Chapter 131 Motor Cortex Stimulation for Intractable Facial Pain
In the early 1990s, Tsubokawa and colleagues first reported using electrical stimulation of the primary motor cortex to alleviate post-stroke thalamic pain.1–3 The basic approach of motor cortex stimulation (MCS) is to implant an array of stimulating electrodes over the primary motor strip in the region corresponding to the territory of painful neuropathy. Chronic, intermittent electrical stimulation is then delivered at intensities below the threshold for evoking a motor response. MCS outcomes have been variable, but application of the technique to TNP has yielded success rates of 75% to 100% in many series4–20 (see Table 131-1). Despite a paucity of controlled trials, MCS has evolved into a standard therapy for disabling TNP at many centers, offering a palliative “last resort” for patients who have exhausted other avenues of treatment.
Historical Perspective
The notion that the motor cortex contributes to pain processing dates to observations made by Penfield in the first half of the 20th century. Operating on epilepsy patients, he recognized that electrical stimulation of the motor strip could elicit sensory responses.21 When a patient with chronic burning pain experienced a relapse in symptoms following a somatosensory cortex resection, Penfield ventured to resect the precentral gyrus. This yielded a complete—albeit temporary—abatement of the patient’s pain.21,22 Three of Penfield’s patients later achieved long-term relief from intractable facial pain with combined resections of the pre- and post-central gyri. Lende and Kirsch adapted Penfield’s approach in two patients with intractable face pain, removing both the precentral and postcentral areas of facial representation.23 Although apparently effective, this aggressive strategy has not been reported since.
The 1970s heralded the introduction of non-lesional techniques—notably deep brain stimulation (DBS)—for mitigating chronic pain. DBS pain targets were typically the periaqueductal or periventricular gray matter or the sensory thalamus. Success rates reported in the literature were inconsistent, and no sizeable controlled trials were conducted for decades. At present, the precise role and efficacy of DBS for chronic pain syndromes remain subjects of debate.24–26
In the early 1990s, Tsubokawa shifted the focus of pain circuit modulation toward cortical entry points. His work was motivated by animal models of deafferentation pain in which bursting hyperactivity in thalamic neurons could be inhibited by stimulation of the motor strip. In 1991, Tsubokawa and colleagues described a series of seven thalamic pain syndrome patients, each of whom realized “excellent or good” long-term pain relief with MCS.2 Subsequent series revealed that TNP patients tended to fare better with MCS than counterparts with neuropathic pain in other distributions.8,11,27
Mechanism of Action
The mechanisms underlying pain modulation by MCS remain speculative.28–31 Evidence from a range of modalities has underscored that an extensive neural circuit is dysfunctional in chronic pain syndromes.32–34 While the role of MCS in suppressing the symptoms of chronic pain syndromes is as yet unclear, experiments in both animals and humans have offered insight.
Microelectrode recordings in deafferentation animal models and humans with central pain syndromes have demonstrated that thalamic neurons manifest spontaneous bursting patterns.2,35–41 Deafferented thalami have an altered somatotopy, and microstimulation of these thalami can elicit “central allodynia”—a phenomenon in which the usual sensations evoked by stimulation are replaced by painful sensations similar to those seen clinically in pain syndromes.35,38 These lines of evidence led some to implicate thalamic hyperactivity as an engine driving the manifestations of central pain.
Concurrently, a picture was emerging that electrical stimulation of the motor cortex could suppress thalamic hyperactivity.2,36,42 At that time, there were already precedents for the hypothesis that cortical stimulation could mitigate pain. Animal studies dating to the 1950s had established that stimulation of sensorimotor cortex diminishes spinal cord afferent activity43 as well as dorsal horn activity.44 MCS also elevated nociceptive response latency45,46 and has since been shown to selectively attenuate dorsal horn responsiveness to high-intensity painful stimuli.47
Tsubokawa’s initial work was based on the hypothesis that MCS inhibits deafferented nociceptive neurons,1–339 perhaps by activating (both anti- and ortho-dromically) a mesh of superficial reciprocal fibers carrying non-nociceptive signals between the motor cortex and both adjoining somatosensory cortex and the sensory thalamus.30,32,48–52 Indeed, recent transcranial magnetic stimulation studies have suggested that chronic pain syndromes may be associated with defective intracortical inhibition, and that MCS restores this inhibition.32,51 Activation of cortical interneurons might reestablish a balance of normal non-nociceptive dominance among systems of hyperactive nociceptors, thereby effecting analgesia.29,41,53
Positron emission tomography (PET) studies permit interrogation of changes in regional cerebral blood flow (CBF) that attend MCS. Patterns of CBF redirection during (and after) MCS reflect modulation of a functional pain network that includes the anterior cingulate cortex, orbitofrontal cortex, medial thalamus, and periaqueductal and periventricular gray matter.54,55 At baseline, there is relative decrement in thalamic CBF in chronic pain patients, and this decrement is reversible with MCS.29,56,57 Analgesic effect parallels the degree of increase in anterior cingulate gyrus CBF,29,31,57–60 perhaps suggesting that MCS may blunt the affective or “suffering” component of chronic pain, a process in which this cortical region has a documented role.61 Regions hemodynamically activated by MCS retain their activation for 30 minutes or more after stimulation is halted,29,31 reminiscent of observations that MCS-associated analgesia may persist for hours after a stimulator has been turned off.3,62
Finally, some have suggested that analgesia can be ascribed to MCS-triggered neurochemical release. For example, Maarrawi et al. demonstrated that MCS enhances secretion of endogenous opioids—particularly in the anterior middle cingulate cortex and periaqueductal gray matter—in correlation with degree of analgesia.63
Indications
Importantly, the clinician should distinguish patients with TNP from those with classical trigeminal neuralgia, the latter disorder being characterized by episodic sharp, lancinating pains in a trigeminal distribution (although the taxonomy of these entities remains a point of contention64). Invasive treatments considered effective for trigeminal neuralgia may in fact intensify the symptoms of TNP. It is our practice to pursue conventional medical and neurosurgical therapies (reviewed elsewhere65–67) for trigeminal neuralgia before resorting to MCS. Of course, trigeminal neuralgia may evolve into a form of TNP. This often occurs in patients who fail neurosurgical interventions for trigeminal neuralgia; for example, rhizotomy patients may develop anesthesia dolorosa—a form of TNP. Under such circumstances, MCS represents an appropriate therapeutic option.
Outcomes
Historically, MCS has been applied to TNP more successfully than to any other neuropathic pain syndrome. One factor cited to account for this disparity is that the face is represented by a large territory of the motor strip on the accessible lateral convexity, meaning that the electrode can be placed with relative ease and consistency. Most MCS studies regard a 50% reduction in baseline visual analog scale (VAS) pain ratings as a threshold for a positive clinical response. Given this criterion, literature reviews cite composite TNP long-term response rates of 60% to 80%.4,68–71 A summary of individual MCS case series (with the number of TNP patients in each) is shown in Table 131-1.
While reports of patient follow-up beyond 2 to 3 years are uncommon, many investigators have noted that the clinical benefit of MCS may decline slowly with time, perhaps due to remodeling of cortical architecture. Anecdotal reports have suggested that a washout or “no-stimulation” period followed by stimulator reprogramming may help to regain lost clinical milestones. Henderson et al. found that intensive reprogramming could indeed yield clinical improvement; potential benefit, however, should be weighed against the risk of inducing seizures.72
Although a majority of TNP patients receiving MCS achieve some measure of pain relief, it not clear why many suitable candidates fail to respond. To aid in patient selection, several investigators have attempted to correlate a variety of factors with MCS responsiveness. Yamamoto et al. reported that pain reduction with infused pharmacologic agents predicted pain relief by MCS; success was correlated with sensitivity to thiamylal and resistance to morphine.73 Katayama and colleagues underscored that integrity of the corticospinal tract is critical to MCS success; 15% of patients in one series achieved adequate pain relief when moderate or dense motor weakness existed in the affected territory and only 9% benefited when no motor contractions could be elicited (vs. a 73% success rate when motor impairment was absent or mild74). Analgesic effect has long been postulated to depend upon accurate placement of the stimulating electrodes.3,11 Nuti et al. found that pain relief at the conclusion of the first month of stimulation was a predictor of long-term relief.62 Finally, pain relief by repetitive transcranial magnetic stimulation may predict response to MCS.75,76
There have been no large controlled, prospective trials evaluating MCS for facial pain (or for any other chronic pain syndrome). A recent literature review identified reports of only 44 nonredundant cases of MCS for TNP.69 This is compounded by the fact that existing case series are heterogeneous with respect to patient population, inclusion criteria, surgical technique, stimulation parameters, outcome assessment, and a host of other factors. Concerns have also been raised of a possible bias toward the reporting of only case series with positive outcomes69 (see discussion of Brown and Pilitsis4). MCS is well-suited for randomized crossover trials with sham stimulation, as the stimulation rarely generates any perceptible sensation. And yet, few investigators have invoked this paradigm. The neurosurgical community now confronts a scenario similar to that seen after the initial reports of DBS for pain: An emerging technique is gradually adopted into widespread use without a rigorous vetting process. While MCS represents a promising therapy for TNP patients who have exhausted traditional treatment strategies, it merits formal interrogation in prospective, controlled trials.
Preoperative Considerations
Preoperative planning begins with identification of the central sulcus and subsequently the face motor region. There are a variety of anatomic landmarks that can assist in identifying the central sulcus (Fig. 131-1).77–80 The central sulcus is immediately posterior to the precentral sulcus, which in turn marks the termination point of the horizontally oriented superior and inferior frontal sulci. The precentral sulcus is sometimes distinguished by an interruption by the middle frontal gyrus, but the central sulcus is usually continuous. The omega-shaped knob, best seen on axial images, marks the hand motor region, and its posterior boundary is the central sulcus (Fig. 131-D).80 This same region has a hook-shaped appearance in sagittal section (Fig. 131-1B). On sagittal images of the mesial wall of the hemisphere, the central sulcus can also be identified as the notch immediately anterior to the termination of the marginal branch of the cingulate sulcus at the vertex (Fig. 131-1E and F).78 In addition, the cortical thickness of the anterior (motor) bank of the central sulcus is approximately twice that of the posterior (sensory) bank on T2-weighted MRI images.77
Once the central sulcus and primary motor cortex have been identified, the face motor region can be determined. Early work has shown that the optimal stimulation target is in the precentral gyrus, approximately at the level of the inferior frontal sulcus.20 Due to individual variations in functional anatomy, however, other means of verifying the face representation within the motor strip can be valuable. Some studies have advocated for the use of transcranial magnetic stimulation (TMS) for this purpose. In TMS, a strong rapidly alternating magnetic field is focused on a cortical region, inducing a local current loop. Placement of the stimulator over the face motor cortex produces contractions of the contralateral face musculature. A few studies have demonstrated some analgesic benefit to patients with TNP from repetitive TMS (rTMS),81,82 and response to rTMS may predict a corresponding response to MCS.71
Individual localization can also be performed with functional MRI (fMRI). Blood oxygenation level–dependent (BOLD) fMRI, the most common form of the technique, relies on changes in local tissue oxygenation due to the hemodynamic response to increased neuronal activity. Voluntary tongue movement is a robust activator of the face motor region on fMRI. The fMRI can be co-registered with the patient’s structural MRI (Fig. 131-2) and imported into the intraoperative navigation system. We feel that using fMRI to help identify the face motor region is a helpful adjunct in preoperative planning and intraoperative navigation during MCS surgery, and others have also documented its utility.19
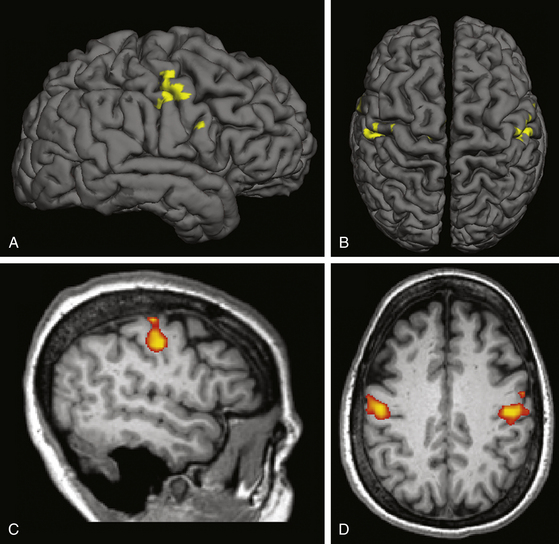
FIGURE 131-2 Functional MRI for face motor localization. This figure depicts an fMRI activation produced by face movement (volitional smiling) of the same patient as in Fig. 131-1. As is typical, the activation is centered within the depths of the central sulci bilaterally. A and C, Lateral surface and sagittal slice, respectively, of the right hemisphere. B and D, Vertex view and axial slice (in radiologic convention), respectively. The fMRI response can be superimposed on a surface registration of the patient’s brain, permitting individual localization of the target.
Operative Technique
Original reports relied on anatomic localization and scalp somatosensory-evoked potential (SSEP) mapping, with placement of the stimulating electrode through a burr hole.3,11 Performing a small craniotomy for more extensive epidural SSEP and motor mapping was introduced in the mid-1990s.6 The addition of more refined localization techniques, including intraoperative navigation13,27 and preoperative functional imaging,19 has further improved targeting and, correspondingly, outcomes. Although variations certainly exist, the operative technique from our institution described below is representative of the current practice.
At the time of surgery, the patient’s preoperative MRI is loaded into the intraoperative navigation system, along with the fMRI, if available. The patient is positioned supine on the operating room table. The head is turned to the side, with the aid of a shoulder roll if necessary, and affixed with a three-pin head frame. We favor the use of general anesthesia,18,19,83 but the procedure can be performed under local anesthesia.3,11,12,15,17,27,84 The MRI is registered to the patient’s head using fiducial markers mounted on the head frame, and the target region re-confirmed.
Using stereotaxy as a guide, the skin incision is planned. The incision should be generous enough to accommodate the craniotomy and leave room for the connection between the electrode and the lead to the pulse generator. We typically perform an approximately 8-cm curvilinear incision along the superior temporal line, from just posterior to the hairline to 2 to 3 cm posterior to the ear. Location of the motor strip is reconfirmed with the navigation system to plan the craniotomy. A burr hole is placed along the border of the planned craniotomy site and the dura stripped carefully using a Penfield #3 instrument. A foot-plated bit is then used to circumscribe the craniotomy, and the bone flap elevated (Fig. 131-3).
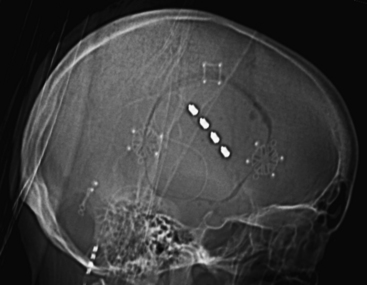
FIGURE 131-3 Craniotomy and electrode placement. The same patient depicted in Figs. 131-1 and 131-2 underwent right craniotomy for MCS placement. This lateral scout sequence of a head computed tomography scan depicts the size and location of a typical craniotomy employed in our institution for MCS. The burr hole is placed at the border of the planned craniotomy, and a foot-plated bit is used to circumscribe the craniotomy. A four-contact paddle electrode is placed epidurally over the face region of motor cortex, as identified by scalp somatosensory-evoked potential and motor mapping. The electrode is placed roughly parallel to the central sulcus. The attached lead exits through the burr hole and is connected to the pulse generator. MCS, motor cortex stimulation.
The central sulcus and motor strip can be mapped using epidural SSEP and/or motor mapping.85 SSEP mapping takes advantage of the phase reversal in SSEPs across the central sulcus. During contralateral median nerve stimulation, the 20-millisecond–latency SSEP recorded by epidural electrodes reverses phases from a positive precentral peak to a negative postcentral peak across the central sulcus (N20–P20 phase reversal).86 In epidural motor mapping, an electrical stimulus is applied to the dura overlying the putative motor cortex. Rhythmic contractions of the contralateral face musculature confirm location over the face region of motor cortex.
When the target region has been satisfactorily identified, the electrode is positioned such that it spans the target region. We typically use a four-contact paddle-style electrode (Resume, Medtronic, Inc., Minneapolis, MN), as have others.4,10,12,15,17,19,70,83,87 Although some have placed the electrode perpendicular to the central sulcus,27,71 we have found that placement parallel to the central sulcus, along the motor strip, increases the likelihood that a pair of contacts will overlie the optimal region (Fig. 131-3). The electrode is secured in position with 4-0 Neurolon sutures.
The stimulating electrodes are typically placed epiduraly, as described here, for increased safety and decreased operative time. In contrast, some authors argue for subdural placement, citing the lower stimulation intensity requirement and concomitant greater battery life, as well as decreased chance of eliciting discomfort via stimulation of dural pain fibers.70,83 Subdural placement, however, does carry an increased risk of causing hemorrhage.88
Staged Procedure for Externalized Testing
Some surgeons perform a staged procedure to permit testing of the device’s efficacy before it is permanently implanted.4,12,15,17,18,27,70,84 In this variation, a multicontact electrode grid (usually 16–20 contacts) is placed over the target region. The leads are tunneled but then externalized and secured to the skin exit site. Over the next several days the patient remains in the hospital, and electrical stimulation is applied to varying combinations of electrodes to determine whether the patient is likely to obtain adequate analgesia. Patients attaining some criterion degree of pain reduction (e.g., ≥50% reduction in VAS score) proceed to internalization of the leads, and connection to an infraclavicular pulse generator. Those who do not experience significant analgesia can be explanted, as the risk of instrument-related complication in those patients would likely outweigh any potential benefit.
Stimulation Regimen
There is a great deal of institutional variability regarding optimal stimulator settings. As is the case for any neuromodulatory device, however, it is clear that an individualized approach is required. Also accepted is the notion that adequate pain control should be achieved at stimulation intensities below the threshold for involuntary muscle contraction. A wide range of stimulation frequencies (15–130 Hz), pulse widths (60–500 microseconds), and intensities (0.5–10.5 mA) have been successfully employed.59 Pain relief typically begins minutes after stimulation onset, and can last for hours after the device is turned off.
Complications
The rates of typical surgical complications, such as infection4,19,62 or hemorrhage,11,20 are comparable between MCS surgery and other neurosurgical procedures involving hardware implantation. There may be a relationship between severe, life-threatening hematomas and the use of subdural electrodes.88
An important complication particular to MCS surgery is the precipitation of seizures, especially when testing the stimulator at relatively high voltages. In one study, the mean seizure threshold was 9 V, with a lower limit of 6 V.72 Therefore, using voltages under that range may be safe. In addition, patients may be maintained on anticonvulsive medications while the voltage parameter space is explored, to further reduce the risk of seizure.70 This approach is particularly helpful for patients in whom the range of maximal efficacy is close to the seizure threshold.
Other less common complications of MCS for neuropathic facial pain include pain due to stimulation of the dura11 and dysesthesias.20 Finally, there is the suggestion that MCS may interfere with simple cognitive processes in elderly patients, especially with pre-existing cognitive difficulty.89
Brown J.A., Barbaro N.M. Motor cortex stimulation for central and neuropathic pain: current status. Pain. 2003;104(3):431-435.
Brown J.A., Pilitsis J.G. Motor cortex stimulation for central and neuropathic facial pain: a prospective study of 10 patients and observations of enhanced sensory and motor function during stimulation. Neurosurgery. 2005;56(2):290-297. discussion 290-297
Ebel H., Rust D., Tronnier V., et al. Chronic precentral stimulation in trigeminal neuropathic pain. Acta Neurochir (Wien). 1996;138(11):1300-1306.
García-Larrea L., Peyron R., Mertens P., et al. Electrical stimulation of motor cortex for pain control: a combined PET-scan and electrophysiological study. Pain. 1999;83(2):259-273.
Gharabaghi A., Hellwig D., Klaus S., et al. Volumetric image guidance for motor cortex stimulation: integration of three-dimensional cortical anatomy and functional imaging. Neurosurgery. 2005;57(suppl 1):114-120.
Gharabaghi A., Hellwig D., Rosahl S.K., et al. Volumetric image guidance for motor cortex stimulation: integration of three-dimensional cortical anatomy and functional imaging. Neurosurgery. 2005;57(suppl 1):114-120. discussion 114-120
Henderson J.M., Boongird A., Rosenow J.M., et al. Recovery of pain control by intensive reprogramming after loss of benefit from motor cortex stimulation for neuropathic pain. Stereotact Funct Neurosurg. 2004;82(5-6):207-213.
Henderson J.M., Lad S.P. Motor cortex stimulation and neuropathic facial pain. Neurosurgical Focus. 2006;21(6):E6.
Herregodts P., Stadnik T., De Ridder F., et al. Cortical stimulation for central neuropathic pain: 3-D surface MRI for easy determination of the motor cortex. Acta Neurochir Suppl (Wien). 1995;64:132-135.
Kishima H., Saitoh Y., Osaki Y., et al. Motor cortex stimulation in patients with deafferentation pain: activation of the posterior insula and thalamus. J Neurosurg. 2007;107(1):43-48.
Koyama S., Katayama Y., Maejima S., et al. Thalamic neuronal hyperactivity following transection of the spinothalamic tract in the cat: involvement of N-methyl-D-aspartate receptor. Brain Res. 1993;612(1-2):345-350.
Lefaucheur J.-P., Drouot X., Cunin P., et al. Motor cortex stimulation for the treatment of refractory peripheral neuropathic pain. Brain. 2009;132(Pt 6):1463-1471.
Lefaucheur J.P., Drouot X., Menard-Lefaucheur I., et al. Neurogenic pain relief by repetitive transcranial magnetic cortical stimulation depends on the origin and the site of pain. J Neurol Neurosurg Psychiatry. 2004;75(4):612-616.
Meyerson B.A., Lindblom U., Linderoth B., et al. Motor cortex stimulation as treatment of trigeminal neuropathic pain. Acta Neurochir Suppl (Wien). 1993;58:150-153.
Mogilner A.Y., Rezai A.R. Epidural motor cortex stimulation with functional imaging guidance. Neurosurg Focus. 2001;11(3):E4.
Nguyen J.P., Lefaucher J.P., Le Guerinel C., et al. Motor cortex stimulation in the treatment of central and neuropathic pain. Arch Med Res. 2000;31(3):263-265.
Nuti C., Peyron R., Garcia-Larrea L., et al. Motor cortex stimulation for refractory neuropathic pain: four year outcome and predictors of efficacy. Pain. 2005;118(1-2):43-52.
Peyron R., Faillenot I., Mertens P., et al. Motor cortex stimulation in neuropathic pain. Correlations between analgesic effect and hemodynamic changes in the brain. A PET study. Neuroimage. 2007;34(1):310-321.
Pirotte B., Voordecker P., Neugroschl C., et al. Combination of functional magnetic resonance imaging-guided neuronavigation and intraoperative cortical brain mapping improves targeting of motor cortex stimulation in neuropathic pain. Neurosurgery. 2005;56(suppl 2):344-359. discussion 344-359
Rainov N.G., Heidecke V. Motor cortex stimulation for neuropathic facial pain. Neurol Res. 2003;25(2):157-161.
Rasche D., Ruppolt M., Stippich C., et al. Motor cortex stimulation for long-term relief of chronic neuropathic pain: a 10 year experience. Pain. 2006;121(1-2):43-52.
Saitoh Y., Kato A., Ninomiya H., et al. Primary motor cortex stimulation within the central sulcus for treating deafferentation pain. Acta Neurochir Suppl (Wien). 2003;87:149-152.
Saitoh Y., Osaki Y., Nishimura H., et al. Increased regional cerebral blood flow in the contralateral thalamus after successful motor cortex stimulation in a patient with poststroke pain. J Neurosurg. 2004;100(5):935-939.
Thomas L., Bledsoe J.M., Stead M., et al. Motor cortex and deep brain stimulation for the treatment of intractable neuropathic face pain. Curr Neurol Neurosci Rep. 2009;9(2):120-126.
Velasco F., Argüelles C., Carrillo-Ruiz J.D., et al. Efficacy of motor cortex stimulation in the treatment of neuropathic pain: a randomized double-blind trial. J Neurosurg. 2008;108(4):698-706.
1. Tsubokawa T., Katayama Y., Yamamoto T., et al. Chronic motor cortex stimulation for the treatment of central pain. Acta Neurochir Suppl (Wien). 1991;52:137-139.
2. Tsubokawa T., Katayama Y., Yamamoto T., et al. Treatment of thalamic pain by chronic motor cortex stimulation. Pacing Clin Electrophysiol. 1991;14(1):131-134.
3. Tsubokawa T., Katayama Y., Yamamoto T., et al. Chronic motor cortex stimulation in patients with thalamic pain. J Neurosurg. 1993;78(3):393-401.
4. Brown J.A., Pilitsis J.G. Motor cortex stimulation for central and neuropathic facial pain: a prospective study of 10 patients and observations of enhanced sensory and motor function during stimulation. Neurosurgery. 2005;56(2):290-297. discussion 290-297
5. Drouot X., Nguyen J.-P., Peschanski M., et al. The antalgic efficacy of chronic motor cortex stimulation is related to sensory changes in the painful zone. Brain. 2002;125(Pt 7):1660-1664.
6. Ebel H., Rust D., Tronnier V., et al. Chronic precentral stimulation in trigeminal neuropathic pain. Acta Neurochir (Wien). 1996;138(11):1300-1306.
7. Gharabaghi A., Hellwig D., Klaus S., et al. Volumetric image guidance for motor cortex stimulation: integration of three-dimensional cortical anatomy and functional imaging. Neurosurgery. 2005;57(suppl 1):114-120.
8. Herregodts P., Stadnik T., De Ridder F., et al. Cortical stimulation for central neuropathic pain: 3-D surface MRI for easy determination of the motor cortex. Acta Neurochir Suppl (Wien). 1995;64:132-135.
9. Katayama Y., Tsubokawa T., Yamamoto T. Chronic motor cortex stimulation for central deafferentation pain: experience with bulbar pain secondary to Wallenberg syndrome. Stereotact Funct Neurosurg. 1994;62(1-4):295-299.
10. Lefaucheur J.-P., Drouot X., Cunin P., et al. Motor cortex stimulation for the treatment of refractory peripheral neuropathic pain. Brain. 2009;132(Pt 6):1463-1471.
11. Meyerson B.A., Lindblom U., Linderoth B., et al. Motor cortex stimulation as treatment of trigeminal neuropathic pain. Acta Neurochir Suppl (Wien). 1993;58:150-153.
12. Mogilner A.Y., Rezai A.R. Epidural motor cortex stimulation with functional imaging guidance. Neurosurg Focus. 2001;11(3):E4.
13. Nguyen J.P., Lefaucheur J.P., Decq P., et al. Chronic motor cortex stimulation in the treatment of central and neuropathic pain. Correlations between clinical, electrophysiological and anatomical data. Pain. 1999;82(3):245-251.
14. Nguyen J.P., Lefaucheur J.P., Le Guerinel C., et al. Treatment of central and neuropathic facial pain by chronic stimulation of the motor cortex: value of neuronavigation guidance systems for the localization of the motor cortex. Neurochirurgie. 2000;46(5):483-491.
15. Rainov N.G., Fels C., Heidecke V., et al. Epidural electrical stimulation of the motor cortex in patients with facial neuralgia. Clin Neurol Neurosurg. 1997;99(3):205-209.
16. Rainov N.G., Heidecke V. Motor cortex stimulation for neuropathic facial pain. Neurol Res. 2003;25(2):157-161.
17. Rasche D., Ruppolt M., Stippich C., et al. Motor cortex stimulation for long-term relief of chronic neuropathic pain: a 10 year experience. Pain. 2006;121(1-2):43-52.
18. Velasco F., Argüelles C., Carrillo-Ruiz J.D., et al. Efficacy of motor cortex stimulation in the treatment of neuropathic pain: a randomized double-blind trial. Journal of Neurosurgery. 2008;108(4):698-706.
19. Pirotte B., Voordecker P., Neugroschl C., et al. Combination of functional magnetic resonance imaging-guided neuronavigation and intraoperative cortical brain mapping improves targeting of motor cortex stimulation in neuropathic pain. Neurosurgery. 2005;56(suppl 2):344-359. discussion 344-359
20. Nguyen J.P., Keravel Y., Feve A., et al. Treatment of deafferentation pain by chronic stimulation of the motor cortex: report of a series of 20 cases. Acta Neurochir Suppl (Wien). 1997;68:54-60.
21. Penfield W., Jasper H. Epilepsy and the Functional Anatomy of the Human Brain. Boston, MA: Little, Brown and Co; 1954.
22. Penfield W., Welch K. The supplementary motor area of the cerebral cortex: a clinical and experimental study. AMA Arch Neurol Psychiatry. 1951;66(3):289-317.
23. Lende R.A., Kirsch W.M., Druckman R. Relief of facial pain after combined removal of precentral and postcentral cortex. J Neurosurg. 1971;34(4):537-543.
24. Coffey R.J. Deep brain stimulation for chronic pain: results of two multicenter trials and a structured review. Pain Med. 2001;2(3):183-192.
25. Burchiel K.J. Deep brain stimulation for chronic pain: the results of two multi-center trials and a structured review. Pain Med. 2001;2(3):177.
26. Bittar R.G., Kar-Purkayastha I., Owen S.L., et al. Deep brain stimulation for pain relief: a meta-analysis. J Clin Neurosci. 2005.
27. Nguyen J.P., Lefaucher J.P., Le Guerinel C., et al. Motor cortex stimulation in the treatment of central and neuropathic pain. Arch Med Res. 2000;31(3):263-265.
28. Brown J.A., Barbaro N.M. Motor cortex stimulation for central and neuropathic pain: current status. Pain. 2003;104(3):431-435.
29. García-Larrea L., Peyron R., Mertens P., et al. Electrical stimulation of motor cortex for pain control: a combined PET-scan and electrophysiological study. Pain. 1999;83(2):259-273.
30. Hanajima R., Ashby P., Lang A.E., et al. Effects of acute stimulation through contacts placed on the motor cortex for chronic stimulation. Clin Neurophysiol. 2002;113(5):635-641.
31. Peyron R., Garcia-Larrea L., Deiber M.P., et al. Electrical stimulation of precentral cortical area in the treatment of central pain: electrophysiological and PET study. Pain. 1995;62(3):275-286.
32. Lefaucheur J.P. The use of repetitive transcranial magnetic stimulation (rTMS) in chronic neuropathic pain. Neurophysiol Clin. 2006;36(3):117-124.
33. Veldhuijzen D.S., Greenspan J.D., Kim J.H., et al. Imaging central pain syndromes. Curr Pain Headache Rep. 2007;11(3):183-189.
34. Borsook D., Becerra L. Phenotyping central nervous system circuitry in chronic pain using functional MRI: considerations and potential implications in the clinic. Curr Pain Headache Rep. 2007;11(3):201-207.
35. Gorecki J., Hirayama T., Dostrovsky J.O., et al. Thalamic stimulation and recording in patients with deafferentation and central pain. Stereotact Funct Neurosurg. 1989;52(2-4):219-226.
36. Koyama S., Katayama Y., Maejima S., et al. Thalamic neuronal hyperactivity following transection of the spinothalamic tract in the cat: involvement of N-methyl-D-aspartate receptor. Brain Res. 1993;612(1-2):345-350.
37. Lenz F.A., Kwan H.C., Dostrovsky J.O., et al. Characteristics of the bursting pattern of action potentials that occurs in the thalamus of patients with central pain. Brain Res. 1989;496(1-2):357-360.
38. Tasker R.R., Gorecki J., Lenz F.A., et al. Thalamic microelectrode recording and microstimulation in central and deafferentation pain. Appl Neurophysiol. 1987;50(1-6):414-417.
39. Tsubokawa T., Katayama Y., Yamamoto T., et al. Deafferentation pain and stimulation of the thalamic sensory relay nucleus: clinical and experimental study. Appl Neurophysiol. 1985;48(1-6):166-171.
40. Namba S., Shimizu Y., Wani T., et al. An experimental model of deafferented pain in the cat. Appl Neurophysiol. 1985;48(1-6):201-211.
41. Rinaldi P.C., Young R.F., Albe-Fessard D., et al. Spontaneous neuronal hyperactivity in the medial and intralaminar thalamic nuclei of patients with deafferentation pain. J Neurosurg. 1991;74(3):415-421.
42. Namba S., Nishimoto A. Stimulation of internal capsule, thalamic sensory nucleus (VPM) and cerebral cortex inhibited deafferentation hyperactivity provoked after gasserian ganglionectomy in cat. Acta Neurochir Suppl (Wien). 1988;42:243-247.
43. Lindblom U.F., Ottosson J.O. Influence of pyramidal stimulation upon the relay of coarse cutaneous afferents in the dorsal horn. Acta Physiol Scand. 1957;38(3-4):309-318.
44. Coulter J.D., Maunz R.A., Willis W.D. Effects of stimulation of sensorimotor cortex on primate spinothalamic neurons. Brain Res. 1974;65(2):351-356.
45. Hardy S.G. Analgesia elicited by prefrontal stimulation. Brain Res. 1985;339(2):281-284.
46. Hardy S.G., Haigler H.J. Prefrontal influences upon the midbrain: a possible route for pain modulation. Brain Res. 1985;339(2):285-293.
47. Senapati A.K., Huntington P.J., Peng Y.B. Spinal dorsal horn neuron response to mechanical stimuli is decreased by electrical stimulation of the primary motor cortex. Brain Res. 2005;1036(1-2):173-179.
48. Arle J.E., Shils J.L. Motor cortex stimulation for pain and movement disorders. Neurotherapeutics. 2008;5(1):37-49.
49. Di Lazzaro V., Oliviero A., Pilato F., et al. The physiological basis of transcranial motor cortex stimulation in conscious humans. Clin Neurophysiol. 2004;115(2):255-266.
50. Holsheimer J., Nguyen J.-P., Lefaucheur J.-P., et al. Cathodal, anodal or bifocal stimulation of the motor cortex in the management of chronic pain? Acta Neurochir Suppl (Wien). 2007;97(Pt 2):57-66.
51. Lefaucheur J.P., Drouot X., Ménard-Lefaucheur I., et al. Motor cortex rTMS restores defective intracortical inhibition in chronic neuropathic pain. Neurology. 2006;67(9):1568-1574.
52. Rouiller E.M., Tanné J., Moret V., et al. Dual morphology and topography of the corticothalamic terminals originating from the primary, supplementary motor, and dorsal premotor cortical areas in macaque monkeys. J Comp Neurol. 1998;396(2):169-185.
53. Lang N., Siebner H.R., Ward N.S., et al. How does transcranial DC stimulation of the primary motor cortex alter regional neuronal activity in the human brain? Eur J Neurosci. 2005;22(2):495-504.
54. Peyron R., Faillenot I., Mertens P., et al. Motor cortex stimulation in neuropathic pain. Correlations between analgesic effect and hemodynamic changes in the brain. A PET study. Neuroimage. 2007;34(1):310-321.
55. Hsieh J., Meyerson B., Ingvar M. PET study on central processing of pain in trigeminal neuropathy. Eur J Pain. 1999;3(1):51-65.
56. Kishima H., Saitoh Y., Osaki Y., et al. Motor cortex stimulation in patients with deafferentation pain: activation of the posterior insula and thalamus. J Neurosurg. 2007;107(1):43-48.
57. Saitoh Y., Osaki Y., Nishimura H., et al. Increased regional cerebral blood flow in the contralateral thalamus after successful motor cortex stimulation in a patient with poststroke pain. J Neurosurg. 2004;100(5):935-939.
58. Canavero S., Bonicalzi V. Therapeutic extradural cortical stimulation for central and neuropathic pain: a review. Clin J Pain. 2002;18(1):48-55.
59. Henderson J.M., Lad S.P. Motor cortex stimulation and neuropathic facial pain. Neurosurgical Focus. 2006;21(6):E6.
60. Mertens P., Nuti C., Sindou M., et al. Precentral cortex stimulation for the treatment of central neuropathic pain: results of a prospective study in a 20-patient series. Stereotact Funct Neurosurg. 1999;73(1-4):122-125.
61. Devinsky O., Morrell M.J., Vogt B.A. Contributions of anterior cingulate cortex to behaviour. Brain. 1995;118(1):279-306.
62. Nuti C., Peyron R., Garcia-Larrea L., et al. Motor cortex stimulation for refractory neuropathic pain: four year outcome and predictors of efficacy. Pain. 2005;118(1-2):43-52.
63. Maarrawi J., Peyron R., Mertens P., et al. Motor cortex stimulation for pain control induces changes in the endogenous opioid system. Neurology. 2007;69(9):827-834.
64. Burchiel K.J. A new classification for facial pain. Neurosurgery. 2003;53(5):1164-1166.
65. Broggi G., Ferroli P., Franzini A. Treatment strategy for trigeminal neuralgia: a thirty years experience. Neurol Sci. 2008;29(Suppl 1):579-582.
66. Cheshire W.P. Trigeminal neuralgia: for one nerve a multitude of treatments. Expert Rev Neurother. 2007;7(11):1565-1579.
67. Obermann M., Katsarava Z. Update on trigeminal neuralgia. Expert Rev Neurother. 2009;9(3):323-329.
68. Cruccu G., Aziz T.Z., Garcia-Larrea L., et al. EFNS guidelines on neurostimulation therapy for neuropathic pain. Eur J Neurol. 2007;14(9):952-970.
69. Fontaine D., Hamani C., Lozano A. Efficacy and safety of motor cortex stimulation for chronic neuropathic pain: critical review of the literature. J Neurosurg. 2009;110(2):251-256.
70. Thomas L., Bledsoe J.M., Stead M., et al. Motor cortex and deep brain stimulation for the treatment of intractable neuropathic face pain. Curr Neurol Neurosci Rep. 2009;9(2):120-126.
71. Cioni B., Meglio M. Motor cortex stimulation for chronic non-malignant pain: current state and future prospects. Acta Neurochir Suppl. 2007;97(Pt 2):45-49.
72. Henderson J.M., Boongird A., Rosenow J.M., et al. Recovery of pain control by intensive reprogramming after loss of benefit from motor cortex stimulation for neuropathic pain. Stereotact Funct Neurosurg. 2004;82(5-6):207-213.
73. Yamamoto T., Katayama Y., Hirayama T., et al. Pharmacological classification of central post-stroke pain: comparison with the results of chronic motor cortex stimulation therapy. Pain. 1997;72(1-2):5-12.
74. Katayama Y., Fukaya C., Yamamoto T. Poststroke pain control by chronic motor cortex stimulation: neurological characteristics predicting a favorable response. Journal of Neurosurgery. 1998;89(4):585-591.
75. Krings T., Naujokat C., von Keyserlingk D.G. Representation of cortical motor function as revealed by stereotactic transcranial magnetic stimulation. Electroencephalogr Clin Neurophysiol. 1998;109(2):85-93.
76. Migita K., Uozumi T., Arita K., et al. Transcranial magnetic coil stimulation of motor cortex in patients with central pain. Neurosurgery. 1995;36(5):1037-1039. discussion 1039-1040
77. Biega T.J., Lonser R.R., Butman J.A. Differential cortical thickness across the central sulcus: a method for identifying the central sulcus in the presence of mass effect and vasogenic edema. AJNR Am J Neuroradiol. 2006;27(7):1450-1453.
78. Gonzales-Portillo G. Localization of the central sulcus. Surg Neurol. 1996;46(1):97-99.
79. Naidich T.P., Valavanis A.G., Kubik S. Anatomic relationships along the low-middle convexity. Part I—Normal specimens and magnetic resonance imaging. Neurosurgery. 1995;36(3):517-532.
80. Yousry T.A., Schmid U.D., Alkadhi H., et al. Localization of the motor hand area to a knob on the precentral gyrus. A new landmark. Brain. 1997;120(Pt 1):141-157.
81. Khedr E.M., Kotb H., Kamel N.F., et al. Longlasting antalgic effects of daily sessions of repetitive transcranial magnetic stimulation in central and peripheral neuropathic pain. J Neurol Neurosurg Psychiatry. 2005;76(6):833-838.
82. Lefaucheur J.P., Drouot X., Menard-Lefaucheur I., et al. Neurogenic pain relief by repetitive transcranial magnetic cortical stimulation depends on the origin and the site of pain. J Neurol Neurosurg Psychiatry. 2004;75(4):612-616.
83. Saitoh Y., Hirano S., Kato A., et al. Motor cortex stimulation for deafferentation pain. Neurosurg Focus. 2001;11(3):E1.
84. Gharabaghi A., Hellwig D., Rosahl S.K., et al. Volumetric image guidance for motor cortex stimulation: integration of three-dimensional cortical anatomy and functional imaging. Neurosurgery. 2005;57(suppl 1):114-120. discussion 114-120
85. Simon M.V., editor. Intraoperative Neurophysiology: a Comprehensive Guide to Monitoring and Mapping. New York: Demos Medical Publishing, 2009.
86. Romstock J., Fahlbusch R., Ganslandt O., et al. Localisation of the sensorimotor cortex during surgery for brain tumours: feasibility and waveform patterns of somatosensory evoked potentials. J Neurol Neurosurg Psychiatry. 2002;72(2):221-229.
87. Anderson W.S., Kiyofuji S., Conway J.E., et al. Dysphagia and neuropathic facial pain treated with motor cortex stimulation: case report. Neurosurgery. 2009;65(3):E626. discussion E626
88. Saitoh Y., Kato A., Ninomiya H., et al. Primary motor cortex stimulation within the central sulcus for treating deafferentation pain. Acta Neurochir Suppl (Wien). 2003;87:149-152.
89. Montes C., Mertens P., Convers P., et al. Cognitive effects of precentral cortical stimulation for pain control: an ERP study. Neurophysiol Clin. 2002;32(5):313-325.