5 Non-ablative laser and light skin rejuvenation
Summary and Key Features
• Non-ablative skin resurfacing is a safe and effective means of improving many aspects of photoaged skin
• While non-ablative skin resurfacing provides modest results, it also provides minimal downtime
• Non-ablative resurfacing alters cellular and non-cellular components of the skin without causing an open wound
• Patient selection requires careful consideration of medical factors as well as patient expectations
• Non-ablative modalities rarely require more than local anesthesia
• When performed with thoughtful consideration, complications are rare
• Photodynamic therapy has been utilized to maximize laser–tissue interaction and subsequent improvement in photoaging
• Treatment of types IV–VI skin requires adjustment of laser parameters to minimize pigment alteration
• Patients can generally return to their normal activities in 1–2 days following treatment with non-ablative modalities
• For patients with limited available downtime, multiple treatments 2–3 months apart may provide an improved result
Introduction
The dermis (and / or deeper epidermis) can be selectively damaged by two basic approaches:
1. Targeting discrete chromophores in the dermis and / or at the dermal epidermal junction, or
2. Using mid-infrared lasers in the range of 1.3–1.55 µm wavelengths, where water absorption is weak enough that relatively deep beam penetration is allowed (there is only 50% beam attenuation at depths of 300–1500 µm).
The laser and non-laser systems used for non-ablative rejuvenation are a heterogeneous group of devices that emit wavelengths in the visible (400–760 nm), near-infrared (760–1400 nm), or mid-infrared (1.4–3 µm) ranges, radiofrequency (RF) devices, intense pulsed light (IPL) devices, as well as light-emitting diode (LED) devices (Box 5.1). Each of these modalities can induce dermal remodeling, as well as target other components, without epidermal ablation. Most investigators believe that photothermal heating of the dermis: (1) increases collagen production by fibroblasts and (2) induces dermal matrix remodeling by altering glycosaminoglycans as well as other components of the dermal matrix. Others believe that the laser / light interaction with molecular cellular components alters the cellular function of enzymes as well as cellular structural components. Altering the different components of cells, from enzymes to cellular wall constituents to nucleic acids, may then alter the environment and productivity of a given cell.
Box 5.1
Devices used in non-ablative skin resurfacing
Photodynamic therapy (PDT) with aminolevulinic acid (ALA) has been show to augment the effects of laser or other light sources. Multiple laser and light sources have been used for photoactivation of protoporphyrin IX, leading to improved skin rejuvenation (Fig. 5.1).
Richard Glogau, MD developed a classification scale to chart the progression of clinical photoaging (Table 5.1). One can follow a patient from an early age, with relatively strong homogeneity of skin coloration and minimal wrinkles, to a more aged patient, with wrinkles at rest and a more heterogeneous skin coloration.
As one would expect, treating a Glogau grade I patient with current non-ablative modalities will achieve a higher percentage of photoaging correction versus more severely photodamaged patients. While ablative skin rejuvenation may achieve superior restoration of normal skin structures, especially for the Glogau grade III or IV patient (see Table 5.1), the downtime and potential risks are prohibitive for many patients. Nevertheless, as non-ablative technologies evolve, restoration of young, healthy skin with diminished risks and negligible recovery times is increasingly possible. The remainder of the chapter will focus on patient selection for non-ablative skin rejuvenation and discussion of the different devices.
Patient selection
Patient selection for non-ablative skin rejuvenation begins with an assessment of the degree and type of photoaging (see Table 5.1). The ideal patient is Glogau grade II or III with mild to moderate photodamage. Non-ablative therapies initiate new collagen formation (collagen I and collagen III) and might be appropriate in a Glogau grade I patient to prevent photodamage progression. Alternatively, a patient and / or a physician expecting dramatic change following a non-ablative rejuvenation procedure in a Glogau grade IV patient may be disappointed.
Sadick divides patients in a different manner, where cosmetic deficiencies are based on the histological location of solar damage. His selection process takes into account epidermal (type I) damage (Fig. 5.2) and dermal / subcutaneous (type II) damage (Fig. 5.3), and subsequently treatment is tailored to laser selectivity of the damage.
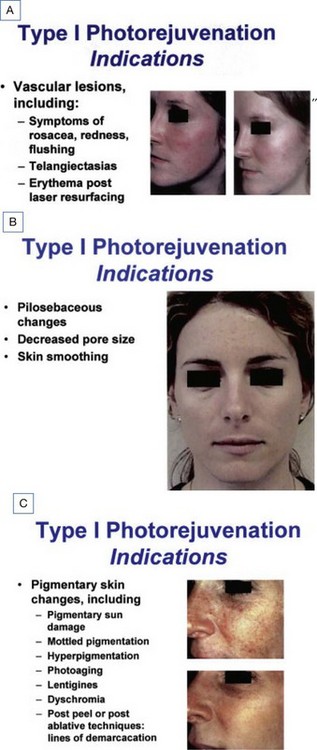
Figure 5.2 (A–C):Type I photoaging indications.
Republished with permission. Sadick NS 2003 Update on non-ablative light therapy for rejuvenation: a review. Lasers in Surgery and Medicine 32:120-128.
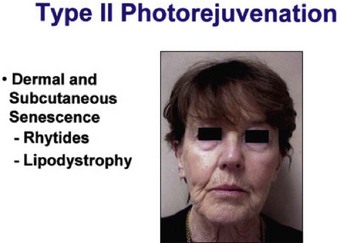
Figure 5.3 Type II Photoaging indications.
Republished with permission. Sadick NS 2003 Update on non-ablative light therapy for rejuvenation: a review. Lasers in Surgery and Medicine 32:120-128.
Another important factor in patient selection is the patient’s Fitzpatrick skin type. Fitzpatrick IV, V, and VI skin types may not be optimal candidates for particular non-ablative rejuvenation modalities that selectively heat melanin. The most common adverse result for non-ablative rejuvenation in darker skin patients is hyperpigmentation, a condition that usually resolves after 4–8 weeks (but can persist longer in some circumstances) with appropriate application of suppressors of melanin synthesis. Mid-infrared lasers, which minimize direct melanin targeting, can be used in patients with darker skin types. However, higher fluences in these patients may result in thermal damage and bulk heating, which can also result in dyspigmentation. Non-cryogen cooling devices can minimize bulk heating, whereas cooling devices that employ cryogen spray may induce pigmentary alterations similar to liquid nitrogen. See Chapter 10 for a detailed discussion of laser and non-laser light sources for the treatment of darker skin types.
Beyond skin type and amount of photodamage, there are some patients who might be excluded from non-ablative lasers and light sources based on medical criteria (Box 5.2). Oral retinoid use, recent rejuvenation procedures, infection, and active dermatitides are reasons to consider deferring a non-ablative rejuvenation procedure. Most likely oral retinoids will not affect the outcome, but no controlled study has investigated their effect on non-ablative skin resurfacing. Many texts advocate waiting a period of 6–12 months, most likely representing an extrapolation from ablative resurfacing wait times. Some cutaneous laser experts have used non-ablative devices 1 month following retinoid use without adverse outcomes.
Box 5.2
Relative contraindications for non-ablative resurfacing
Active dermatitis (i.e. acne, autoimmune disease, etc.)
Active infection (i.e. herpes, impetigo, etc.)
History of keloid / hypertrophic scar formation
History of koebnerizing dermatitis (i.e. psoriasis, vitiligo, etc.)
History of photoinduced dermatitis (i.e. polymorphous light eruption, lupus, etc.)
History of oral retinoid use in the past 6–24 months
Recent ablative resurfacing procedure
Physicians must also consider the wavelength of the device. For example, devices that utilize visible light (i.e. LED devices, etc.) may exacerbate a phototoxicity / photosensitivity or a systemic condition that is photosensitive, like cutaneous lupus (although in a recent study only 7% of SLE patients reacted to visible light) (Fig. 5.4). On the other hand, some lasers may confer a protective quality. There is increasing evidence that IPL can activate fibroblasts as well as confer protection from future UV-induced skin damage.
Visible light and near-infrared / vascular lasers (Table 5.2)
Other wavelengths that target hemoglobin in blood vessels have been shown to rejuvenate skin. The long-pulsed 755 nm alexandrite laser (Case study 1), the 810 nm diode, and the 1064 Nd : YAG lasers are used for deeper and larger-caliber vessels. The subsequent ‘coincidental’ dermal remodeling correlates to the depth of penetration of each respective laser. Weng et al have demonstrated that collagen synthesis by fibroblasts and antioxidant enzymes were significantly increased following irradiation with the 532 nm, 1064 nm Q-switched Nd : YAG, and 1064 nm long-pulse Nd : YAG lasers. The 1064 nm Nd : YAG laser induces deeper remodeling than the 532 nm laser due to its lower degree of dermal scattering and chromophore absorption at 1064 nm. Thus, some physicians use multiple lasers, such as the 532 nm laser to treat dyschromia and telangiectasia, and following it with a pass with the 1064 nm laser to obtain some deeper remodeling in the same treatment session.
A Caucasian female in her early 60s presents for total facial rejuvenation with request to focus on lentigenes, telangiectasias, and overall facial rejuvenation (Fig. 5.5A). The long-pulsed alexandrite 755 nm laser was used at a fluence of 36 J/cm2 using an 8 mm spot size and a 3 ms pulse duration to treat the patient’s forehead, cheeks, nose, and chin. At 6 weeks following her treatment, significant improvement in hyperpigmented macules, telangiectasias, and an overall more youthful appearance is appreciated (Fig. 5.5B).
Photodynamic therapy
Over the past 20 years, photosensitizing agents have enjoyed an increasing role in medical and cosmetic dermatology. Twenty percent 5-aminolevulenic acid (5-ALA, a ‘prodrug’) is absorbed by rapidly proliferating epidermal and dermal cells and converted into photoreactive products of the hemoglobin pathway, most notably protoporphyrin IX (see Fig. 5.1). Protoporphyrin IX is subsequently activated by certain wavelengths of light, as highlighted by the absorption peaks in Figure 5.1, resulting in singlet oxygen production and resultant cellular destruction.
Many light sources have been used for PDT (Box 5.3). This variety is possible owing to multiple absorption peaks by protoporphyrin IX. The largest peaks are at 417, 540, 570, and 630 nm. The PDL, IPL, and LED devices have all been used to activate protoporphyrin IX. There are many variables that affect the immediate PDT response, among them the ALA incubation time, pre-ALA skin preparation regimen, degree of skin photodamage, anatomical region, light dose, wavelength range, and power density. Overall, lower power densities (i.e. continuous wave light sources) create more singlet oxygen than pulsed light. Also, we have found that applying numbing creams simultaneously with the ALA solution can accelerate ALA absorption and thereby accelerate protoporphyrin formation, leading to a much more robust response.
Box 5.3
Lasers and light sources used with ALA-PDT
1. IPL Quantum SR* (Lumenis Ltd., Yokneam, Israel) 2. Lumenis One* (Lumenis Ltd., Yokneam, Israel) 3. VascuLight SR* (Lumenis Ltd., Yokneam, Israel) 4. EsteLux Pulsed Light System* (Palomar Medical Technologies, Burlington, MA) 5. Photogenica V Star* (Cynosure Inc., Chelmsford, MA) |
7. ClearLight* (Lumenis Ltd., Yokneam, Israel)
8. BluU* (Dusa Pharmaceuticals, Wilmington, MA)
9. SkinStation* (Radiancy, Orangeburg, NY)
10. Aurora (Syneron Medical Ltd., Yokneam, Israel)
11. PhotoLight* (Cynosure Inc., Chelmsford, MA)
12. Sciton BBL* (Sciton Inc., Palo Alto, CA)
The following lasers and light secretes are correctly being used by the author for ALA-PDT treatments for aces regards (other devices listed above also able to be used) with similar parameters as listed above:
Data from Gold MH 2005 Skin and Aging 13(2):Feb.
* Device used by author: other settings from colleagues.
(Reproduced with permission of the publisher. Chart appeared in Skin & Aging 13(2):49, 2005.)
Many studies have shown the improvement of actinic keratoses and acne with PDT. Some studies have shown evidence of increased collagen formation. Gold et al have reported improvement in crow’s feet, skin texture, mottled hyperpigmentation, telangiectasias, and actinic keratosis with the addition of 5-ALA prior to IPL treatment (Case study 2).
A 57-year-old white male presents for evaluation and treatment of overall actinic damage, including actinic keratoses and lentigenes on his cheeks (Fig. 5.6A). Treatment was initiated by the application of Levulan® (DUSA Pharmaceuticals, Inc), a 2-hour incubation period, and blue light for 5 minutes; 5% lidocaine cream was placed on the treated areas for the last 30 minutes of the patient’s ALA incubation, which has been shown to enhance ALA absorption and photodynamic therapy effect. Finally, the 532 nm long-pulsed green laser (Gemini®, Laserscope, San Jose, CA) was used with cooling at a fluence of 7 J/cm2 at 18 ms using a 10 mm spot size to treat the entire cheek and nose area. Two months after treatment, the patient’s actinic damage was significantly improved (Fig. 5.6B).
Overview of treatment strategy
Patient selection is important to obtain the best expectation–outcome match. Patients may present for treatment of wrinkles and not the other characteristics of photoaging, even though telangiectasia or lentigines may be present. The modality of treatment is also important as each light device offers unique advantages. For example, if the patient presents with a concern of excessive telangiectasia, use of the 532 nm potassium titanyl phosphate (KTP), a PDL, or an IPL device may be warranted. If the goal is to obtain deeper dermal remodeling, one could consider longer-wavelength modalities such as the mid-infrared devices. Due to the widespread use and versatility of IPLs, many dermatologists are treating multiple photoaging characteristics simultaneously, including hyperpigmentation, telangiectasia, rhytides, and skin texture abnormalities. If the patient presents with actinic keratoses, PDT can be performed either at the same appointment or before or after visible pulsed light treatment for red and brown dyschromias. Often a patient presents with multiple telangiectasias and actinic keratoses. If one uses only a vascular laser or IPL, the actinic damage and the associated telangiectasias within the actinic keratoses will persist or relapse; accordingly, either pre-treatment with 5% fluorouracil cream or PDT will enhance the total rejuvenation effect and decrease the likelihood of an incomplete response (Case study 3).
A 51-year-old white male presents with hyperpigmented patches along bilateral cheeks as well as multiple actinic keratoses on his cheeks and forehead (Fig. 5.7A). Treatment was initiated by the application of Levulan® (DUSA Pharmaceuticals, Inc.), a 2-hour incubation period, and photodynamic therapy illumination. He was subsequently treated with the V-Beam Perfecta® (Candela Corporation, Wayland, MA) using a 10 mm spot size, 8 J/cm2, a 10 ms pulse duration, with 3 bars of cooling to bilateral cheeks. At 6-week follow-up, there was significant reduction in actinic keratoses, solar lentigenes, telangiectasias, and overall facial rejuvenation (Fig. 5.7B).
Alam M, Dover JS. Treatment of photoaging with topical aminolevulinic acid and light. Skin Therapy Letter. 2004;9(10):7–9.
Barolet D, Roberge CJ, Auger FA, et al. Regulation of skin collagen metabolism in vitro using a pulsed 660 nm LED light source: clinical correlation with a single-blinded study. Journal of Investigative Dermatology. 2009;129(12):2751–2759.
Berlin AL, Hussain M, Goldberg DJ. Cutaneous photoaging treated with a combined 595/1064 nm laser. Journal of Cosmetic and Laser Therapy. 2007;9(4):214–217.
Bhat J, Birch J, Whitehurst C, et al. A single-blinded randomised controlled study to determine the efficacy of Omnilux Revive facial treatment in skin rejuvenation. Lasers in Medical Science. 2005;20(1):6–10.
Boulos PR, Kelley JM, Falcao MF, et al. In the eye of the beholder – skin rejuvenation using a light-emitting diode photomodulation device. Dermatologic Surgery. 2009;35(2):229–239.
Chan HH, Yu CS, Shek S, et al. A prospective, split face, single-blinded study looking at the use of an infrared device with contact cooling in the treatment of skin laxity in Asians. Lasers in Surgery and Medicine. 2008;40(2):146–152.
Cho SB, Lee SJ, Kang JM, et al. Treatment of refractory arcuate hyperpigmentation using a fractional photothermolysis system. Journal of Dermatologic Treatment. 2010;21(2):107–108.
Dang Y, Ren Q, Hoecker S, et al. Biophysical, histological and biochemical changes after non-ablative treatments with the 595 and 1320 nm lasers: a comparative study. Photodermatology, Photoimmunology and Photomedicine. 2005;21(4):204–209.
Dang Y, Ren Q, Li W, et al. Comparison of biophysical properties of skin measured by using non-invasive techniques in the KM mice following 595 nm pulsed dye, 1064 nm Q-Switched Nd : YAG and 1320 nm Nd : YAG laser non-ablative rejuvenation. Skin Research and Technology. 2006;12(2):119–125.
Goldman MP, Alster TS, Weiss R. A randomized trial to determine the influence of laser therapy, monopolar radiofrequency treatment, and intense pulsed light therapy administered immediately after hyaluronic acid gel implantation. Dermatologic Surgery. 2007;33(5):535–542.
Gu W, Liu W, Yang X, et al. Effects of intense pulsed light and ultraviolet A on metalloproteinases and extracellular matrix expression in human skin. Photomedicine and Laser Surgery. 2011;29(2):97–103.
Karrer S, Baumler W, Abels C, et al. Long-pulse dye laser for photodynamic therapy: investigations in vitro and in vivo. Lasers in Surgery and Medicine. 1999;25(1):51–59.
Katz BE, Truong S, Maiwald DC, et al. Efficacy of microdermabrasion preceding ALA application in reducing the incubation time of ALA in laser PDT. Journal of Drugs in Dermatology. 2007;6(2):140–142.
Kim HS, Yoo JY, Cho KH, et al. Topical photodynamic therapy using intense pulsed light for treatment of actinic keratosis: clinical and histopathologic evaluation. Dermatologic Surgery. 2005;31(1):33–36. discussion 36-37
Kono T, Groff WF, Sakurai H, et al. Comparison study of intense pulsed light versus a long-pulse pulsed dye laser in the treatment of facial skin rejuvenation. Annals of Plastic Surgery. 2007;59(5):479–483.
Lee SY, Park KH, Choi JW, et al. A prospective, randomized, placebo-controlled, double-blinded, and split-face clinical study on LED phototherapy for skin rejuvenation: clinical, profilometric, histologic, ultrastructural, and biochemical evaluations and comparison of three different treatment settings. Journal of Photochemistry and Photobiology B. 2007;88(1):51–67.
Liu H, Dang Y, Wang Z, et al. Laser induced collagen remodeling: a comparative study in vivo on mouse model. Lasers in Surgery and Medicine. 2008;40(1):13–19.
Ross EV, Sajben FP, Hsia J, et al. Nonablative skin remodeling: selective dermal heating with a mid-infrared laser and contact cooling combination. Lasers in Surgery and Medicine. 2000;26(2):186–195.
Ross EV, Zelickson BD. Biophysics of nonablative dermal remodeling. Seminars in Cutaneous Medicine and Surgery. 2002;21(4):251–265.
Ruiz-Rodriguez R, Lopez-Rodriguez L. Nonablative skin resurfacing: the role of PDT. Journal of Drugs in Dermatology. 2006;5(8):756–762.
Sadick NS. Update on non-ablative light therapy for rejuvenation: a review. Lasers in Surgery and Medicine. 2003;32(2):120–128.
Sadick NS. A study to determine the efficacy of a novel handheld light-emitting diode device in the treatment of photoaged skin. Journal of Cosmetic Dermatology. 2008;7(4):263–267.
Seguchi K, Kawauchi S, Morimoto Y, et al. Critical parameters in the cytotoxicity of photodynamic therapy using a pulsed laser. Lasers in Medical Science. 2002;17(4):265–271.
Sterenborg HJ, van Gemert MJ. Photodynamic therapy with pulsed light sources: a theoretical analysis. Physics in Medicine and Biology. 1996;41(5):835–849.
Tanaka Y, Matsuo K, Yuzuriha S. Objective assessment of skin rejuvenation using near-infrared 1064-nm neodymium:YAG laser in Asians. Clinical, Cosmetic and Investigational Dermatology. 2011;4:123–130.
Wang R, Liu W, Gu W, Zhang P. Intense pulsed light protects fibroblasts against the senescence induced by 8-methoxypsoralen plus ultraviolet-A irradiation. Photomedicine and Laser Surgery. 2011;29(10):685–690.
Weiss RA, McDaniel DH, Geronemus RG, et al. Clinical trial of a novel non-thermal LED array for reversal of photoaging: Clinical, histologic, and surface profilometric results. Lasers in Surgery and Medicine. 2005;36(2):85–91.
Weiss RA, McDaniel DH, Geronemus RG, et al. Clinical experience with light-emitting diode (LED) photomodulation. Dermatologic Surgery. 2005;31(9 pt 2):1199–1205.
Weng Y, Dang Y, Ye X, et al. Investigation of irradiation by different nonablative lasers on primary cultured skin fibroblasts. Clinical and Experimental Dermatology. 2011;36(6):655–660.