19 Neuro-ophthalmology
OCULAR MOVEMENT
SUPRANUCLEAR GAZE CONTROL
Saccadic movements are rapid and relocate fixation of gaze, either reflexly or voluntarily. They are initiated in the contralateral premotor frontal cortex and, once initiated, the movement is irrevocable and ocular position cannot again be modified until the saccade has been completed. A saccade occurs after a latent period of about 200 ms following initiation and has a high velocity of up to 700°/sec. Saccades are tested clinically by instructing the patient to look first at one stationary target and then at another, or to look right and left or up and down with no target present (see Fig. 19.4).
Pursuit movements are slower and are concerned with keeping the target at the fovea. They appear to be generated in the ipsilateral occipital cortex but little is known about the supranuclear pathway. Pursuit movements have a latency of about 125 ms from initiation and a maximum velocity of less than 50°/sec. The movement is smooth and modified continuously according to the speed of the target if the pursuit movement lags behind the target position a corrective saccade is inserted to keep up. Pursuit movements are tested by asking the patient to follow a slowly moving target. It is of great importance that the target is clearly visible and that it is not moved too fast (see Fig. 19.5).
Vestibular ocular movements may be tested by a ‘doll’s head’ manoeuvre, in which the patient is asked to fixate on a target while the examiner rotates the patient’s head (see Fig. 19.6). The doll’s head manoeuvre tests both the left and right labyrinths and may be normal even if one labyrinth is totally nonfunctioning. To test each labyrinth separately, caloric stimulation can be employed which induces nystagmus by syringing the external auditory meatus with cold or warm water. Recently a bedside test has been described, based on the observation that the initial component of a rapid head rotation depends upon the integrity of the labyrinth towards which the head is turned (see Fig. 19.8).
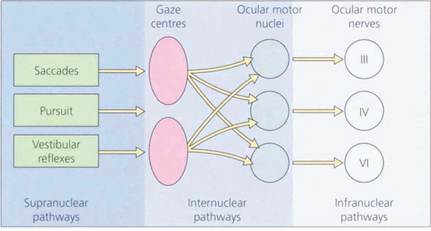
Fig. 19.1 The major inputs to the vertical and horizontal gaze centres are saccadic and pursuit commands from the cerebral hemispheres and the vestibular ocular reflexes from the vestibular nuclei in the medulla. These impulses are then transmitted to the individual ocular motor nuclei in the midbrain and pons. Internuclear pathways coordinate the movements generated in the gaze centres with each other and with the third, fourth and sixth nerve nuclei. Excitatory impulses to an agonist muscle are accompanied by inhibitory impulses to its antagonist.
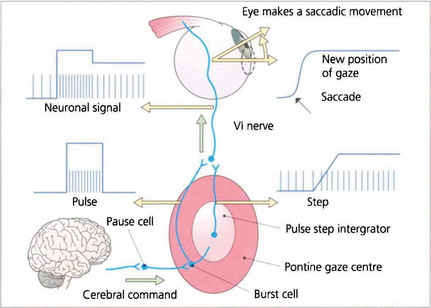
Fig. 19.2 Saccadic movements have been shown biomechanically to be generated by impulses in the form of a pulse and step. To change eye position a rapid burst of neuronal signals (the pulse) is fired by the gaze centre and this rapidly moves the eye to the new position. This rapid burst is integrated in the ‘pulse-step generator’ (a neuronal complex within the gaze centre) to produce an increased tonic discharge (the step) which holds the eye in its new position. Similar responses take place in the agonist of the fellow eye and reciprocal impulses relax the antagonist muscles. These changes are reflected by the EMG recording in an individual muscle. Neurophysiologically saccades are initiated by burst cells in the paramedian pontine reticular formation (PPRF) which are inhibited from firing by pause cells at all times except during a saccade. Thus the saccadic input suppresses the pause cell which permits the burst cell to discharge immediately.
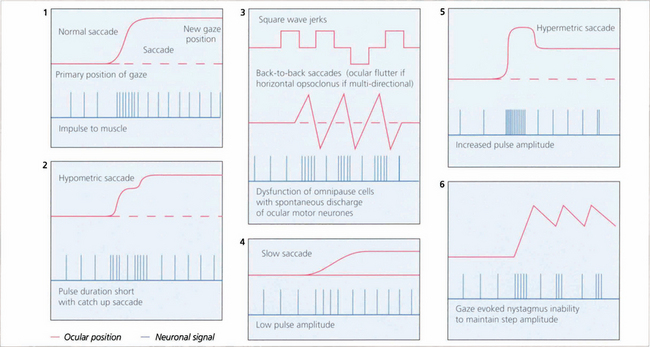
Fig. 19.3 The pulse–step generator exists physiologically in the horizontal gaze centre—otherwise known as the paramedian pontine reticular formation (PPRF)— in the pons and various mismatches of the pulse to step signals have been shown to explain some types of nystagmus and eye movement abnormalities seen in clinical practice.
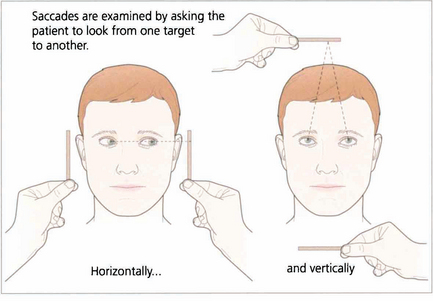
Fig. 19.4 Saccadic eye movements can be elicited simply by asking patients to look right and left or up and down. This is a useful strategy where the observation of large amplitude saccades may reveal subtle abnormalities of saccadic velocity. An alternative strategy is to ask patients to shift fixation from one target to another, in this way saccades of large and small amplitude and involving a variety of gaze shifts can be observed. The accuracy of saccades (under- or over-shooting) can be tested. Slowing of the velocity of saccades may be conjugate (as in a gaze palsy), dysconjugate (as in a lateral rectus paresis or internuclear ophthalmoplegia) or global (as in an ocular myopathy or some forms of cerebellar disease).
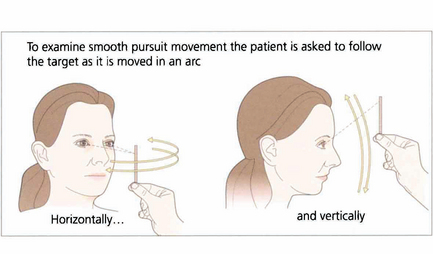
Fig. 19.5 When asking a subject to follow a target it is essential that the target is clearly visible and is moved neither too slowly nor too rapidly. The reader should experiment by trying to follow his or her own finger as it is moved in a 180° arc from one side of the head to the other at arm’s length. A comfortable speed is about 30° per seconds (6 seconds from ear to ear). Pursuit movements are used to examine ductions and versions but, instead of looking at the range of movement, we are now interested in the accuracy of the following movement itself. The observer must watch for saccadic intrusions which will indicate that the eye is not keeping up with the target (in other words, there is reduced gain of the smooth pursuit reflex). This is a universal finding in cerebellar disease.
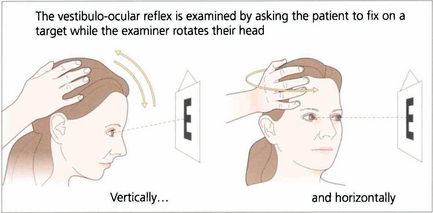
Fig. 19.6 Vestibular ocular reflexes (VORs) are tested by asking the patient to fixate a distant target (such as an acuity chart) and then gently moving the patient’s head from side to side or up and down with increasing frequency until the patient reports movement of the target or the subjective acuity falls, indicating retinal slip of the image. Alternatively a retinal landmark can be fixed using the direct ophthalmoscope while the examiner rocks the head to and fro. Loss of the VOR causes symptoms such as instability of the horizon as the patient walks and is seen not uncommonly with multiple sclerosis.
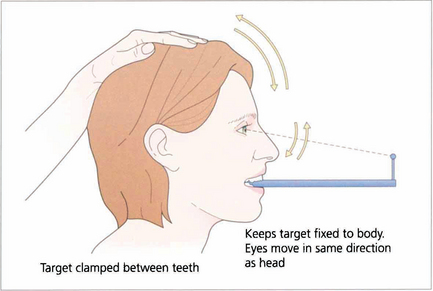
Fig. 19.7 Suppression of the VOR can be tested by asking the patient to grip a target mounted on a spatula between the teeth and to move the head from side to side or up and down with increasing rapidity until the patient reports an inability to keep the image ‘still’ or saccadic eye movements are observed as fixation is lost and taken up again. The mechanism used to suppress the VOR is the pursuit mechanism; if smooth pursuit is abnormal then so will be VOR suppression, and vice versa. Patients who are able to suppress their VOR complain of unusual symptoms such as difficulty with reading when travelling.
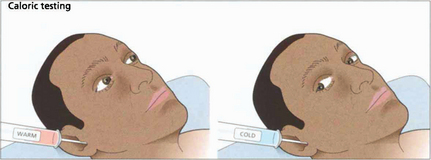
Fig. 19.8 Only one functioning labyrinth is required for the tests described in Fig. 19.6 to be normal. Caloric testing can be used to examine each labyrinth separately. Caloric reflexes are produced by stimulating the semicircular canals and vestibular nuclei with warm or cold water and can provide useful information on the integrity of these pathways in the brainstem; this is especially useful in the neurological assessment of brainstem damage in an unconscious patient. In a conscious patient cold water in the external auditory meatus generates a nystagmus of both eyes with a fast phase to the opposite side but in unconscious patients the saccadic phase is lost and a tonic deviation to the same side is seen. This indicates an intact pons but the results must be interpreted with caution after acute drug overdoses when false-negative responses may be seen. Calorics can be adapted to test vertical gaze (and therefore the integrity of the midbrain) by syringing both ears.
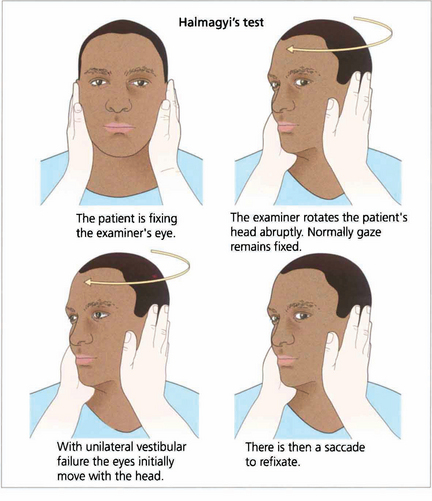
Fig. 19.9 Unilateral labyrinthine function can be tested at the bedside. If the head is rotated abruptly, the initial phase of the VOR is mediated by the labyrinth towards which the head is turned. If that labyrinth is not functioning initially the eyes move with the head and there is a corrective saccade as fixation is taken up again.
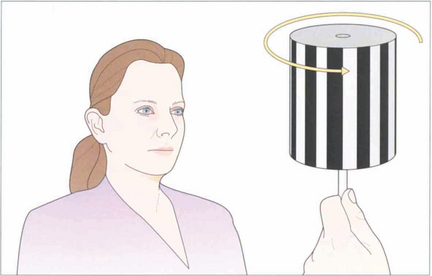
Fig. 19.10 The vestibular system responds only to acceleration and slow head movements (less than 1° per second) stimulate the optokinetic reflex (OKN). The hand-held OKN drum is useful as a bedside test of the pursuit and saccadic systems. If there is a saccadic palsy the eyes will deviate tonically in the direction of the stripe movement: the pursuit mechanism has been elicited but there is no saccade to return gaze towards primary position. Turning the drum to the right elicits an ipsilateral pursuit movement to the right and a contralateral saccade to the left. In essence turning the drum to the right tests the right hemisphere and vice versa to the left.
ANATOMY OF THE OCULAR MOTOR PATHWAYS
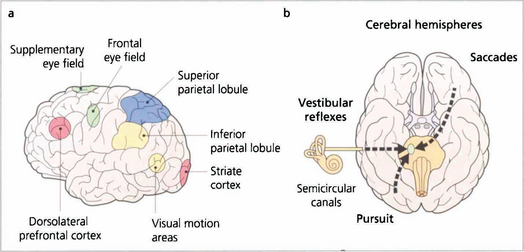
Fig. 19.11 Saccades are generated in a number of areas in the frontal premotor area of the contralateral hemisphere including the frontal eye fields; thus a saccade to the right is generated in the left frontal lobe, passes through the anterior limb of the internal capsule and decussates in the midbrain to the horizontal gaze centre in the pons. The incoming visual information that controls smooth pursuit is analysed in an occipitoparietal motion area. Pursuit movements are then generated in the ipsilateral superior parietal lobule so that a pursuit movement to the right is controlled by the right hemisphere. Little is known of the neuronal pathway, but the final common pathways for saccades and pursuit are the conjugate horizontal and vertical gaze mechanisms in the pons and midbrain. The inferior parietal lobule may be concerned with shifts of attention, which may also generate saccades.
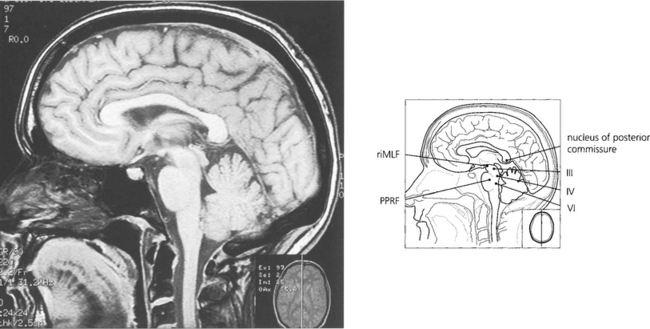
Fig. 19.12 The final common pathway for all horizontal movements are the sixth nerve nuclei to which the PPRF, pursuit mechanisms and vestibular nuclei project. Interneurones in the sixth nerve nucleus project through the medial longitudinal fasciculus (MLF) to the contralateral medial rectus subunit of the oculomotor nucleus. For vertical saccades, the rostral interstitial nucleus of the MLF (riMLF) projects to the third and fourth nerve nuclei. Vestibular inputs controlling vertical eye movements ascend from the lower brainstem to the fourth and third nerve nuclei via the MLF. The nucleus of the posterior commissure projects on to both riMLFs and is particularly important for upgaze.
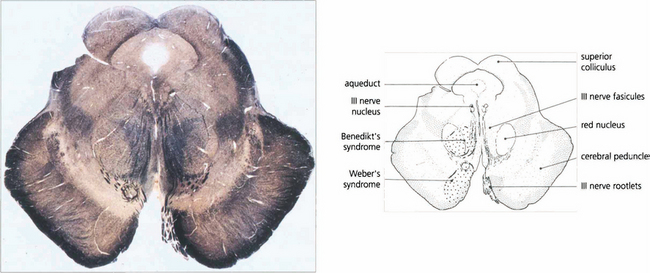
Fig. 19.13 A transverse histological section of the midbrain at the level of the superior colliculus demonstrates the third nerve nucleus inferior to the aqueduct with its efferent fibres. The medial longitudinal fasciculus (MLF) in connecting the third and fourth nerve nuclei and contralateral horizontal gaze centres is responsible for coordinating horizontal and vertical movements. Infarcts in this region cause Weber’s syndrome (unilateral third nerve palsy and contralateral hemiplegia) and Benedikt’s syndrome (unilateral third nerve palsy, contralateral tremor and hyperaesthesia). (Stained to show myelin.)
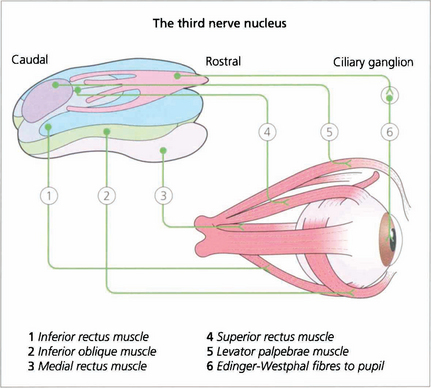
Fig. 19.14 The third nerve nucleus is a complex assemblage of motor neurones. The parasympathetic nucleus (Edinger–Westphal nucleus) of the pupil lies rostrally and superiorly. The inferior rectus, medial rectus and inferior oblique muscles are all supplied from ipsilateral subnuclei, but the superior rectus subnucleus is contralateral. Both levator palpebrae muscles have a single caudal superior nucleus. A complete ‘nuclear’ unilateral third nerve palsy is therefore characterized by ipsilateral inferior oblique, medial and inferior rectus weakness with bilateral ptosis and contralateral superior rectus weakness.
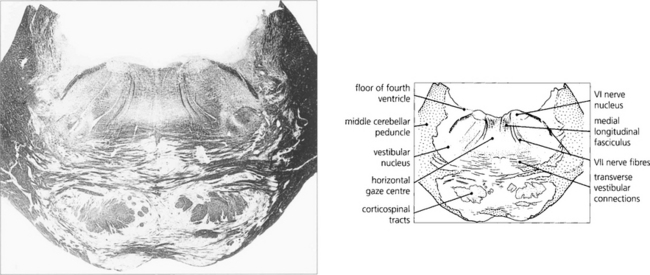
Fig. 19.15 A transverse histological section through the pons at the level of the sixth nerve nuclei demonstrates the medial longitudinal fasciculus (MLF) and the centre for horizontal gaze (PPRF). Interneurones from the PPRF pass initially to the sixth nerve nucleus where they synapse with the MLF (see Fig. 19.34). (Stained to show myelin.)
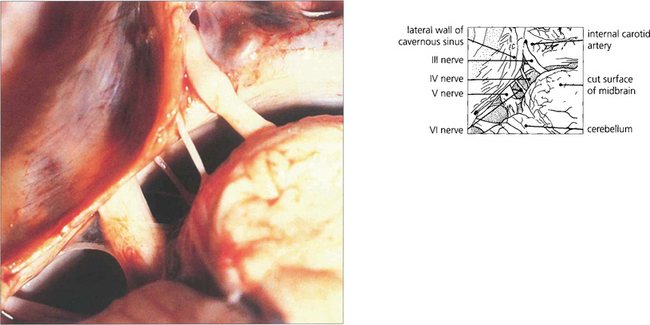
Fig. 19.16 The third and sixth nerves leave the ventral surface of the brain and pass through the interpeduncular cistern before entering the cavernous sinus. The sixth nerve passes almost vertically upwards and bends forwards under the petroclinoid ligament at the apex of the petrous temporal bone. Here it can be compressed against the bone by raised CSF pressure or damaged by fractures or disease in the bone (hence producing the sixth nerve palsy associated with raised intracranial pressure). The fourth nerve leaves the midbrain dorsally, decussates in the superior medullary velum and passes through the subarachnoid space around the midbrain between the superior cerebellar and posterior cerebral arteries before entering the cavernous sinus.
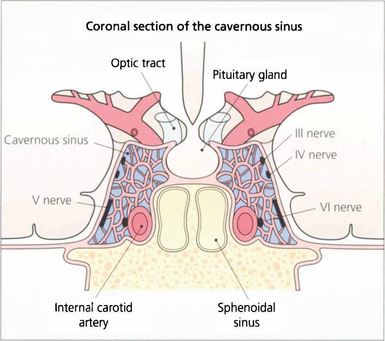
Fig. 19.17 In the cavernous sinus the third and fourth nerves lie superiorly in the lateral wall; at the anterior end the third nerve divides into superior (superior rectus and lid levator) and inferior (inferior and medial rectus, inferior oblique, pupillary and ciliary muscles) branches. Sensory fibres from the eye and forehead enter the cavernous sinus anteriorly in the ophthalmic division of the fifth nerve, lying inferiorly in the lateral wall to join the trigeminal ganglion at the posterior limit. The internal carotid artery and sixth nerve lie within the sinus.
CONJUGATE GAZE PALSIES
HORIZONTAL SUPRANUCLEAR PALSY
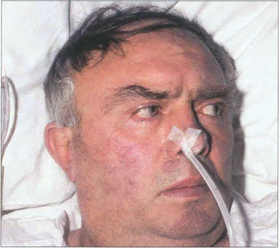
Fig. 19.18 Following an acute cerebrovascular accident involving supranuclear pathways, saccadic gaze is lost in the direction opposite to the side of the lesion and input from the remaining hemisphere deviates the eyes towards the side of the lesion. (Occasionally, irritative lesions, such as cerebral tumours or abscesses, produce deviation of the eyes to the contralateral side.)
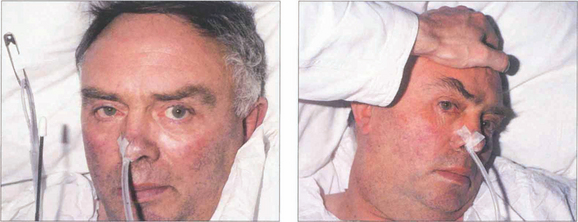
Fig. 19.19 (Left) On command the patient is unable to make a gaze movement to the target on his right. (Right) On doll’s head rotation to the left, however, the eyes deviate fully to the right demonstrating intact pontine reflexes and the supranuclear nature of the lesion.
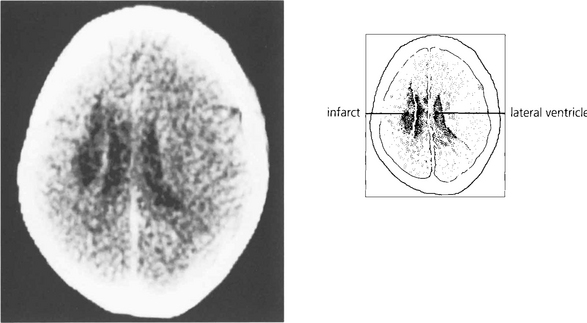
Fig. 19.20 A CT scan of the patient seen in Fig. 19.18, showing a large infarct in the internal capsule due to the occlusion of middle cerebral artery branches, corresponding to lesion 1 in Fig. 19.21. If the patient survives the acute ocular deviation recovers rapidly. Horizontal gaze becomes full and saccades return to normal. This is probably due to a restoration of control mechanisms by the superior colliculus.
VERTICAL GAZE PALSY

Fig. 19.22 This elderly woman suffered a cerebrovascular accident in the upper midbrain. She retained the ability to make rapid and full horizontal saccades.
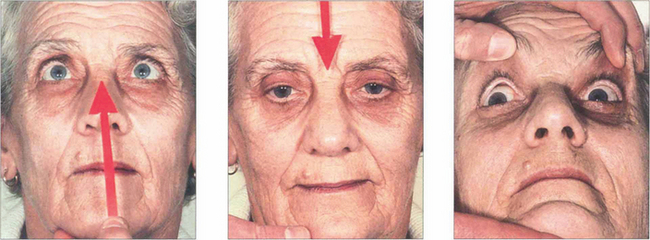
Fig. 19.23 In the same patient, voluntary up and down gaze to saccadic command is lost and compensated for by head movement. ‘Doll’s head’ rotation shows that vertical movements can be produced and that pontine reflexes are intact.
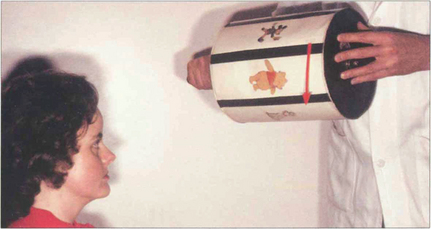
Fig. 19.24 Convergence retraction nystagmus is an interesting ocular movement abnormality sometimes seen with vertical gaze palsies. Bursts of convergence with retraction of both eyes into the orbits are seen either spontaneously or on attempting an upward saccade. These movements may also be accompanied by accommodative spasm which accounts for the frequent complaint of blurred vision. Convergence retraction nystagmus is shown particularly well if the patient views a downward rotating optokinetic drum which stimulates upward refixational saccades.
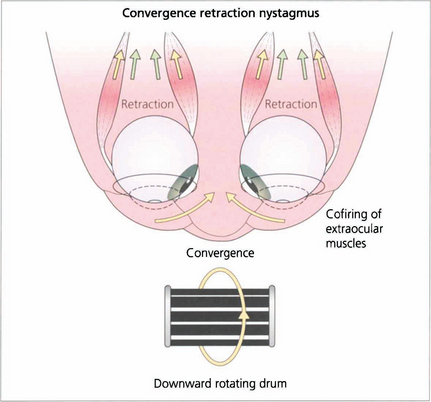
Fig. 19.25 In convergence retraction nystagmus the lesion is thought to cause disinhibition of the ocular motor nuclei allowing bursts of co-firing of the external ocular muscles. The medial recti muscles are the most powerful of the external ocular muscles and consequently the globes converge and retract into the orbits.
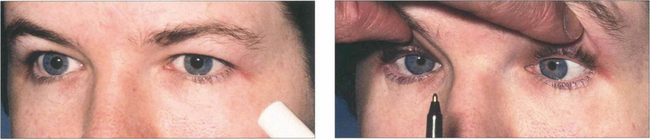
Fig. 19.26 Light–near dissociation of the pupils is the third sign of the triad of Parinaud’s syndrome. The pupils are normally moderately dilated and show a poor or absent light reaction but preserve the near reaction. It has been suggested that light reflex fibres are more dorsal in the midbrain and are therefore selectively damaged by lesions in this area with comparative sparing of the more ventrally sited near reflex pathways.