Modes of Mechanical Ventilation
I Pressure and Volume Targeting (Table 39-1)
TABLE 39-1
Pressure- Versus Volume-Targeted Ventilation
Pressure | Volume | |
Peak airway pressure | Constant | Variable |
Peak alveolar pressure | Constant | Variable |
Tidal volume | Variable | Constant |
Peak flow | Variable | Constant |
Flow pattern | Decelerating | Preset |
Inspiratory time | Preset | Preset |
Minimum rate | Preset | Preset |
A Regardless of terminology used to identify a mode of ventilation, all modes operate by targeting pressure, volume, or both of these variables.
B With volume targeting, a specific volume of gas is delivered each tidal volume (Vt). As a result
2. Peak flow or inspiratory time must be selected.
3. A gas flow pattern must be set.
a. Square wave and decelerating wave flow patterns are available on current generation ventilators (Figure 39-1).
b. With square wave flow, the set flow is constant throughout inspiration.
c. With a decelerating wave flow pattern, the flow decreases from the maximum set flow to a minimal end-inspiratory flow.
d. The minimal flow in decelerating wave is variable from ventilator to ventilator and in some ventilators can be adjusted.
e. The flow waveform affects peak flow and the inspiratory time required to deliver a given Vt.
f. If the peak flow is constant, inspiratory time must be longer with a decelerating flow pattern than with a square wave flow pattern.
g. If inspiratory time is constant, peak inspiratory flow must be higher with a decelerating flow pattern than with a square wave flow pattern.
h. When changing flow pattern care must be exercised to ensure that proper peak flow or inspiratory time is set.
4. Pressure is the resultant variable, and it can vary considerably from breath to breath.
C With pressure targeting, maximum preset peak airway pressure is set, and this limits peak alveolar pressure to the set level. As a result
1. Maximum airway and alveolar pressures are targeted.
2. Vt can vary from breath to breath.
3. Gas flow delivery is variable (Figure 39-2).
a. A high initial flow is delivered. The rate of initial flow delivery is based on set peak pressure, the patient’s lung mechanics, and patient demand.
b. Peak flow must rapidly decrease as the target pressure is reached to prevent pressure from exceeding the target level.
(1) The higher the set pressure, the higher the initial flow.
(2) The higher the patient demand, the greater the initial flow.
(3) The higher the resistance and the lower the compliance, the lower the initial flow.
c. To prevent pressure from exceeding the target, flow may decrease to zero before the end of inspiration.
d. Inspiratory time may be set or the breath may end when inspiratory flow decreases to a predetermined level.
D With pressure and volume combined targeting modes, the ventilator attempts to ensure a preselected maximum pressure is never exceeded and a preselected Vt is delivered.
1. Gas delivery is usually in a pressure-targeting format but may change to a volume format during the breath in some modes.
2. A high initial flow is delivered that rapidly decelerates over time.
3. System pressure is maintained below the maximum selected but may change on a breath-to-breath basis to ensure that the Vt selected is delivered.
E In all of these different gas delivery formats
F Pressure- and volume-targeted breaths respond differently to changes in compliance and resistance of the respiratory system (Table 39-2).
TABLE 39-2
Effect of Changing Compliance and Resistance During Pressure and Volume Ventilation
Pressure | Volume | |
Decreased compliance | ↓ Volume | ↑ Pressure |
Increased compliance | ↑ Volume | ↓ Pressure |
Increased auto-PEEP | ↓ Volume | ↑ Pressure |
Decreased auto-PEEP | ↑ Volume | ↓ Pressure |
Pneumothorax | ↓ Volume | ↑ Pressure |
Bronchospasm | ↓ Volume | ↑ Pressure |
Mucosal edema | ↓ Volume | ↑ Pressure |
Secretions | ↓ Volume | ↑ Pressure |
Pleural effusion | ↓ Volume | ↑ Pressure |
Increased patient effort | ↑ Volume | ↓ Pressure |
Decreased patient effort | ↓ Volume | ↑ Pressure |
1. Any factor that increases resistance or decreases compliance during volume ventilation causes the peak airway and peak alveolar pressure to increase.
2. Any factor that decreases resistance or increases compliance during volume ventilation causes the peak airway and peak alveolar pressure to decrease.
3. Any factor that increases resistance or decreases compliance during pressure ventilation causes VT to decrease.
4. Any factor that decreases resistance or increases compliance during pressure ventilation causes VT to increase.
G During pressure and volume ventilation, effective static compliance can be measured if a static condition is achieved (Figure 39-3).
1. With volume ventilation an end-inspiratory pause is set, allowing all airflow to cease. Effective total respiratory system compliance is calculated by:
< ?xml:namespace prefix = "mml" />

a. Volume compressed in the ventilator circuit must be subtracted from the ventilator set volume to identify the actual Vt entering the airway. Some ventilators automatically correct for compressed volume in the ventilator circuit. Normally approximately 1 to 2 ml/cm H2O plateau pressure minus total PEEP is compressed in the ventilator circuit.
b. Total PEEP (applied plus any additional auto-PEEP) is subtracted from the plateau pressure to ensure that only the actual pressure needed to maintain the Vt in the lung is identified.
2. During pressure ventilation, compliance can be calculated if the flow rate returns to zero 200 to 300 msec before the end of the breath (see Figure 39-2, A). If this occurs the set pressure level is equal to the plateau pressure, which is equal to the average peak alveolar pressure.

H Estimation of airway resistance can be determined during volume ventilation but not pressure ventilation (see Figure 39-3).
1. To calculate airway resistance during mechanical ventilation, a constant gas flow rate must be provided.
2. Constant flow (square wave) is only possible during volume ventilation.
3. When flow is constant the pressure difference between the peak pressure and the plateau pressure is the pressure required to overcome airway resistance.

A All modes of ventilation on current generation ventilators allow the patient to trigger (activate) gas delivery.
B Controlled ventilation, the absence of patient active interaction with the ventilator, can only truly be established by sedating the patient to apnea.
C The sensitivity control on ventilators allows the clinician to determine the amount of patient effort required to trigger a ventilator-assisted breath.
D Even at sensitivity settings requiring large inspiratory efforts, patients with strong ventilatory demands can trigger a breath.
E Ventilator triggering can occur by two mechanisms: change in pressure or flow (Figure 39-4).
1. Traditionally all ventilator modes were pressure triggered.
2. To activate a breath the patient had to decompress the ventilator circuit a sufficient level to overcome the sensitivity setting.
3. The major problem with pressure triggering is the location of pressure sensing.
a. If pressure is sensed at the ventilator, pressure at the wye of the ventilator circuit must be less than that measured at the ventilator.
b. If the sensitivity is set at −2 cm H2O the patient may have to establish a −4 cm H2O pressure at the wye.
c. If pressure is measured at the circuit wye this concern is eliminated.
d. With pressure triggering pressure changes before flow is provided.
e. During flow triggering either the patient must divert a set flow from a low continuous flow or generate a specific flow through a pneumotachograph placed at the airway.
f. As a result of the design of flow-triggered systems they tend to minimize effort to a greater extent than pressure triggering.
g. With flow triggering flow is established before the ventilator generates airway pressure.
h. Less effect is required to trigger a ventilator set at 2 L/min flow triggering versus −2 cm H2O pressure triggering.
i. Regardless of the trigger available on a given ventilator, trigger sensitivity should always be set as sensitive as possible to avoid auto-triggering and to minimize patient effort.
j. With pressure triggering, water in the ventilator circuit tends to increase auto-triggering.
k. With flow triggering, leaks in the system tend to increase auto-triggering. This also occurs in pressure triggering when PEEP is set.
l. All factors being equal, flow triggering seems to be the most efficient method of triggering a breath.
A This mode of ventilation can be provided in pressure and volume targeting (Figures 39-5 and 39-6).
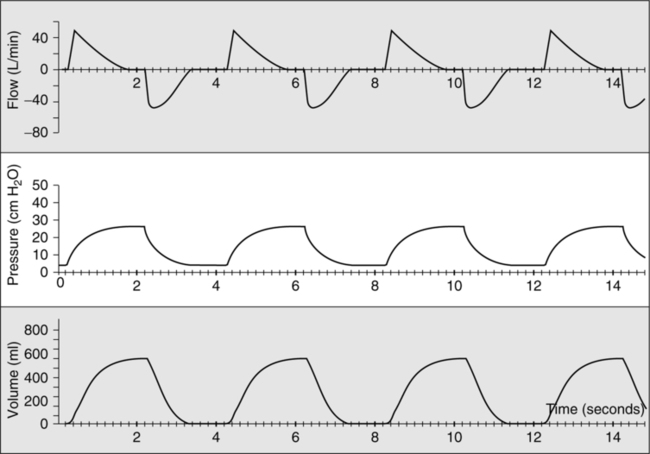
B The basic operation is that every breath is a positive-pressure breath triggered by the patient.
C If the patient does not trigger the breath, the ventilator automatically delivers a back-up breath (controlled breath).
D Volume-targeted assist/control (AC) mode is the most common mode of ventilation used worldwide.
E During volume AC the following variables must be set.
a. Setting of peak flow along with Vt and flow waveform determines the inspiratory time.
b. Peak flow in assisted ventilation is set to meet patient’s inspiratory flow demand (Figure 39-7). If peak flow is set too low, patients may be required to perform a large amount of the work of breathing.
c. Figure 39-7 depicts the airway pressure waveforms during controlled and assisted ventilation in a patient with all ventilator settings constant.
d. During controlled ventilation the area under the airway pressure curve is equal to the total work required to ventilate a patient.
e. During assisted ventilation the same amount of work must be done, but the patient now shares the work.
f. As a result the area in Figure 39-7 (bottom) identified as subject work can be high if the peak flow is inadequate.
g. Figure 39-8 indicates an airway pressure waveform in which the peak flow is grossly inadequate, indicating excessive patient work.
h. The more the actual airway pressure waveform deviates from the expected airway pressure waveform (see Figure 39-8), the greater the work performed by the patient.
i. Peak flow frequently needs to be set ≥80 L/min to meet patient peak inspiratory demands and minimize the work of breathing.
4. Inspiratory time (set on some ventilators)
a. During assisted ventilation patients usually require an inspiratory time ≤1.0 seconds.
b. It is unusual for spontaneously breathing patients to desire an inspiratory time >1.0 second.
c. During controlled ventilation inspiratory time can be lengthened (see inverse ratio ventilation).
6. In some ventilators the inspiratory to expiratory (I:E) ratio is set.
a. The I:E ratio is the relationship between inspiratory and expiratory time.
b. During normal breathing I:E ratios are approximately 1:2.
c. In patients with obstructive lung disease I:E ratios are smaller, 1:3 to 1:6 or more.
d. Most do not set I:E ratio, preferring to set inspiratory time directly or indirectly by setting peak flow and rate.
F Pressure AC essentially functions the same as volume AC except by pressure targeting.
G During pressure AC the following variables must be set.
4. With some units I:E can be set.
8. Rise time (see later section)
a. Inspiratory time is usually ≤1.0 seconds in patients actively triggering the ventilator.
b. It is unusual for patients to desire an inspiratory time >1.0 second when spontaneously breathing.
c. During control ventilation inspiratory time can be lengthened; however, it is questionable whether this is beneficial (see inverse ratio ventilation).
IV Synchronized Intermittent Mandatory Ventilation
A Figure 39-9 illustrates a typical volume-targeted square wave flow synchronized intermittent mandatory ventilation (SIMV) breath.
B With this mode of ventilation a mixture of spontaneous and mechanically assisted breaths is provided.
C SIMV can be provided using volume- or pressure-targeted ventilation for the mandatory breaths.
D During the spontaneous breaths pressure support (PS; see Section VI, Pressure Support) can be added (Figure 39-10).
E In SIMV a mandatory rate is set and provided in an AC format. However, only the set number of mandatory breaths is provided.