Molecular Techniques
At the conclusion of this chapter, the reader should be able to:
• Describe the composition of DNA and RNA.
• Compare the functions of DNA and various forms of RNA.
• Describe the polymerase chain reaction (PCR) amplification technique.
• Compare various PCR modifications.
• Identify and briefly describe other amplification techniques.
• Describe the gold standard of genetic analysis.
• Compare DNA sequencing and branched DNA protocols.
• Identify and compare three hybridization techniques.
• Explain how microarrays are applied to immunologic testing.
• Discuss the general concept of nucleic acid blotting.
• Compare the characteristics and clinical applications of Southern, Northern, and Western blotting techniques.
• Analyze a case study related to immunoassay.
• Correctly answer case study related multiple choice questions.
• Be prepared to participate in a discussion of critical thinking questions.
Characteristics of Nucleic Acids
DNA and RNA are polymers made up of repeating nucleotides or bases that are linked together (Fig. 14-1). DNA and RNA have the same two purine bases, adenine (A) and guanine (G), but the pyrimidine bases differ. DNA has cytosine (C) and thymine (T); RNA substitutes uracil (U) for T. DNA is predominantly a double-stranded molecule with specific base pairs linked together (Fig. 14-2). Nucleotides are bonded together and two strands are twisted into an alpha helix (Fig. 14-3).
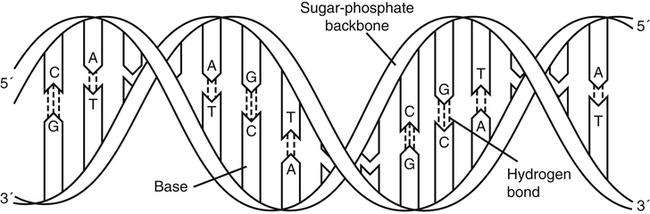
The DNA molecule is a double helix that consists of two sugar-phosphate backbones with four bases—cytosine (C), guanine (G), adenine (A), and thymine (T)—attached. C and G residues and A and T residues on opposite strands pair through hydrogen bonding. (From LeGrys V, Leinbach SS, Silverman L: Clinical applications of DNA probes in the diagnosis of genetic diseases, Crit Rev Clin Lab Sci 25:255, 1987.)
How Does DNA Replicate?
DNA is a very stable molecule and replication is straightforward. The process of replication (Fig. 14-4) involves one strand of the molecule acting as a template for the creation of a complementary strand. As a result of this process, two identical daughter molecules are produced. In the laboratory, the hydrogen bonds that hold the strands of the double helix can be broken apart or denatured. If complementary strands of DNA are denatured in the laboratory, they can spontaneously rejoin, or anneal. The process of denaturation and annealing (see later discussion) can be used effectively in molecular testing.
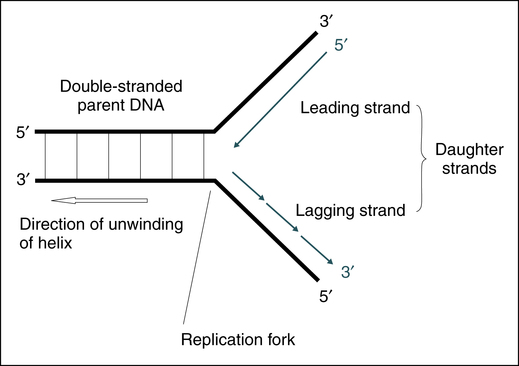
Double-stranded DNA is separated at the replication fork. The leading strand is synthesized continuously, whereas the lagging strand is synthesized discontinuously but joined later by DNA ligase. (From Burtis CA, Ashwood ER, Bruns DB: Tietz fundamentals of clinical chemistry, ed 6, St Louis, 2008, Saunders.)
Forms of RNA
• mRNA—translates DNA coding into functional proteins (Fig. 14-5)
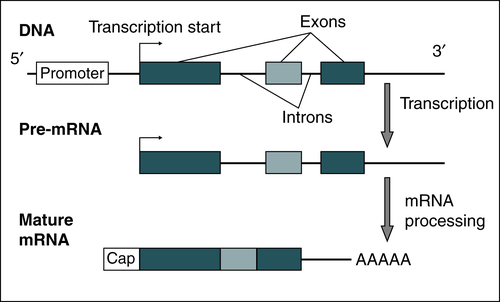
A gene that encodes for a protein contains a promoter region with a variable number of introns and exons. Transcription commences at the transcription start site. Pre-mRNA is processed by capping, polyadenylation, and intron splicing and becomes a mature mRNA. (From Burtis CA, Ashwood ER, Bruns DB: Tietz fundamentals of clinical chemistry, ed 6, St Louis, 2008, Saunders.)
• tRNA—transports various amino acids to manufacture proteins
Amplification Techniques in Molecular Biology
Polymerase Chain Reaction
The polymerase chain reaction (PCR) is an in vitro method that amplifies low levels of specific DNA sequences in a sample to higher levels suitable for further analysis (Fig. 14-6, A). To use this technology, the target sequence to be amplified must be known. Typically, a target sequence ranges from 100 to 1000 base pairs (bp) in length. Two short DNA primers, typically 16 to 20 bp, are used. The oligonucleotides (small portions of a single DNA strand) act as a template for the new DNA. These primer sequences are complementary to the 3′ ends of the sequence to be amplified.
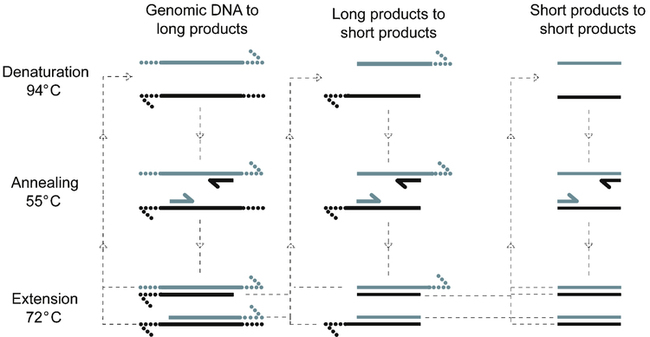
Repetitive cycles of denaturation, annealing, and extension are paced by temperature cycling of the reaction. Two primers indicated as short segments anneal to opposite template strands (long line) to define the region to be amplified. Extension occurs from the 3′ ends (half-arrowheads). In each cycle, genomic DNA is denatured and annealed to primers that extend in opposite directions across the same region, producing long products of undefined length. Long products generated by extension of one of the primers anneal to the other primer during the next cycle, producing short products of defined length. Any short products present also produce more short products. After n cycles, up to 2n new copies of the amplified region are present—n long products and (2nn − n) short products plus one original genomic copy. A similar approach can be used to amplify RNA targets by initial reverse transcription of the RNA template to produce the DNA template. (From Burtis CA, Ashwood ER, Bruns DB: Tietz fundamentals of clinical chemistry, ed 6, St Louis, 2008, Saunders.)
This enzymatic process is carried out in cycles. Each repeated cycle consists of the following:
• DNA denaturation. Separation of the double DNA strands into two single strands through the use of heat.
• Primer annealing. Recombination of the oligonucleotide primers with the single-stranded original DNA.
• Extension of primed DNA sequence. The enzyme DNA polymerase synthesizes new complementary strands by the extension of primers.
Three important applications of PCR are as follows:
The PCR technique has undergone modifications (see Fig. 14-6, B). One uses nested primers in a two-step amplification process. First, a broad region of the DNA surrounding the sequence of interest is amplified, followed by another round of amplification to amplify the specific gene sequence to be studied. Another PCR modification successfully differentiates alleles of the same gene.