Chapter 48 Mosquitoes and Mosquito-Borne Diseases
Mosquitoes are in the biologic phylum Arthropoda, class Insecta, order Diptera, family Culicidae. There are approximately 35 genera, which include Anopheles, Culex, Psorophora, Ochlerotatus, Aedes, Sabethes, Wyeomyia, Culiseta, and Haemagogus. Worldwide there are more than 3000 species and subspecies, and in the United States alone, there are an estimated 200 different species of mosquitoes that vary in habitat and behavior.179 In addition to transmitting the parasitic disease of malaria to 300 to 500 million people per year and thereby killing up to 2.7 million people per year,13 mosquitoes also serve as vectors for arboviruses that cause significant morbidity and mortality to humans worldwide. In addition, mosquitoes are responsible for transmission of the larval nematode that leads to lymphatic filariasis.
Mosquitoes
Mosquito Anatomy
Mosquito anatomy (Figure 48-1) includes a slender body with two wings (rather than the four possessed by flies) and six long, delicate legs. The wings are covered with scales. The body is divided into three parts: head, thorax, and abdomen. The head has two large compound eyes with many lenses angled in different directions, and two antennae. The mouth, or proboscis, looks like a funnel extending downward, and is used to pierce skin and suck blood (for females) or sip nectar (for males). The thin and short neck connects the head to the thorax, which is triangular and holds the wings in place. The thorax also has two pairs of spiracles, which are tubes through which the mosquito can breathe. The shape of the abdomen can be pointed or rounded, depending on the species, and it has eight pairs of spiracles. The legs are divided into the coxa, femur, tibia, and tarsus.
The spiracles form the basis of the mosquito’s tracheal system, which is finely branched so that the cells of the body are directly oxygenated. Mosquitoes have a dorsal blood vessel that extends directly from the eighth abdominal segment into the head. The heart is the portion of the blood vessel located within the thorax and although it is not innervated, it pulses automatically at a rate of 85 beats/min.161
Mosquito Life Cycle
The life cycle of most species of mosquitoes is defined by four stages: egg, larva, pupa, and adult. The time a mosquito spends in each stage depends on the species and temperature. Culex tarsalis, for example, has a 14-day life cycle at 20° C (68° F), and 10-day life cycle at 25° C (77° F). The life cycle varies from 4 days to 1 month, but averages around 2 weeks, which means that several generations can arise within a single year.179 Most mosquitoes that do not have habitats in the tropics lie dormant as eggs, although some, such as the genus Culex, overwinter as larvae or adults.
The female mosquito usually mates once in her lifetime and stores sperm in her body. She fertilizes her eggs when she lays them, which is generally on the surface of the water—often in the form of egg rafts, shown in Figure 48-2—or sometimes in groups in empty containers or jars, shown in Figure 48-3. Eggs can survive winter and hatch in the spring. They hatch into larvae (also known as wrigglers), about 1 to 2 cm (0.4 to 0.8 inch) long, that feed on microscopic animals, plant life, and, in some cases, other mosquito larvae. Except for the genus Anopheles, the larvae must come to the surface to employ their air tubes, or siphons, which supplement their gills (Figure 48-4). After molting several times, they develop into pupae (Figure 48-5), floating on the surface of the water and breathing through an air tube, now called a trumpet. While encased in the hard pupal case, they transform into adult mosquitoes. Enough pressure is created to burst the case, and when their wings harden, they can fly and live on land.161
Mechanism of Mosquito Bites
Mosquitoes technically do not sting, because there is no stinger; they simply pierce the skin and suck blood. Only the females of most species have sucking mouth parts that can pierce skin and thus transmit disease (Figure 48-6). The blood protein is required because the normal mosquito diet of nectar and fruit juices does not contain enough protein for egg development. (One genus, Toxorhynchites, does not suck blood, and its larvae prey on other mosquito larvae). Mosquitoes generally identify victims by scent, as well as by the carbon dioxide of exhaled breath and some chemicals found in sweat. Mosquitoes have been shown to have odorant-binding proteins on their antennae that bind human-specific odorants, stimulating their olfactory neurons and allowing them to locate their human prey.154 Other risk factors for getting bitten include male gender, heavier weight, and type O blood.61
When mosquitoes pierce human skin, all six legs are usually positioned on the skin surface, along with the labella (part of the proboscis). The female proboscis is made up of six shafts, four of which cut and pierce skin. One serves as a conduit for blood into the mosquito, and another transports mosquito saliva into the skin. The mosquito lays the labella on the skin, which allows the maxillae of the fascicle (a bundle of feeding stylets, contained within the proboscis) to saw into the skin in an oscillatory cutting fashion. Blood is drawn up into the fascicle, which collects into the labrum held between the mandibles of the mosquito. The female mosquito usually requires about 50 seconds to insert the fascicle into the skin, and approximately 2 minutes to finish feeding. She can withdraw her fascicle in 5 seconds.60,69
Mosquito saliva contains a wide array of antihemostatic molecules that allow feeding upon blood. These molecules include vasodilators, such as amines and prostaglandins; platelet aggregation inhibitors, such as nitric oxide; prostaglandins; apyrase; molecules that sequester adenosine diphosphate (ADP-sequestering molecules), and peptides and proteins specifically targeted to integrin receptors; and anticoagulants, such as thrombin and factor Xa inhibitors. The saliva and its accompanying proteins (specifically, the 33-kilodalton F-1 protein found in mosquito saliva) that remain in human skin after feeding serve as antigens that evoke an immune response.43
Pathophysiology and Clinical Manifestations of Mosquito Bites
Individuals vary in their responses to mosquito bites. Almost all experience local irritation caused by a type I immunoglobulin E (IgE)-mediated response of a soft, pale, pruritic wheal or plaque (Figure 48-7). The subsequent effects reveal which immune response a person has to the bite. Type I IgE responses can also create immediate hive-like skin lesions (rare), whereas type IV cell-mediated immunity causes delayed pruritic papules or vesicles within 48 hours of the bite. Other type IV reactions caused by mosquito bites include blisters, bullae, and erythema multiforme or purpura, but these are rare.
One common type IV hypersensitivity response is papular urticaria, which describes an eruption of pruritic papules measuring 3 to 10 mm (0.1 to 0.4 inch) in diameter that may be surrounded by vesicles grouped in irregular clusters (Figure 48-8). Because repeated exposures may lead to desensitization in adults, these lesions are more common in children. They begin as an erythematous wheal, lasting from 2 to 10 days, and may lead to temporary hyperpigmentation. Children who suffer this hypersensitivity may develop a firm, light-brown papule, whereas most adults have only a transient wheal without formation of a persistent papule. The dermatopathology of the epidermis in papular urticaria shows spongiosis with exocytosis and vesicle formation. The upper dermal layer reveals a localized perivascular infiltrate with lymphocytes, histiocytes, eosinophils, and mast cells, and a spattering of eosinophils and mast cells in the mid-dermis.168
The pathophysiology behind the phenomenon of itching is complex. There are abundant theories on the neurophysiologic basis of itch. The most traditional is that the local reaction induces production of histamine, which stimulates C nerve fibers that travel to the brain and induce the sensation of itching.195 Histamine, however, is only one of many pruritogenic substances that stimulate a subset of specialized skin C fibers. Other mediators of itch include proteases, opioids, lipid peroxidation metabolites (such as leukotrienes and prostaglandins), neuropeptides (e.g., substance P), cytokines, and growth factors (e.g., nerve growth factor). Pruritus is now seen as an interdisciplinary issue that reaches into the fields of neurophysiology, neuroimmunology, neuropharmacology, protease research, internal medicine, and dermatology.
Diseases
The effects of mosquitoes on human life are far reaching. Every year, they are responsible for transmitting a wide spectrum of diseases, such as malaria, dengue fever, yellow fever, and encephalitis to millions of people. This chapter discusses the main diseases transmitted by mosquitoes, other than malaria (see Chapter 49). Table 48-1 summarizes the viruses discussed in this chapter.
Dengue
Dengue was first identified as being caused by a virus in the 1940s, although its existence was probably present much earlier, as reports of dengue fever epidemics occurred as early as 1779. Like West Nile virus (WNV), dengue viruses belong to the family Flaviviridae, genus Flavivirus, and have four distinct but closely related serotypes (DEN-1, DEN-2, DEN-3, and DEN-4), all of which cause infection. Dengue has become a major public health issue over recent decades, as the incidences of cases and dengue hemorrhagic fever (DHF) epidemics have dramatically increased. Currently, it is estimated that 50 to 100 million people in more than 100 countries are infected yearly with dengue viruses.76,189
Epidemiology and Transmission
Several species of Aedes can transmit dengue, but the most important is Aedes aegypti, which is found in temperate zones from latitude 45 degrees north to 35 degrees south. Certain factors make Aedes mosquitoes a particularly effective vector. Specifically, the mosquito itself is quite susceptible to dengue virus; they feed preferentially on human blood; they inhabit highly populated areas and breed inside or close to houses; and their bite is often unnoticed by humans. Moreover, because they feed many times in a breeding cycle, numerous people living in one household can be infected by the same mosquito. Aedes albopictus has a larger geographic distribution than does A. aegypti and can also transmit the virus, but it is less often the culprit responsible for dengue transmission because these mosquitoes breed farther from households and bite humans less frequently.67,76
Dengue virus transmission is classified as epidemic dengue when a single virus strain is introduced into a region with a large number of hosts and mosquitoes, or as hyperendemic when there is uninterrupted circulation of numerous dengue serotypes in one area. Epidemic dengue is the usual transmission pattern for many smaller islands in South America, Africa, and Asia, where several years pass between epidemics and then the disease reemerges. For travelers, this means that the risk for acquiring the infection is high during epidemics, but it is very low between those periods. Generally the frequency of DHF during epidemics seems to be lower than that in areas where the transmission pattern is hyperendemic. In hyperendemic areas, competent mosquito vectors are present throughout the year, and the population of uninfected individuals is sufficiently large to sustain the disease. This latter pattern of transmission accounts for the preponderance of global dengue virus infections.74
As shown in Figure 48-9, dengue virus has been found in Southeast Asia and the western Pacific islands (with hyperendemic transmission of all four serotypes present in Thailand, Vietnam, Indonesia, Malaysia, and the Philippines). Hyperendemic dengue fever (DF) is also found in India, Pakistan, and Sri Lanka. A. aegypti mosquitoes are also distributed across much of sub-Saharan Africa and the Middle East. In the Americas, A. aegypti exists in the southeastern United States, Mexico, Central America, South America, and the Caribbean.76
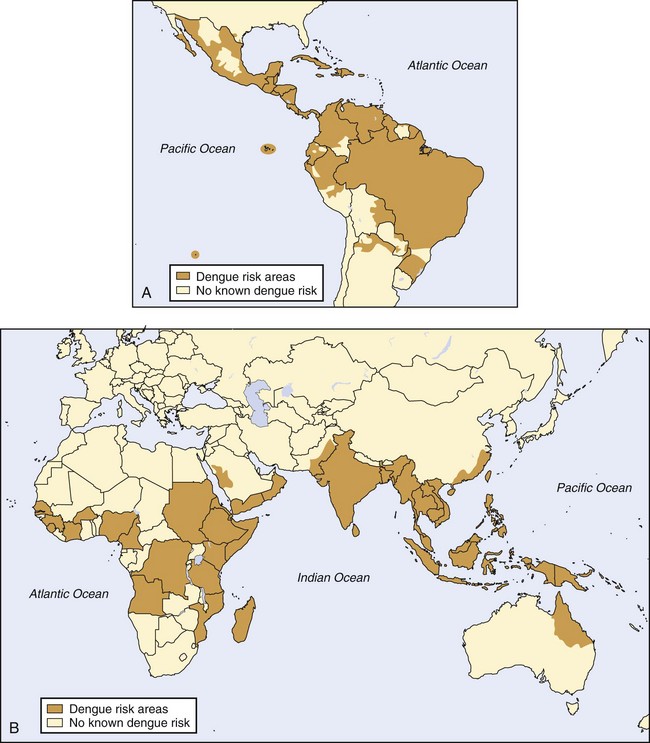
FIGURE 48-9 A, Distribution of dengue, western hemisphere. B, Distribution of dengue, eastern hemisphere.
(From http://wwwnc.cdc.gov/travel/yellowbook/2010/chapter-5/dengue-fever-denguehemorrhagic-fever.aspx.)
Reports of DF and DHF are increasing. In fact, before 1970, only nine countries had suffered DHF epidemics; by 1995, this number had expanded more than fourfold. In the past, DF was considered a relatively benign and nonfatal disease. However, since World War II, during which time Aedes mosquitoes were transported around the world in cargo, there has been expansion of the disease not only in frequency and geographic distribution but also in morbidity and mortality. The 1980s and 1990s saw a rise in DHF (specifically, serotype DEN-3) in southern Asia, and in the 1980s, there was a rise in epidemic DF/DHF (specifically serotype DEN-2) in Taiwan and the People’s Republic of China. Africa has also seen large increases in DF incidence since 1980.72 Dengue has also been emerging much more rapidly in the Americas, with the first major outbreak in Cuba in 1981 and continuing outbreaks in other Central and South American countries since then.145 The World Health Organization (WHO) reports that in 2007 alone, nearly 900,000 cases of dengue were reported in the Americas, with 26,000 cases of DHF. Countries such as Venezuela reported devastating epidemics in the same year, with more than 80,000 cases of DF and more than 6000 cases of DHF.189
Demographic changes, such as population growth and increased human density, contribute to transmission and also to emergence of DHF. Moreover, unplanned urbanization resulting in substandard housing situations and poor water and sanitation systems leads to the same outcome. The situation seems to be potentiated by deterioration of mosquito control programs and of health systems in general in dengue-endemic countries, where the disease cannot be well controlled.110 Climate change alters the interaction between infective mosquitoes and humans. Increased rainfall during certain seasons in tropical areas causes an increased density of mosquitoes. Not only do warmer temperatures decrease the incubation period of the virus inside the mosquito so that the virus can be transmitted earlier, but they may also allow greater mosquito population growth and raise the number of susceptible humans, who perpetuate the disease.79
Clinical Presentation
The clinical presentation of dengue virus can range from asymptomatic infection to self-limited mild febrile illness to life-threatening shock syndrome. Risk factors influence disease severity and development of DHF or dengue shock syndrome (DSS), as opposed to simply dengue fever. These factors include serotype, prior exposure, age, malnutrition, and genetic factors such as human leukocyte antigen (HLA) type. All four serotypes can cause DHF, but DEN-2 appears to be most virulent. The four serotypes do not provide cross-protective immunity, so it is possible for one individual to sustain four separate dengue infections over a lifetime. Although previous infection provides homologous immunity to one serotype, individuals who experience secondary infection by another serotype are at higher risk for more severe forms of disease. This theory supports the observation that infants less than 1 year of age with passively acquired maternal antibodies have a higher rate of DHF/DSS. This phenomenon, known as immune enhancement or antibody-dependent enhancement, is thought to occur because the Fc receptors of monocytes by dengue viruses complexed to IgG antibodies are more accessible to infectious immune complexes and result in a higher number of cells being infected with this different serotype.75,114,123 In terms of age distribution, DHF in the past was seen as a disease of children (especially in Asia, where the disease was previously more predominant). This distribution, however, is slightly different in the Americas, and epidemics have shown increasing numbers of adult patients. Moreover, some epidemiologic studies have demonstrated that most children less than 15 years infected with dengue virus tend to be asymptomatic or have a mild fever. Malnourished children, compared with well-nourished children, seem less inclined to develop DHF/DSS than other diseases, perhaps secondary to immunosuppression. Whites have a higher frequency of developing severe disease than do blacks.114
The incubation period for the virus in the mosquito is 8 to 12 days before it is transmissible, and in humans there is a 4- to 6-day incubation period from bite to clinical infection. Pathogenesis of the virus is hypothesized to be a result of endothelial cell dysfunction, activation of cytokines and complement, and suppression of the reticuloendothelial system.76 At autopsy, the virus is commonly found in the liver, spleen, thymus, lymph nodes, and lung cells.187
WHO currently classifies symptomatic dengue infections into three categories for surveillance purposes. These include undifferentiated fever, classic dengue fever, and DHF. Although this classification system remains controversial,51 the clinical characteristics of DF, DHF, and DSS are discussed here.
The majority of children infected with dengue viruses are asymptomatic or present with mild self-limited fever. Typically, older children and adults with DF present with high fever (greater than 39° C [102.2° F]), myalgias, headache, arthralgias, and rash.187 Patients may also describe retro-orbital pain, and suffer gastrointestinal (nausea, vomiting, and diarrhea) and respiratory (cough, sore throat, and nasal congestion) symptoms.49 A particular characteristic of dengue infection is central nervous system (CNS) involvement in both DF and DHF. Patients present with symptoms that range from irritability and depression to encephalitis with seizures and death, with the more severe symptoms noted in DHF. Despite these complications, the prognosis for patients with DF is generally good, with an acute phase of 1 week and up to 2 weeks of convalescence with general malaise and anorexia. Case fatality rates for dengue fever are less than 1%.33
The main characteristic that differentiates DHF from DF is development of increased vascular permeability resulting in plasma leakage. After 2 to 7 days of high fever, patients with DHF have bleeding (ranging from petechiae, ecchymoses, epistaxis, and mucosal bleeding, to more severe gastrointestinal bleeding and hematuria), thrombocytopenia (<100,000/mm3), and, paradoxically, hemoconcentration and hepatomegaly in some settings.114 Symptoms include severe abdominal pain, nausea and vomiting, and restlessness or lethargy. Plasma leakage may cause pleural effusion, ascites, and hypoproteinemia. This may lead to circulatory failure, resulting in DSS with hypotension, cool extremities, and altered mental status. Encephalopathy is possible in DHF and can result from cerebral anoxia, edema, or intracranial hemorrhage.165 It is still currently unknown whether dengue viruses infiltrate the CNS directly or if neurologic manifestations are nonspecific complications. Case fatality rates for DHF are approximately 5%38; in DSS they can be greater than 40%.33
Diagnosis
Dengue is typically diagnosed clinically, because laboratory confirmation is typically only available in specialized reference laboratories in the developed world. Clinical characteristics are as discussed above; however, it may remain difficult to differentiate dengue from other febrile illnesses. One recent systematic review evaluating 15 studies conducted between 1990 and 2007 attempted to identify clinical criteria to help differentiate such disease processes. In this review, thrombocytopenia, leukopenia, neutropenia, transaminitis, and petechiae were all associated with dengue across multiple studies.147 Another study of 1200 patients from Vietnam and Singapore with acute febrile illness demonstrated a decision algorithm using clinical and hematologic parameters (platelet count, white blood cell count, neutrophil and lymphocyte counts, hematocrit, and body temperature), which accurately identified 84.7% of the dengue cases in their study population. This algorithm had a 71.2% sensitivity and 90.1% specificity for predicting the diagnosis of dengue but has not been externally validated.171
Such studies use both clinical and laboratory findings to identify patients with DF. The tourniquet test (Figure 48-10) for capillary fragility, which describes the appearance of 20 or more petechiae over a square-inch patch on the forearm after deflation of the blood pressure cuff (held for 5 minutes between systolic and diastolic pressures), may also be positive. Although WHO typically uses a positive tourniquet test to help distinguish DHF from DF, the test has been shown to be positive in both disease states.184 Patients may have neutropenia with subsequent lymphocytosis marked by atypical lymphocytes, hyponatremia, hypoproteinemia, and mild elevation of liver enzymes. Other findings include albuminuria and occult blood in the stool. In patients with DHF, either thrombocytopenia (defined by WHO as less than 100,000/mm3) or hemoconcentration (elevation of hematocrit 20% or greater than the average for age, gender, and population) is used by WHO to reach a provisional diagnosis of DHF if certain clinical observations (e.g., acute onset of high fever, hemorrhagic manifestations, hepatomegaly, shock) are made. In cases with extensive liver involvement, vitamin K–dependent prothrombin factors, such as II, VII, IX, and X, may be reduced, and assays of coagulation factors may reveal reduced fibrinogen, prothrombin, factors VIII and XII, or antithrombin III. About one-third to one-half of patients with DHF have increased partial thromboplastin and prothrombin times. Chest radiographs may show a unilateral (usually right-sided) pleural effusion in more severe disease.187
Definitive diagnosis of dengue can be made by isolating the virus or detecting specific antibodies. Immunofluorescence assay of serum or cerebrospinal fluid (CSF) with serotype-specific monoclonal antibodies (IgM enzyme-linked immunosorbent assay [ELISA]) is the test of choice to confirm diagnosis. Although the IgM immunoassay has a sensitivity of 90% to 97%,76 samples collected within the first 4 to 5 days of illness may lead to false negative results.184 Serologic diagnosis of DF then commonly depends on demonstration of a fourfold or greater increase in IgM or IgG antibody titer between acute-phase and convalescent-phase serum samples by hemagglutination inhibition or enzyme immunoassay (and less commonly complement fixation and neutralization test). Other methods for laboratory detection include detection of DF genomic sequences from autopsy tissue, serum, or CSF with polymerase chain reaction (PCR).187 Several protocols have been written for PCR detection of DF, because this technology can aid in detection of the virus within 1 to 2 days.46,47,52,95 However, this method is not widely available, and thus its use is limited to detecting dengue viruses in pathologic tissue, cell cultures, and mosquito specimens.76 Unfortunately, attempts to develop rapid bedside tests using immunochromatographic or immunoblot technologies remain relatively unsuccessful.11 Virus identification can also be confirmed by inoculation of serum into mosquitoes or certain mosquito cell lines (most commonly A. albopictus [C6-36] and Aedes pseudoscutellaris [AP61], but with better sensitivity when mosquitoes in the genus Toxorhynchites are used), but these methods are not commonly available.
The recommendation of the Centers for Disease Control and Prevention (CDC) is that, for testing and reporting, both acute-phase and convalescent-phase (6 days after the onset of symptoms) serum samples be sent to the state health department, which will forward them to the CDC for testing. The acute-phase specimen should be stored on dry ice (or unfrozen at 4° C [39.2° F] if sent within a week), and the convalescent-phase specimen should be shipped on ice (or in a rigid container without ice if next-day delivery is possible).33
Despite all this, however, dengue infections are often diagnosed clinically because laboratory-based diagnosis is often unavailable and has limitations as well.184 That said, dengue should be considered only after other common tropical infections such as malaria are ruled out.184 History is of utmost importance; symptoms in a traveler or immigrant that begin 2 weeks after departure from an endemic area cannot be attributed to dengue, because the incubation period is less than 2 weeks.158
Treatment and Prevention
Treatment includes supportive therapy, including aggressive fluid and electrolyte resuscitation. Early recognition is important, because the acute increase in vascular permeability may cause extreme amounts of plasma to be lost from vascular compartments for 24 to 48 hours. Fever should be treated with antipyretics (especially in children to avoid febrile seizures), and WHO recommends treatment with acetaminophen (paracetamol) if the temperature is greater than 39° C (102.2° F), with dosing as follows: for infants less than 1 year of age, 60 mg per dose; 1 to 3 years of age, 60 to 120 mg per dose; 3 to 6 years of age, 120 mg per dose; and 6 to 12 years of age, 240 mg per dose. WHO recommends no more than six doses in a 24-hour time period, and advises against salicylates to prevent bleeding complications and Reye-like syndrome in children.187
Volume expanders can include normal saline, Ringer’s lactate, or 5% glucose diluted in half-normal or normal saline administered in 10 to 20 mL/kg boluses initially, and then continued with maintenance therapy as needed. One recent double-blind randomized study suggests that Ringer’s lactate at 25 mg/kg over 2 hours should be given for initial resuscitation in children with moderately severe DSS, and that 6% hydroxyethyl starch (also in the amount of 25 mg/kg) may be preferable in children with severe shock.185 Corticosteroids have also been attempted in DSS for their antiinflammatory properties. However, one recent Cochrane meta-analysis of 284 patients demonstrated no reductions in mortality, length of hospital stay, DSS-related complications, or need for blood transfusions when comparing patients who received corticosteroids with those those who received placebo.138 Because of plasma loss, the hematocrit may initially increase, but blood transfusion should be given if there is a clinical indication of internal bleeding. WHO recommends fresh-frozen plasma and concentrated platelets only if there is massive risk for bleeding from coagulopathy. At the same time, because of the volume resuscitation, a drop in hematocrit does not automatically indicate internal hemorrhage, because reabsorption of extravasated plasma may occur when shock ceases. Continued overzealous administration of fluids at this point can lead to pulmonary edema or heart failure. All these measures must be based on clinical judgment, in turn based on indicators such as vital signs, urine output, and hematocrit.187
Despite ongoing vaccine initiatives, there is not yet a licensed vaccine against dengue viruses. As discussed above, a primary infection with one serotype induces long-term protective immunity to reinfection with the homologous serotype but not the heterotypic serotypes. In addition, infection of any serotype can lead to DHF, and sequential exposure to DF may predispose a person to DHF. Thus a successful vaccine must induce long-lasting neutralizing antibodies to all four dengue serotypes. Tetravalent vaccines that enable immunity to all four serotypes are in development and have shown promising results in preliminary clinical testing.58,129 However, vaccine development remains difficult, because administration of four live viruses can result in competition between serotypes and incomplete stimulation of neutralizing antibodies. At this point, vector control through environmental, biologic, and chemical means remains a mainstay of prevention (see information regarding vector control in Global Programs, later).
Yellow Fever
The first epidemic caused by the yellow fever virus is thought to have been in the Yucatan Peninsula in 1648. This single-stranded RNA flavivirus causes infection in up to 200,000 persons per year, with an estimated 30,000 deaths annually, mainly in Africa (90% of cases) and South America (10% of cases).8,182
Epidemiology and Transmission
Unfortunately, factors such as increased urbanization and waning yellow fever immunization programs have contributed to resurgence of the disease since the 1980s.8,150 Accurate data regarding the burden of disease are difficult to obtain because of underreporting (especially in remote areas), lack of diagnostic facilities, and delayed recognition of outbreaks. Nevertheless, of particular concern are the increasing incidence of yellow fever in West Africa (which includes multiple countries suffering humanitarian crises that hinder effective immunization campaigns) and the high (>50%) case fatality rate in South America. Given such concerns, the Yellow Fever Initiative was launched in 2006 as a collaborative effort toward improved yellow fever surveillance and prevention through mass vaccination campaigns.193,194 Figures 48-11 and 48-12 show the most recent endemic areas in Africa and the Americas.
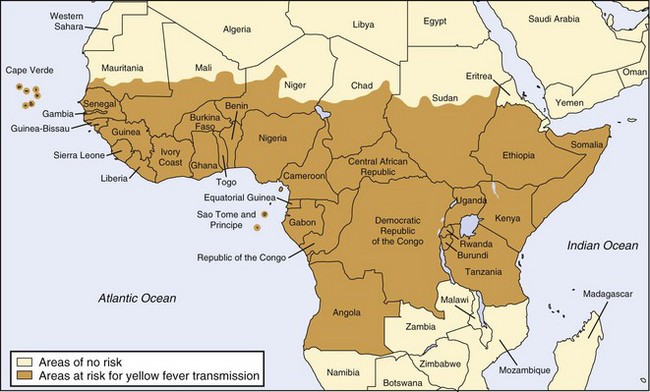
FIGURE 48-11 Yellow fever–endemic zones in Africa, 2009.
(From http://wwwnc.cdc.gov/travel/yellowbook/2010/chapter-2/yellow-fever.aspx#668.)
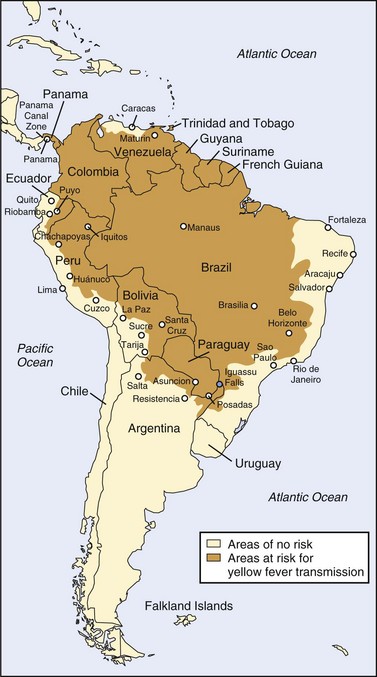
FIGURE 48-12 Yellow fever–endemic zones in the Americas, 2009.
(From http://wwwnc.cdc.gov/travel/yellowbook/2010/chapter-2/yellow-fever.aspx#668.)
Yellow fever in travelers to South America and Africa has been very rare, likely due to routine vaccination. Between 1970 and 2002, only 10 cases of yellow fever were reported, 9 of which occurred in unvaccinated individuals.38
The mosquito species A. aegypti was hypothesized as early as 1881 to be the vector, and it is indeed one of the main mosquitoes that transmit yellow fever in urban settings. There are three patterns of yellow fever transmission: urban, intermediate, and sylvatic (jungle). In urban yellow fever, no monkeys are involved in transmission, and domestic mosquitoes, such as A. aegypti, carry the virus from person to person. In intermediate yellow fever, mosquitoes of the genus Haemagogus, or other forest-canopy species, are known to transmit this disease to both monkeys and humans; this is the most common type of transmission in recent outbreaks in Africa. Finally, in sylvatic yellow fever, nonhuman primates, such as monkeys, are the zoonotic focus, and wild mosquitoes can become infected by the monkeys and pass the disease to humans.38,190
Interestingly, there is also vertical transmission of the virus within mosquitoes; a female mosquito can pass the virus to her offspring. Thus mosquitoes serve as additional reservoirs and vectors of the virus, which ensures endemicity of the disease.182 Peak transmission in Central and South America is during the rainy season between January and March, whereas in Africa it is highest through the end of the rainy season into the early dry season (July through October).38
Clinical Presentation
The main risk factor for disease is exposure, as evidenced by the fact that a large portion of cases are in men of ages 15 to 45 years who have occupational exposure in mosquito-infested areas. Unvaccinated individuals (such as persons too young to have been immunized) in epidemics are also at higher risk, as are individuals in areas with poor health care.182
There are three phases in the classic description of yellow fever: infection, remission, and intoxication. After an incubation period of 3 to 6 days, the infection stage consists of sudden onset of fever, headache, photophobia, malaise, back and epigastric pain, anorexia, and vomiting. Helpful clinical signs include Faget sign (bradycardia occurring at the height of the fever), conjunctival injection, and a coated tongue with pink edges. The infection stage may resolve rapidly within 3 to 4 days and be followed by up to 48 hours of remission.8,128,182
The intoxication period occurs in an estimated 15% or more of individuals infected with this virus, manifesting with liver and renal failure (jaundice, azotemia, and oliguria), myocardial involvement, encephalopathy, and shock. Viral-induced thrombocytopenia and acquired coagulopathy can cause severe hemorrhage, ranging from epistaxis, metrorrhagia, and gastrointestinal bleeding (the reason Spanish-speaking peoples called the disease vomito negro, or black vomit, before the disease was officially named).16 Severe illness has a case fatality rate of about 20%, which may approach 50% in some outbreaks.117
Diagnosis
Preliminary diagnosis is based on clinical presentation and travel history. However, given the clinical overlap with several other disease processes (e.g., leptospirosis, louse-borne relapsing fever, DHF, viral hepatitis), virus detection or serologic examination is required for definitive diagnosis. Laboratory findings may also be supportive in making the diagnosis. They may include leukopenia with relative neutropenia; thrombocytopenia with reduction of all liver-produced clotting factors; elevation of liver enzymes (but usually a normal alkaline phosphatase); elevated blood urea nitrogen (BUN) and creatinine levels; and albuminuria.128
Definitive diagnosis is typically obtained by IgM ELISA and is best done with paired acute and convalescent sera. The acute sample should be drawn within several days of symptom onset, and the convalescent sample should be drawn about 14 days later. A neutralization test, which is done by showing loss of infectivity through reaction of the virus with a specific antibody, can also be done to confirm the diagnosis of yellow fever. Given cross-reactivity between antibodies from other flaviviruses, such confirmatory tests are especially important in Africa, where multiple flaviviruses coexist. The virus can be isolated from serum specimens or liver tissue after death, via inoculation of mice, mosquitoes, or cell cultures, but these techniques are most effective in the preicteric state. Once the intoxication period begins, the viral RNA is usually undetectable and thus such methods are generally not used for diagnosis. Other methods, including PCR for viral genome identification and liver biopsy for demonstration of characteristic midzonal necrosis, have been discussed but are not used for diagnosis.7,38,128,182
Treatment and Prevention
Although there is no treatment other than supportive care, there is a vaccine against yellow fever (17D-204 strain YF-VAX); 0.5 mL of the reconstituted vaccine is administered subcutaneously.42 This vaccine is a live, attenuated vaccine that is generally considered very effective and safe. Seroconversion rates exceed 95%, and side effects are usually limited to injection site pain, mild headaches, myalgias, or low-grade fevers for several days.9,65,128,131 As more travelers enter endemic areas without receiving the vaccine, the number of yellow fever cases imported into the United States and Europe has increased.117 The CDC roughly estimates the risk for acquiring yellow fever for an unvaccinated traveler going to endemic areas of West Africa and South America to be 50 per 100,000 and 5 per 100,000, respectively.38 The CDC and the Advisory Committee on Immunization Practices recommend that travelers (older than 9 months) to certain areas in Africa and South America be vaccinated. Some countries (not the United States) require vaccination before allowing travelers to enter from endemic areas. Vaccination guidelines (and authorized centers for vaccination) can be found on the CDC Yellow Book website at http://wwwnc.cdc.gov/travel/yellowbook/2010/chapter-2/yellow-fever.aspx.
International travelers must receive yellow fever vaccines approved by WHO and administered at an approved yellow fever vaccination center and then obtain an international certificate of vaccination. Although the International Health Regulations require boosters every 10 years, many studies suggest that vaccine immunity endures for over 30 years and possibly for life.190
Immediate hypersensitivity reactions in people with allergies to eggs or other substances are thought to occur in 1 per 131,000 vaccinations, whereas anaphylaxis is reported to occur in 1.8 per 100,000 doses administered. Additional significant adverse reactions to the yellow fever vaccine typically occur in first-time vaccinees and are categorized as yellow fever vaccine–associated neurotropic disease (YEL-AND) and yellow fever vaccine–associated viscerotropic disease (YEL-AVD). YEL-AND is typically due to neuroinvasion by the 17D virus and represents multiple different clinical syndromes, including Guillain-Barré syndrome and meningoencephalitis. Historically the vaccine was associated with encephalitis in children, but standardization of the virus vaccine has decreased the incidence in the United States to 0.8 case per 100,000 doses administered. Although the incidence is thought to be higher in the older adult population, the case fatality rate remains low.9,38 YEL-AVD is a severe illness resembling wild-type disease, in which vaccine virus replication often leads to multisystem organ failure and death. The incidence in the United States is 0.4 cases per 100,000 doses administered, but host factors such as increased age, history of thymus disease, and male gender are thought to increase the risk. The case fatality rate remains a staggering 53%.9,38 Caution is advised in infants less than 9 months old; in individuals with a history of hypersensitivity to vaccine products, thymus disease, or human immunodeficiency virus (HIV); and in individuals who are pregnant, nursing, immunocompromised, older adults, or receiving other vaccines simultaneously.9,38,42 The CDC encourages health care providers to report febrile illness thought to be caused by the yellow fever vaccination to the CDC/FDA Vaccine Adverse Events Reporting System (VAERS) at http://vaers.hhs.gov/esub/index.
Eradication of A. aegypti via well-executed control campaigns in the 1970s has been successful in many Central and South American countries, with much credit owed to the Pan American Health Organization. However, vigilance must be maintained, because A. aegypti has reestablished itself in many of these areas. Much work must be done in Africa, where the mosquito has never been eradicated.117
Japanese Encephalitis
The Japanese encephalitis (JE) virus, a member of the Flavivirus genus, is the major cause of viral encephalitis in Asia, with as many as 50,000 cases reported every year. Although JE virus is a leading cause of viral encephalitis in Asia, there is less than one case per year in U.S. civilians and military personnel living in or traveling to Asia, with rare outbreaks in U.S. territories in the western Pacific. There has been more recent expansion of JE into northern Australia (Figure 48-13).39,40,81,135
Epidemiology and Transmission
Culex mosquitoes (usually Culex tritaeniorhynchus, C. vishnui, and C. pseudovishnui) are the vectors for this virus, and vertebrate hosts such as pigs and birds sustain the virus. Because humans are not necessary for transmission, the virus can still be maintained in an enzootic cycle even where JE immunity is high (either in endemic regions or through vaccinations) and human cases do not occur. The mosquitoes breed in flooded fields associated with rice production. Because these settings contain factors that enhance the JE transmission cycle (e.g., large numbers of pigs and birds to serve as reservoirs for infection), JE infection rates may be as high as 3% in these areas.117
JE has two main transmission patterns in Asia. Seasonal transmission occurs in the more temperate northern regions (e.g., Japan, People’s Republic of China, Taiwan, northern India) and can cause large epidemics. The second pattern of year-round transmission presents in tropical southern regions (e.g., southern Thailand, southern Vietnam, southern India, Indonesia, Malaysia, Philippines, Sri Lanka) and is usually more endemic or sporadic.54,117,159
Clinical Presentation
Persons at increased risk include individuals (residents, active duty military, or expatriates) living in endemic areas. Travelers have a low risk for acquiring the disease, estimated to be less than one in a million American travelers going to endemic areas.19 Most residents of endemic areas are infected during childhood or early adulthood,86 with about 10% of the susceptible population infected yearly. The disease appears to affect mainly children less than 15 years of age, although there also seems to be a secondary increase of disease in older adults, posited to be caused by waning immunity.19
The incubation period is from 5 to 15 days. Most infections with JE are subclinical. Early symptoms include sudden onset of fever, headache, nausea and vomiting, and abdominal pain. JE may also manifest with coryza, diarrhea, and rigors. In some individuals, abnormal behavior is the only clinical manifestation, so JE has been misidentified as mental illness.163 In the Korean conflict, for example, American military personnel with JE were sometimes diagnosed with “war neurosis.”113 After several days, JE may develop into aseptic meningitis with or without encephalopathy, or into encephalitis (less than 1% of infected individuals) presenting with delirium and abnormal motor movement. Children in particular seem to have more convulsions than do adults infected with the virus.56,107,126,164