Chapter 89 Natural and Human-Made Hazards
Disaster Risk Management Issues
For online-only figures, please go to www.expertconsult.com
Disaster Risk Reduction and Management
Selection of management options depends on the type of hazard and its characteristics. Box 89-1 lists the elements usually found in a disaster preparedness plan for sudden-onset hazards, such as earthquakes, tsunamis, volcanic eruptions, tropical cyclones, and floods. Preparedness measures for slow-onset disasters, such as drought, include early warning systems that alert authorities to precursory conditions and allow preparations to avert food and water shortages.
BOX 89-1 Essentials of a Preparedness Plan for Rapid Onset Disasters
Disaster Mitigation Strategies
Earthquakes
Causal Phenomena
Faults are areas of stress at plate boundaries that release accumulated energy by slipping or rupturing. Elastic rebound occurs when the maximum point of supportable strain is reached and a rupture occurs, allowing the rock to rebound until the strain is relieved (Figure 89-1). Usually, the rock rebounds on both sides of the fault in opposite directions. The point of rupture is called the focus and may be located near the surface or deep below it. The point on the surface directly above the focus is termed the epicenter (Figure 89-2).
Characteristics
There are three types of seismic waves. The body waves (P, or primary, and S, or secondary) penetrate the body of the earth, vibrating quickly (Figure 89-3). P waves travel at about 6 km per second (kps) (3.7 miles per second [mps]) and provide the initial jolt that causes buildings to vibrate up and down. S waves travel about 4 kps (2.5 mps) in a movement similar to the snap of a whip, causing a sharper jolt that vibrates structures from side to side and usually resulting in the most destruction. Surface waves (L waves) vibrate the ground horizontally and vertically and cause swaying of tall buildings, even at great distances from the epicenter.
Earthquake Scales
The earthquake intensity scale measures the effects of an earthquake where it occurs. The most widely used scale of this type is the modified Mercalli scale, which expresses the intensity of earthquake effects on people, structures, and the earth’s surface in values from I to XII (Table 89-1). Another, more explicit, scale used in Europe is the Medvedev-Sponheuer-Karnik (MSK) scale.
Scale | Description |
---|---|
I | Not felt except by very few persons under especially favorable circumstances. |
II | Felt only by a few persons at rest, especially on upper floors of buildings. Delicately suspended objects may swing. |
III | Felt quite noticeably indoors, especially on upper floors of buildings, but many people do not recognize it as an earthquake. Standing motor vehicles may rock slightly. Vibration similar to passing of truck. Duration estimated. |
IV | During the day felt indoors by many but outdoors by few. At night some awakened. Dishes, windows, doors disturbed; walls make creaking sound. Sensation resembles heavy truck striking building. Standing motor vehicles rocked noticeably. |
V | Felt by nearly everyone; many awakened. Some dishes, windows, etc., broken. A few instances of cracked plaster. Unstable objects overturned. Disturbances of trees, poles, and other tall objects sometimes noticed. Pendulum clocks may stop. |
VI | Felt by all; many frightened and run outdoors. Some heavy furniture moved; a few instances of fallen plaster or damaged chimneys. Damage slight. |
VII | Everybody runs outdoors. Damage negligible in buildings of good design and construction, slight to moderate in well-built ordinary structures, considerable in poorly built or badly designed structures. Some chimneys broken. Noticed by persons driving motor vehicles. |
VIII | Damage slight in specially designed structures, considerable in ordinary substantial buildings with partial collapse, great in poorly built structures. Panel walls thrown out of frame structures. Fall of chimneys, factory stacks, columns, monuments, and walls. Heavy furniture overturned. Sand and mud ejected in small amounts. Changes in well water. Persons driving motor vehicles disturbed. |
IX | Damage considerable in specially designed structures. Well-designed structures thrown out of plumb, greatly in substantial buildings with partial collapse. Buildings shifted off foundations. Ground cracked conspicuously. Underground pipes broken. |
X | Some well-built wooden structures destroyed. Most masonry and frame structures with foundations destroyed; ground severely cracked. Rails bent. Landslides considerable from river banks and steep slopes. Shifted sand and mud. Water splashed (slopped) over banks. |
XI | Few, if any, (masonry) structures remain standing. Bridges destroyed. Broad fissures in ground. Underground pipelines completely out of service. Earth slumps and land slips in soft ground. Rails bent greatly. |
XII | Damage total. Practically all works of construction are damaged greatly or destroyed. Waves seen on ground surface. Lines of sight and level are distorted. Objects are thrown upward into the air. |
Location and Predictability
Earthquake Hazards
Typical Adverse Effects
The January 2010 Haiti earthquake affected an estimated 3 million people. It killed approximately 100,000 persons and injured approximately 300,000, although estimates of casualties widely vary. More than 1 million Haitians were left homeless. Vital infrastructure necessary to respond to the disaster, including air, sea, and land transport facilities and communication systems, was severely damaged or destroyed. Treatment of the injured was hampered by the lack of hospitals and morgue facilities; bodies were left to decay on the streets for many days. International assistance was offered in abundance, but the logistical capabilities in Haiti to receive emergency aid were limited. Doubtless, more lives were lost as a result of this vulnerability (Figures 89-4 and 89-5, online).
Earthquake Risk Reduction Measures
Tsunamis
Causal Phenomena and Characteristics
The geologic movements that cause a tsunami are produced in three major ways (Figure 89-7). The foremost cause is fault movement on the sea floor, accompanied by an earthquake. The second most common cause is a landslide occurring underwater or originating above the sea and then plunging into the water. The highest tsunamis ever reported were produced by a landslide at Lituya Bay, Alaska, in 1958. A massive rock slide produced a wave that reached a high water mark of 530 m (1740 feet) above the shoreline. A third cause of a tsunami is volcanic activity, which may uplift the flank of the volcano or cause an explosion.
Vulnerability
The following major factors contribute to vulnerability to tsunamis:
Typical Adverse Effects
The force of water in a bore, with pressures up to 10,000kg/m2, can raze everything in its path. The flooding from a tsunami, however, affects human settlements most, by water damage to homes, businesses, roads, and infrastructure. Withdrawal of the tsunami also causes significant damage. As the water is dragged back toward the sea, bottom sediments are scoured out, collapsing piers and port facilities and sweeping out foundations of buildings. Entire beaches have disappeared, and houses have been carried out to sea. Water levels and currents may change unpredictably, and boats of all sizes may be swamped, sunk, or battered (Figures 89-8 to 89-10; Figures 89-8 and 89-10, online).
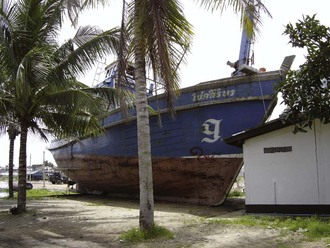
FIGURE 89-8 Ship washed ashore in tsunami, December 2004, in Koh Lanta, Thailand.
(Courtesy Sheila B. Reed.)
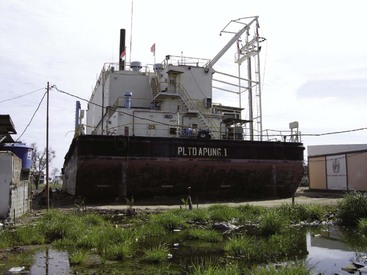
FIGURE 89-10 Generator ship washed ashore 2.3 miles inland, near Banda Aceh, Indonesia.
(Courtesy Sheila B. Reed.)
Casualties and Public Health
Deaths occur principally from drowning as water inundates homes or neighborhoods. Many people may be washed out to sea or crushed by the giant waves. Injuries occur from battering by debris. Little evidence exists of tsunami flooding directly causing large-scale health problems. Rapid effective assistance to the Banda Aceh (Indonesia) area in early 2005 prevented widespread outbreaks of disease in displacement camps (Figure 89-11). Malaria mosquitoes may increase because of water trapped in pools. Open wells and other groundwater may be contaminated by salt water and debris or sewage. Normal water supplies may be inaccessible for days because of broken water mains.
Volcanic Eruptions
A volcano is a vent or chimney to the earth’s surface from a reservoir of molten rock, called magma, deep in the earth’s crust (see Chapter 15). Approximately 500 volcanoes are active (have erupted in recorded history), and many thousands are dormant (could become active again) or extinct (are not expected to erupt again). On average, about 50 volcanoes erupt every year; only about 150 are routinely monitored. Since 1000 AD, more than 300,000 people have been killed directly or indirectly by volcanic eruptions, and currently about 10% of the world’s population lives on or near potentially dangerous volcanoes.
Types
Volcanic eruptions may be described as follows in descending order of intensity (Figure 89-12).
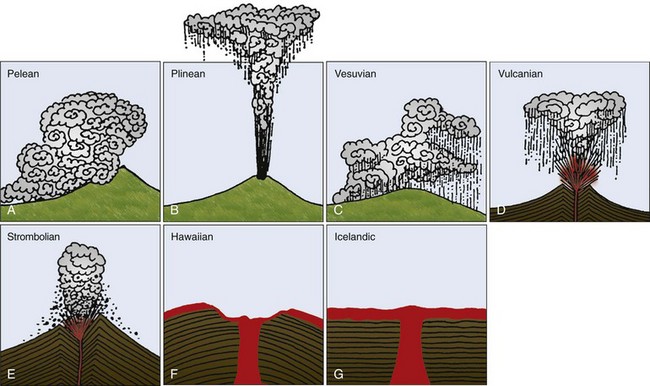
FIGURE 89-12 Eruption types. A, Pelean. B, Plinean. C, Vesuvian. D, Vulcanian. E, Strombolian. F, Hawaiian. G, Icelandic.
(Modified from United Nations Development Program: Introduction to hazards, ed 3, Disaster Management Training Programme, UN Organization for Coordination of Humanitarian Assistance, New York, 1997.)