CHAPTER 104 Neural Scarring
INTRODUCTION
Of the myriad causes of failed back surgery syndrome (FBSS), neural scarring has been reported to be the cause of failed back surgery syndrome in 6–24% of patients.1–7 In 1996, Ross et al., using a prospective, controlled, randomized, blinded, multicenter methodology, demonstrated a significant association between the gross amount of peridural scar and the occurrence of recurrent radicular pain; those with extensive epidural scarring were 3.2 times more likely to experience recurrent radicular pain than those with less scarring.7 The degree of postoperative scarring relates to the extent and magnitude of the surgery. It is undeniable that the presence of postoperative scar tissue is not solely due to the operative trauma, but is also generated as a reparative response to the herniated disc material as not all patients with scarring are symptomatic.8 Although epidural adhesions are most commonly present following spine surgery, leakage of disc material from a herniated nucleus pulposus or annular disc tear can cause an inflammatory response with fibrocyte deposition and resultant epidural adhesions in the absence of surgery.9,10 There are those who believe that neural scarring such as epidural and/or intraneural fibrosis do not ever cause symptoms. This school of thought is based upon the fact that neural scarring is a normal bodily response to injury and that all postoperative patients will have some degree of scarring, the majority of which are asymptomatic.8 However, just as there are symptomatic and asymptomatic disc protrusions, the same tenet holds true for epidural fibrosis and neural scarring. The fact that not all focal disc protrusions or epidural fibrosis, as demonstrated by magnetic resonance imaging (MRI), are clinically symptomatic does not prove that focal disc protrusions or epidural fibrosis are not pain generators and have no relationship to low back or lower limb pain preoperatively or postoperatively. Such a view is simplistic and is more a function of the lack of an effective clinical pathway in the diagnosis of symptomatic neural scarring. Historically, epidural fibrosis or arachnoiditis was an uncommon clinical entity prior to the introduction of lumbar spine surgery for the treatment of degenerative spine conditions.11 A large number of reports of epidural fibrosis found on repeat surgery led to the association of recurrent symptomatology with perineural scarring.11–13 This chapter will offer a diagnostic algorithmic approach to determine an accurate diagnosis of symptomatic neural scarring. A review of the clinical anatomy of the nerve root and its anatomic relationships is critical to understanding the basis and pathophysiology of symptomatic neural scarring.
ANATOMY
Sunderland14 described the connective tissue coverings of the nerve fiber. Each axon is surrounded by an endoneurial sheath in which multiple axons or nerve fibers form a funiculi. The endoneurial sheath resists elongation under tension. Each funiculi is invested by perineurial connective tissue. Multiple funiculi are surrounded by the epineurium which consists of alveolar connective tissue. The outer perinerium and epineurium provide some degree of protection from tensile and compressive forces (Fig. 104.1). The portions of the nerve roots which lack this outer sheath are more vulnerable to traction or compression. When there is nerve injury, fibrotic tissue may form perineurally/epidurally or intraneurally. It is important to understand the relationship between the nerve roots and the intervertebral foramen (IVF). Each intervertebral foramen is in the shape of an inverted pear that is bounded laterally by the pedicle, posteriorly by the articular facets and ligamentum flavum, and anteriorly by the intervertebral disc and vertebral bodies.15 The radicular complex of ganglia, nerve roots, spinal nerve, and surrounding sheath accounts for 20–35% of the cross-sectional area of the IVF (Fig. 104.2).15 The remainder of the IVF is occupied by loose areolar or adipose tissue, a radicular artery, and numerous venous channels that often encircle the nerve roots. The depth of the foramen varies from 4 to 9 mm.15 The lumbar nerve root complex is situated toward the upper pole of the foramen. At and beyond the ganglion, the dura loses its identity and forms the outer connective tissue sheath which represents the epineurium and perineurium of the ganglion, anterior nerve root, and the newly formed spinal nerve. The Hofmann ligaments, initially described in 1898, are fine filamentous bands of connective tissue that connect the ventral surface of the dural sac and the exiting nerve roots to the posterior longitudinal ligament and posterior vertebral periosteum (Fig. 104.3).16 The ventral ligamentous attachments within the spinal canal reduce the ability of a nerve root to be dorsally displaced by a disc herniation. The nerve root complex is mobile, but does not have unlimited motion. The lumbar roots undergo an excursion of 0.5–5 mm, depending on the anatomic level.17 There is an average excursion of 3 mm for the intrathecal portion of the L5 nerve root, while for the S1 nerve root this value ranges between 4 and 5 mm.17 Movement is induced by straight leg raising in the lumbosacral roots, nerves, and plexus, and in the intrapelvic section of the sciatic nerve.17 Since the nerve root and its dural sheath are relatively fixed within the spinal canal and the radicular foramen, they cannot easily slip away from a disc protrusion. Fibrosis and adhesions may impair the gliding capacity of the lumbar nerve roots within the radicular canals and increase the vulnerability of the extrathecal intraspinal nerve root to compression. Consequently, the nerve root is stretched and compressed, resulting in edema, ischemia, and radicular symptoms.
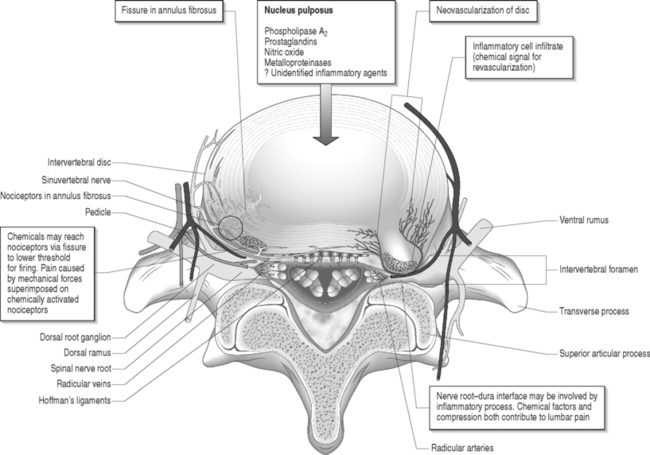
Fig. 104.2 Nerve root complex with dorsal root ganglion, nerve root, and its arterial and venous blood supply.
ADHESIVE ARACHNOIDITIS
Arachnoiditis is inflammation of the pia–arachnoid membrane that covers the spinal cord, cauda equina, nerve roots, or a combination. The extent of scarring varies from mild membrane thickening to severe scarring that can obliterate the subarachnoid space and obstruct cerebrospinal fluid (CSF) flow. The etiology of arachnoiditis is unclear, but typically the intrathecal contents are exposed to an inciting agent such as lumbar spine surgery, intraoperative dural tears, postoperative infections, intrathecal pharmacologic medications, and injection of oil-based contrast agents. Arachnoiditis is more common in patients who undergo extensive or bilateral surgical procedures, and repeat surgeries.
Pathophysiology
Adhesive arachnoiditis causes proliferation of soft tissue leading to filmy adhesions and later to a dense fibrotic matrix. Inflammatory changes are seen microscopically. It is thought that the severity and duration of arachnoiditis is directly correlated to the degree of inflammation and tissue remodeling associated with wound healing. A vascular etiology has also been proposed whereby venous obstruction and dilatation leads to endothelial damage, fibrin deposition, intravascular thromboses, and ultimately fibrosis of the neural elements.18 In the subarachnoid space, the nutritional support of nerve roots is dependent upon its limited vascular supply and the circulation of CSF. The tenuous blood supply and nutrition to the nerve roots within the subarachnoid space can easily be interrupted by neural scarring with subsequent ischemia possible. The exact mechanism of adhesive arachnoiditis is still unknown.
EPIDURAL AND INTRANEURAL FIBROSIS
Epidural fibrosis refers to scar tissue formation outside the dura, on the cauda equina or directly on the nerve roots. Epidural fibrosis develops when epidural fat is replaced by the hematoma that typically forms in the path of surgical dissection. The hematoma is gradually absorbed and simultaneously replaced by granulation tissue that matures into fibrotic connective tissue. Scar tissue development in postoperative patients is not solely due to the operative trauma, but is also a physiologic and reparative response to a herniated disc.8 Epidural scar tissue may constrict the neural elements and cause postoperative pain. Intraneural fibrosis is the formation of scar tissue within the nerve root as opposed to epidural fibrosis, which refers to scar tissue formation outside the nerve root or directly on the nerve root. Intraneural fibrosis cannot be detected by any imaging study and can only be confirmed by pathologic examination under microscopy.
Pathophysiology
Mechanical compression
Nerve fibers encased in collagenous scar tissue suffer an increase in neural tension, impairment of axoplasmic transport, and restriction of arterial supply and venous return. Spinal nerve roots and dorsal root ganglia are particularly sensitive to mechanical deformation due to intraspinal disorders.19 The importance of axonal stretching and deformation in response to a mechanical force and the consequent morphological changes within the nervi nervorum were first described by Horsley et al. in 1883.20 A chronic compressive force decreases blood perfusion causing ischemia and limits CSF circulation which leads to endoneural hyperemia21 and further intraneural damage.22 Since a nerve root lacks a perineurium and has a poorly developed epineurium, it may be more susceptible to compression from intraspinal disorders such as epidural fibrosis or a disc herniation. It has been shown that compression of a peripheral nerve will induce direct mechanical effects on the nerve tissue such as deformation of nerve fibers, displacement of nodes of Ranvier, decreased intraneural microcirculation, and neurophysiologic changes of conduction block. Olmarker et al.19 reported increased permeability of the endoneurial capillaries of the nerve roots resulting in edema from mechanical compression. Intraneural edema may impair capillary blood flow and compromise nutritional transport, causing ischemia. Compression and edema may lead to intraneural fibrosis. These processes have important implications as intraneural damage causes inflammation and eventual fibrotic tissue formation after healing leading to intraneural fibrosis. Similarly, perineural or epidural fibrotic tissue in response to surgical trauma or part of the normal reparative process, in response to inflammation or vascular compromise, may mechanically compress the nerve roots and initiate the above cascade, leading to intraneural fibrosis. Tension or stretching of nerve segments may lead to similar anatomic alterations. This may occur if a segment of the spinal nerve root is fixed (e.g. intervertebral foramen) or tethered by fibrotic epidural scar tissue (Fig. 104.4.) Ebeling et al.2,23 demonstrated nerve root immobilization as a result of epidural fibrosis causing nerve root impingement from small recurrent disc fragments embedded in fibrotic tissue. This finding occurred in 23% of cases during second operation following lumbar disc surgery in 92 patients.
Inflammatory etiology
Marshall24 suggested that leakage of breakdown products from degenerating nucleus pulposus may induce a chemical radiculitis. In animal studies, Bobechko and Hirsch25 demonstrated an autoimmune response to the nucleus pulposus of rabbits. This was confirmed by Gertzbein et al.26 in 1975. Using human nucleus pulposus tested with rabbit sera, they demonstrated the presence of a cellular immune response in 73% of patients whose discs were found to be sequestered at the time of surgery and 26% in those patients whose discs were herniated.
McCarron et al.9 showed that there was an inflammatory response to nucleus pulposus injectate in the epidural fat and dura. Saal et al.27 demonstrated that high concentrations of phospholipase A2, the rate-limiting enzyme for the inflammatory cascade of prostaglandins and leukotrienes, were present in human lumbar herniated nucleus pulposus and degenerative discs. Franson et al.28 reported that extracted PLA2 form human lumbar disc has powerful inflammatory activity in vivo. Byrod et al.29 reported that epidural application of substances can have a direct transport route to the axons of the spinal nerve roots. PLA2 may act to excite nociceptors within the anulus or within the epidural space. Direct contact with a nerve root may cause neural injury either by enzymatic activity on the membrane phospholipids or by production of inflammatory mediators. Human disc PLA2 has been shown to cause perineural inflammation, conduction block, and axonal injury by extrathecal application to animal nerve.30 Leakage of PLA2 or another neurotoxic chemical within the disc may irritate small unmyelinated nerve fibers in the anulus or nearby structures such as spinal nerve roots. It has been suggested that radicular pain results from the ectopic firing within sensory fibers injured by the inflammatory chemical mediators released from degenerated disc tissue.31,32 Perineural inflammation or demyelination induced by phospholipase A2 may be responsible for the hypersensitivity of a nerve root to mechanical stimulation.33
Herniated intervertebral disc material can cause inflammatory changes which may lead to epidural or intraneural fibrosis. It has also been suggested that ventrally located epidural scar tissue that adheres to the dorsal aspect of the disc can create injury via mechanical tension followed leakage of inflammatory enzymes.34
Vascular etiology
Disc degeneration has been known to be closely associated with abnormalities in the anatomy and physiology of the adjacent nerve roots. Holt and Yates35 correlated the histology of cervical disc degeneration with adjacent spinal nerve root fibrosis in their cadaver study. Lindahl and Rexed36 demonstrated intraneural fibrosis in 78% of dorsal nerve root biopsies taken at the time of surgery in patients operated on for herniated intervertebral discs. Jayson37 found that there is a statistically significant relationship between the extent of disc degeneration and prolapse with evidence of epidural venous obstruction, perineural/intraneural fibrosis, focal demyelination and neuronal atrophy. A fibrinolytic defect was found in patients with back pain, suggesting that a decreased ability to clear fibrin may be responsible for chronic tissue damage.38 Epidural fibrotic tissue may induce vascular compromise and ischemia with resultant intraneural fibrosis (Fig. 104.5).
DIAGNOSIS
Imaging
Gadolinium-enhanced MRI is the imaging study of choice when evaluating for epidural fibrosis.7,34 Epidural fibrosis can only be seen on MRI performed with gadolinium dye. Gadolinium enhances vascularized fibrotic scar tissue and distinguishes epidural fibrosis from a recurrent disc protrusion in a postoperative spine patient. MRI is the imaging study of choice in diagnosing arachnoiditis and differentiating other causes of FBSS such as epidural fibrosis, retained or recurrent disc protrusion, lateral recess stenosis, and infection.7,34 MRI has demonstrated excellent correlation with CT-myelography in the diagnosis of adhesive arachnoiditis without the additional risks of an intrathecal injection.39 The characteristic central clumping or clustering of nerve roots within the thecal sac is best seen on axial T2-weighted images (Fig. 104.6).40,41 Peripheral adhesions or severe thecal sac distortion can also be seen. These findings are due to the adhesive nature of the inflamed pia–arachnoid membranes. There is still an occasional role for CT-myelography in a patient with clinically suspected adhesive arachnoiditis and a normal MRI. The ability of CT-myelography to delineate the intrathecal nerve root anatomy may be useful in those patients with limited, localized areas of arachnoiditis involvement.42 It is important to note that findings consistent with arachnoiditis on an imaging study may be clinically asymptomatic.
Clinical assessment
The diagnosis of postoperative low back and limb pain due to neural scarring is a diagnosis of exclusion. Any possible cause for the radicular symptoms other than scar formation has to be excluded.43 The common etiologies of FBSS must be algorithmically eliminated. This concept has been discussed by Jerome Schofferman in the preceding chapter.