intracoronary thrombus is recognized as a filling defect surrounded on three sides by contrast visible in multiple projections in the absence of calcification, with or without persistent contrast staining (Fig. 6-5). Other angiographic features of thrombus include reduced contrast density and/or haziness. The overall sensitivity and specificity of detecting thrombus by angiography when compared to angioscopy is reported as 26% and 92%, respectively.
Mechanical Thrombectomy Systems
Aspiration Thrombectomy
Aspiration thrombectomy devices are dual lumen tubes (Fig. 6-6) that are passed across the target lesion over a standard 0.014-inch guidewire. The smaller lumen houses the guidewire using a rapid exchange monorail system while the larger, aspiration lumen connects the distal aperture(s) to a proximal port that is attached to a large (30–50 mL) lockable syringe.
Technique and Technical Tips
Aspiration thrombectomy catheters are appealing because of their simplicity and ease of use. Virtually no preparation of the catheter is necessary (short of flushing the aspiration lumen with saline), and thus there is no delay in achieving rapid reperfusion during primary PCI. The technique, however, is limited by catheter deliverability (especially in tortuous, small, or calcified vessels), trackability, and pushability. In addition, the maximum thrombus extraction rate is limited to the minimum diameter of the extraction lumen. Although extraction of blood and thrombus may be up to three times more effective using the larger 7 F thrombectomy catheters, these benefits are traded for the increased risk of difficult delivery (i.e., larger profile, stiffer catheter), restriction of use in only larger epicardial vessels, and increased risk of vessel injury (e.g., dissection, perforation, etc.). Figure 6-7 is a case example demonstrating the utility of aspiration thrombectomy.
Rheolytic Thrombectomy
The AngioJet Rheolytic Thrombectomy System (MEDRAD, Inc., Warrendale, PA) aspirates thrombus by creating high-pressure water jets directed backward into the aspiration catheter, thereby producing a strong suction (approximately 600 mm Hg) at the space near the catheter tip (i.e., Venturi effect). Thrombotic material is drawn into the catheter shaft where the powerful water jets macerate and extract it. Cross-Stream technology enhances thrombus removal by allowing a small amount of saline to wash into the coronary artery prior to maceration and extraction (Fig. 6-8). The system can be used to aspirate and remove intracoronary thrombus in the setting of acute MI, stent thrombosis, saphenous vein graft thrombosis, and thrombosed peripheral vessels or grafts (Fig. 6-9).
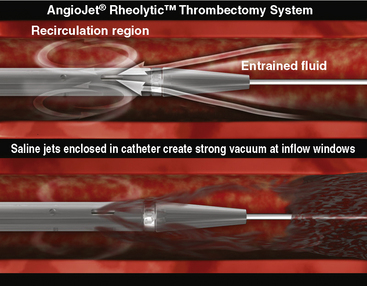
Figure 6-8 AngioJet Rheolytic Thrombectomy System Cross-Stream technology.
(From MEDRAD, Inc.,Warrendale, PA.)
Cutting Balloons
The Flextome Cutting Balloon (Boston Scientific Corporation, Natick, MA) is a special balloon catheter that was designed to reduce trauma to the vessel wall and on plaque by making small incisions into the plaque. Doing so theoretically may limit the splitting and tearing of the plaque and vessel wall as commonly occurs during standard balloon angioplasty. The Flextome Cutting Balloon has three or four 0.1- to 0.4-mm thick stainless steel blades (i.e., atherotomes) that are fixed to the surface of the balloon (Fig. 6-10). The blades are safety embedded within the folds of the undeployed balloon to allow for safe delivery to the lesion site. Upon balloon inflation, the blades are purported to make microscopic incisions in the plaque and do not cut through the plaque completely. The force of inflation is concentrated on the incising element, thereby allowing for predictable cutting of the coronary plaque.
Indications and Contraindications
The cutting balloon is indicated primarily in three clinical situations:
Technique and Technical Tips
• Use a 1.5- to 2.0-mm conventional balloon to predilate the lesion.
• Use the buddy wire technique in tortuous segments or into a stent.
• Use a Wiggle wire (Abbott Vascular, Abbott Park, IL) to aid with wire bias.
• Inflate the device when it is partially in the lesion and then advance the device as it deflates.
Embolic Protection Devices
Embolic Protection Systems
A number of embolic protection systems have been developed throughout the years, each employing one of two primary mechanisms to capturing embolic material: balloon occlusion (either proximal to distal to the lesion) to trap particles which are subsequently aspirated, or deployment of a filter device distal to the lesion to trap antegrade traveling particulate matter. Table 6-6 outlines the major advantages and disadvantages of each system type (i.e., balloon occlusion vs. filter).
Table 6-6 Advantages and Disadvantages of Embolic Protection Device Systems
Filter | Balloon Occlusion | |
---|---|---|
Perfusion | Permits antegrade blood flow | Prevents antegrade blood flow during use precipitating ischemia if no collateral flow is present |
Emboli | Traps emboli > filter pore size (e.g., 100 μm) | All emboli and debris remain stagnant, allowing for aspiration prior to restoration of antegrade blood flow |
Vasoactive substances & cytokines | Freely flows through filter | Limited ability to reach the distal bed due to lack of antegrade blood flow |
Technical considerations in crossing lesions | Bulky; risk of distal embolization prior to device deployment | Lower profile; ability to be deployed prior to crossing the lesion, minimizing distal embolization |
Retrieval considerations | Full of debris; may be difficult to collapse and fully retrieve filter; may become ensnared in proximal stent | Slow balloon deflation may prolong ischemic time |
Embolization during device placement | Possible | Possible (unlikely with proximal balloon occlusion devices, however) |
Visualization of distal vessel | Adequate | Compromised during balloon inflation |
Balloon Occlusion
The best known EPD utilizing proximal balloon occlusion is the Proxis Embolic Protection System (St. Jude Medical, Inc., St. Paul, MN). The device is housed in a 3.6 F infusion catheter that is advanced through a 7 F guide catheter over a standard guidewire and is positioned proximal to the lesion. The balloon is inflated, which occludes antegrade flow, creating a stagnant column of blood that is aspirated after the intervention before coronary blood flow is restored upon balloon deflation (Fig. 6-11). The Proxis system is designed for use in vessels 3.0 to 5.0 mm in diameter. Use in larger diameter vessels is not recommended, as the occlusion balloon may not allow for complete vessel occlusion. Similarly, use of the device in smaller diameter vessels is not recommended because of the risk of vessel trauma during inflation of the occlusion balloon.
Distal Embolic Protection
The Filterwire EZ Embolic Protection System (Boston Scientific Corporation, Natick, MA) is comprised of a uniform, 110-micron-pore basket filter fixed to a guidewire that, when released, expands up to 5.5 mm (Fig. 6-12). Prior to deployment, the entire Filterwire EZ system is advanced across the lesion, positioned in the distal vessel, and subsequently deployed. At the conclusion of the procedure, the filter is collapsed into a proprietary retrieval catheter, trapping the particulate matter, and is removed from the body. The primary advantage of the Filterwire EZ system over the balloon occlusion-based systems is that it allows for antegrade blood flow throughout the procedure while still capturing larger embolic particles. Although the Filterwire EZ system may theoretically not capture small particulate matter because of limitations in filter pore size, studies comparing it to balloon occlusion systems have demonstrated similar debris size distribution and equivalent clinical outcomes. The primary limitation of the Filterwire EZ system is the need for an adequate distal “landing zone” to safely deploy the device (25–30 mm from the distal edge of the lesion).
The SpiderFX Embolic Protection Device (ev3, Inc., Plymouth, MN) is another distal protection device that can be employed during SVG interventions (Fig. 6-13). The device comes in a variety of filter sizes (ranging from 3.0 to 7.0 mm), is heparin coated, and offers the advantage of delivery over any standard 0.014-inch interventional guidewire (unlike the Filterwire EZ, which is integrated onto the guidewire itself). The filter is delivered via a 3.2 F catheter employing a rapid exchange (SpideRX) system and, following intervention, is retrieved using a separate 4.2 F or 4.9 F SpideRX retrieval catheter.
Albiero R., Silber S., Di Mario C., et al. Cutting balloon versus conventional balloon angioplasty for the treatment of in-stent restenosis: results of the restenosis cutting balloon evaluation trial (RESCUT). J Am Coll Cardiol. 2004;43:943–949.
Ali A., Cox D., Dib N., et al. Rheolytic thrombectomy with percutaneous coronary intervention for infarct size reduction in acute myocardial infarction: 30-day results from a multicenter randomized study. J Am Coll Cardiol. 2006;48:244–252.
Antoniucci D., Valenti R., Migliorini A., et al. Comparison of rheolytic thrombectomy before direct infarct artery stenting versus direct stenting alone in patients undergoing percutaneous coronary intervention for acute myocardial infarction. Am J Cardiol. 2004;93:1033–1035.
Braden G., Young T., Love W. Rotational atherectomy of chronic total coronary occlusion is associated with very low clinical rates: the treatment of choice. J Am Coll Cardiol. 33, 1999. (Suppl A):48A
Brogan W.C.3rd, Popma J.J., Pichard A.D., et al. Rotational coronary atherectomy after unsuccessful coronary balloon angioplasty. Am J Cardiol. 1993;71:794–798.
Buchbinder M., Fortuna R., Sharma S. Debulking prior to stenting improves acute outcomes: early results from the SPORT trial. J Am Coll Cardiol. 35, 2000. 8A
Burzotta F., Trani C., Romagnoli E., et al. Manual thrombus-aspiration improves myocardial reperfusion: the randomized evaluation of the effect of mechanical reduction of distal embolization by thrombus-aspiration in primary and rescue angioplasty (REMEDIA) trial. J Am Coll Cardiol. 2005;46:371–376.
de Feyter P.J., Ozaki Y., Baptista J., et al. Ischemia-related lesion characteristics in patients with stable or unstable a angina. A study with intracoronary angioscopy and ultrasound. Circulation. 1995;92:1408–1413.
Halkin A., Masud A.Z., Rogers C., et al. Six-month outcomes after percutaneous intervention for lesions in aortocoronary saphenous vein grafts using distal protection devices: results from the FIRE trial. Am Heart J. 2006;151:915e1–915e7.
Henneke K.H., Regar E., Konig A., et al. Impact of target lesion calcification on coronary stent expansion after rotational atherectomy. Am Heart J. 1999;137:93–99.
Kim M.S., Wang T.Y., Dai D., et al. Association of previous coronary artery bypass graft surgery with door-to-balloon time and in-hospital outcomes: a report from the National Cardiovascular Data Registry (NCDR). J Am Coll Cardiol. 2008;52:1665–1670.
Mauri L., Bonan R., Weiner B.H., et al. Cutting balloon angioplasty for the prevention of restenosis: results of the Cutting Balloon Global Randomized Trial. Am J Cardiol. 2002;90:1079–1083.
Mauri L., Cox D., Hermiller J., et al. The PROXIMAL trial: proximal protection during saphenous vein graft intervention using the Proxis Embolic Protection System: a randomized, prospective, multicenter clinical trial. J Am Coll Cardiol. 2007;50:1442–1449.
Mauri L., Reisman M., Buchbinder M., et al. Comparison of rotational atherectomy with conventional balloon angioplasty in the prevention of restenosis of small coronary arteries: results of the Dilatation vs. Ablation Revascularization Trial Targeting Restenosis (DART). Am Heart J. 2003;145:847–854.
Quan V.H., Huynh R., Seifert P.A., et al. Morphometric analysis of particulate debris extracted by four different embolic protection devices from coronary arteries, aortocoronary saphenous vein conduits, and carotid arteries. Am J Cardiol. 2005;95:1415–1419.
Reifart N., Vandormael M., Krajcar M., et al. Randomized comparison of angioplasty of complex coronary lesions at a single center. Excimer Laser, Rotational Atherectomy, and Balloon Angioplasty Comparison (ERBAC) Study. Circulation. 1997;96:91–98.
Resnic F.S., Wainstein M., Lee M.K., et al. No-reflow is an independent predictor of death and myocardial infarction after percutaneous coronary intervention. Am Heart J. 2003;145:42–46.
Silva-Orrego P., Colombo P., Bigi R., et al. Thrombus aspiration before primary angioplasty improves myocardial reperfusion in acute myocardial infarction: the DEAR-MI (Dethrombosis to Enhance Acute Reperfusion in Myocardial Infarction) study. J Am Coll Cardiol. 2006;48:1552–1559.
Stone G.W., Rogers C., Hermiller J., et al. Randomized comparison of distal protection with a filter-based catheter and a balloon occlusion and aspiration system during percutaneous intervention of diseased saphenous vein aorto-coronary bypass grafts. Circulation. 2003;108:548–553.
Sharma S., Kini A., King T. Randomized trial of rotational atherectomy vs. balloon angioplasty for diffuse instent restenosis (ROSTER): final results. Circulation, 102. 2000. I1730
Svilaas T., Vlaar P.J., van der Horst I.C., et al. Thrombus aspiration during primary percutaneous coronary intervention. N Engl J Med. 2008;358:557–567.
Taeymans Y., Theroux P., Lesperance J., et al. Quantitative angiographic morphology of the coronary artery lesions at risk of thrombotic occlusion. Circulation. 1992;85:78–85.
Tsuchikane E., Otsuji S., Awata N., et al. Impact of pre-stent debulking for chronic coronary occlusions on restenosis reduction. J Invas Cardiol. 2001;13:584–589.
Vlaar P.J., Svilaas T., van der Horst I.C., et al. Cardiac death and reinfarction after 1 year in the Thrombus Aspiration During Percutaneous Coronary Intervention in Acute Myocardial Infarction Study (TAPAS): a 1-year follow-up study. Lancet. 2008;371:1915–1920.
vom Dahl J., Dietz U., Haager P.K., et al. Rotational atherectomy does not reduce recurrent in-stent restenosis: results of the Angioplasty Versus Rotational Atherectomy for Treatment of Diffuse In-stent Restenosis trial (ARTIST). Circulation. 2002;105:583–588.