Mobilization and Exercise
Physiological Basis for Assessment, Evaluation, and Training
The routine application of mobilization and exercise should be distinguished from the prescriptive application of mobilization and exercise; the three objectives of prescribing mobilization and exercise for the purpose of maximizing oxygen transport are differentiated in this chapter. The detrimental multisystem effects of prolonged sedentary activity and restricted activity (bed rest) in the general population are outlined, and techniques to counter those effects are described. The acute and chronic responses to exercise described in this chapter are typical for individuals without pathology. Chapter 19 outlines testing and training prescription principles and practical details for exercise testing and prescription. Special considerations in common patient populations with respect to exercise testing and training prescription are described in Chapters 24 and 25.
Terminology in Mobilization and Exercise
Mobilization
Mobilization is the therapeutic and prescriptive application of low work load activity in the management of cardiovascular and pulmonary dysfunction. Primarily, the goal of mobilization is to exploit the acute effects of exercise in order to optimize oxygen transport. Although this activity is considered low work load, it can elicit high patient-specific relative metabolic demand. Even a relatively low dose of a mobilization stimulus can impose considerable metabolic demand on a patient with cardiovascular or pulmonary compromise, which is why it is prescribed for patients who are acutely ill. Mobilization is also used because of its beneficial effects on other organ systems such as the musculoskeletal, neurological, integumentary, gastrointestinal, and renal systems. Whenever possible, mobilization is performed in the upright position, which is the physiological position (Chapter 20), to optimize gravitational stress on fluid shifts and central and peripheral hemodynamics. Mobilization is thus prescribed as both a gravitational stimulus and an exercise stimulus.
Training
Although specific principles of training have been designed for athletes involved in performance-based activities, these principles also apply to any patient that physical therapists encounter. Training in both cases, is defined as the systematic application of progressive exercise stimuli to elicit a specific physiological, functional, or skill-based goal (or goals). Typically, to achieve specific patient-based goals, the application of multiple exercise modes and training guidelines is required. This type of training often involves both aerobic and anaerobic exercise using cyclic and resistance-based exercises. Application of training principles to exercise testing and prescription is discussed further in Chapter 19.
Basic Principles of Mobilization and Exercise
Exercise as an Assessment and Evaluation Tool
Physiological response can vary in deconditioned people where a given work rate is perceived as highly strenuous, yet the absolute work rate is relatively low. These responses can be markedly altered in people with a range of pathologies (depending on their types and severities) and these differences need to be anticipated and considered (see Chapters 24 and 25) in the assessment of a patient.
For evaluation, exercise tests can serve as standardized outcome measures. As evaluation tools, tests are repeated periodically (e.g., every few weeks) in order to progress the exercise or training prescription until the target conditioning goal is achieved. Special attention is paid in these cases to pre-exercise conditions and the standardization of both exercise procedures and measurement procedures (Chapter 19).
Often for people with chronic conditions, many of whom may be older, submaximal tests can be used to compare responses from one time to another for given work rates. In other cases, the physical therapist may wish to estimate by conducting a
test, which can be done directly or indirectly. Direct measurement of
requires metabolic measurement with a cart and capacity for expired gas analysis for O2 and CO2 (Figure 18-1). Such carts require pretest gas calibration in addition to calibration of the exercise modality (treadmill or ergometer). The patient needs to be familiarized and habituated to the tester(s) and testing situation, and practice beforehand, to ensure the results are valid. Indirect assessment of
is imprecise but can provide an index of general conditioning if methods are tightly standardized; this is particularly important when test results are used as outcome measures. Practices related to exercise testing and training prescription are detailed in Chapter 19.
Oxygen Transport and Metabolic Demand of Patients
In patients whose oxygen transport capacity is reduced or threatened, mobilization and exercise constitute a metabolic load that is superimposed on other factors that increase metabolic cost (Table 18-1). Hospitalized patients may be hypermetabolic. In addition to their basal metabolic demands, their energy demands are increased secondarily to factors such as pain, anxiety, increased body temperature, healing and repair processes, increased work of breathing, and increased work of the heart, as well as in response to interventions and procedures such as physical therapy. The goal, therefore, in prescribing mobilization and exercise is to ensure a safety margin wherein the patient’s demand for oxygen does not exceed the available normal supply or delivery. This can be indicated clinically if the patient’s oxygen transport levels worsen by either objective or subjective measurement.
Table 18-1
Factors That Contribute to Increased Metabolic Demand and Oxygen Consumption in Patient Populations
Type of Factors | Specific Factors |
Pathophysiological Factors |
Alerting, noxious, and painful responses to routine nursing, medical, and physical therapy interventions, including injections, insertion of lines, procedures, and neurological checks
Feeding, enterally or parenterally
Being handled physically (by health care workers)
Changing body position (passive, active assist, or active changes)
Range of motion exercises (passive, active assist, or active)
Several interventions can increase and overall metabolic demand;1,2 therefore the capacity of the oxygen transport system to meet the patient’s metabolic demands needs to be established in the assessment. These interventions include:
Metabolic demand and are determined by many factors. In patients, the effect of arousal, anxiety, pain, and noxious stimulation, in addition to the hypermetabolic demands of recovery, contribute to energy cost and demand on the oxygen transport system. Thus relaxing and calming patients are central components of physical therapy because these interventions reduce oxygen demand, as do physical therapy interventions for pain management. Although relaxation, often coupled with sedation or analgesia, is central to physical therapy management in other settings, relaxation procedures should be applied in the management of patients with cardiovascular and pulmonary dysfunction in situations in which agitation, anxiety, and pain contribute to increased oxygen demand. This is especially true in intensive care, where oxygen transport is compromised or threatened in most patients (see Chapters 34 and 35).
The typical method for prescribing exercise to achieve desired acute and long-term adaptations is longer duration, whole-body aerobic exercise at a moderate-vigorous intensity. This type of stimulus appropriately optimizes both central (cardiac, blood volume, oxygen delivery) and peripheral (oxygen extraction, glycolytic/mitochondrial enzyme function, capillarization) components for increasing . This type of training, however, also requires an adequate central response of the cardiovascular and/or respiratory systems to produce a high level of cardiac output during exercise. In patients with compromised cardiovascular or respiratory function, this type of exercise is extremely difficult and can often lead to undue fatigue. The stimulus for increasing metabolic demand with exercise can be achieved in ways that can help minimize
, thus reducing the impact of reduced cardiovascular and/or respiratory function.3 For example, in patients with chronic obstructive lung disease, breathing a low-density helium gas mixture relieves some of the respiratory loading, resulting in increases in exercise tolerance4 and peripheral muscle trainability.5 Use of such breathing gases is not usually practical in clinical settings, however. Other methods such as single limb training and high-intensity interval training, have been shown to reduce central demand and optimize peripheral load (see Chapter 19).
Cellular Energetics in Response to Acute Mobilization and Exercise
The integrity of the cardiopulmonary unit is fundamental to maximize oxygen transport and cellular respiration. Oxygen is continuously being used by every cell in the body for oxidative phosphorylation and the synthesis of adenosine triphosphate (ATP). The splitting of a phosphate bond from ATP to form adenosine diphosphate yields a considerable amount of energy for metabolism. The energy for this process comes from the reduction of hydrogen in the formation of water and carbon dioxide, which are the end products of the Krebs cycle and the electron transfer chain (i.e., cellular respiration). These metabolic processes, which make up oxidative phosphorylation, take place in the mitochondria of the cells (see Chapter 2).
The balance between oxygen consumption and oxygen delivery is precisely regulated to ensure not only that there is an adequate supply of oxygen but also that, under typical resting conditions, approximately four times as much oxygen is delivered to the tissues as is used. This safety margin permits the immediate availability of oxygen when the system is perturbed by physical, gravitational, or psychological challenges (see Chapter 2).
Lactic acid accumulates in the blood during anaerobic metabolism. In healthy, untrained individuals lactic acid increases exponentially at an exercise intensity of about 55% of maximal aerobic power.6
The first line of defense is the response to an increase in pericellular pH. The concentration of carbon dioxide is increased, and this, as a result of the decrease in pH, facilitates the dissociation of oxygen from hemoglobin (i.e., it shifts the oxyhemoglobin dissociation curve to the right). CO, the product of stroke volume and heart rate (SV × HR), increases commensurate with metabolic demand. The immediate response to oxygen utilization and lowered tissue oxygen is an increase in CO to increase DO2. This averts arterial desaturation that is not typically observed in health, even with extreme exertion. With progressive exercise intensity, SV increases disproportionately at lower intensities to increase CO, with HR contributing to a greater extent at moderate and high exercise intensities. However, older individuals exercising at high intensity in upright positions rely more on the Frank-Starling effect than on HR.7 In health, exercise-induced cardiac fatigue has been proposed as a limiter to prolonged exercise.8 The mechanisms of the associated reduced left ventricular systolic and diastolic function are unclear.
The increase in systemic CO results in increased venous return and pulmonary CO. The SV plateaus at 40% of , and thereafter CO is augmented by increases in HR (Figure 18-2). One exception in health is in elite athletes, whose SV may continue to increase commensurate with exercise intensity.9 To oxygenate a greater volume of blood in the lungs, an increased volume of air must be inspired. To increase
, tidal volume (VT) and respiratory rate (RR) increase. At low intensities of exercise, VT increases disproportionately to RR, whereas at moderate to high intensities, VT plateaus and further increases in
are effected by an increase in RR (Figure 18-3).10 Exercise is associated with a small increase in airway diameter and length of pulmonary structures, hence, a reduction in airway resistance. Zone 2, the zone of greatest alveolar ventilation to perfusion (
) matching in the lungs, is increased as a result of pulmonary capillary dilation and recruitment. Diaphragmatic excursion is enhanced, and the distributions of ventilation and perfusion become more uniform throughout the lungs, which minimizes airway closure and atelectasis. Exercise increases diaphragmatic excursion, which leads to inflation of the lungs in three planes (anteroposterior, transverse, and cephalocaudal, particularly in the upright position).
First, this action increases alveolar ventilation (
) by a primary increase in VT.
Second, exercise-induced lung motion facilitates lymphatic flow and drainage. Optimal lymphatic drainage is essential for lung water balance with the increased volume of blood being processed through the pulmonary circulation and lung parenchyma. This action may explain, in part, the beneficial effect of mobilization on the distribution and function of pulmonary immune factors.11 The increased movement of the lungs during exercise has a primary effect on mucociliary transport and mucus clearance.12 Physical activity may also minimize bacterial colonization in the airways, hence reducing the risk for pulmonary infection.13
Finally, lung movement stimulates surfactant production and its distribution over parenchymal tissue. Surfactant is essential for reducing surface tension in the alveoli, maintaining alveolar stability, and maintaining lung compliance, thereby minimizing airway closure and areas of lung collapse.
Effects of Mobilization and Exercise on Oxygen Transport
Conversely, the absence of gravitational stress and exercise stress are the two primary factors contributing to impaired oxygen transport secondary to bed rest deconditioning. Exploiting both gravitational and exercise stress to prevent cardiovascular and pulmonary dysfunction is thus indicated. Further, mobilization and exercise are the two most vital physiological interventions available to the physical therapist for remediating cardiovascular and pulmonary dysfunction. Body position has marked effects on pulmonary and cardiovascular function (Chapter 20) and thus on exercise performance. The more acutely ill a patient is, the more important it is that upright body positioning be coupled with mobilization to increase aerobic capacity and tolerance to exercise stress. The degree to which this is done, however, depends on the patient’s status and ongoing monitoring (see Chapters 16, 34, and 35).
The Prescription of Mobilization and Exercise
The prescription of exercise is fundamental to the management of primary and secondary cardiovascular and pulmonary dysfunction. Guidelines have been well developed for prescribing exercise to maximize functional work capacity or aerobic power for healthy people and individuals with chronic heart and lung conditions.14–21
As clinical exercise specialists, physical therapists strive to take advantage of the effects of exercise in most patients and adapt the prescription based on the patient’s clinical presentation and needs. Exercise training is typically based on a person’s responses to a maximum graded exercise test. Although somewhat less precise, exercise training intensity can be prescribed based on a proportion of the individual’s age-predicted maximum heart rate provided there is no significant medical history. Submaximal exercise testing is often indicated for patients with severely impaired functional work capacity because of the inherent risks associated with maximal testing and the potential for invalid test results.22 Submaximal exercise testing has had a greater role in patient assessment and evaluation than as a basis for exercise prescription. Because of the greater utility of submaximal exercise testing in patient populations, guidelines for the latter warrant being better elucidated.
The prescription of mobilization for the patient with acute cardiovascular and pulmonary dysfunction has been a relatively neglected research priority when compared with the number of studies concerning the prescription of exercise. This is surprising, given that early mobilization is generally a key component of cardiovascular and pulmonary physical therapy for patients who are acutely ill. Orlava (1959)23 was among the first to report the beneficial use of mobilization to manage acute cardiovascular and pulmonary compromise; specifically, bronchopulmonary pneumonia. In addition, the physiological literature has long supported an unequivocal role for therapeutic mobilization to maximize oxygen transport in patients with acute cardiovascular and pulmonary dysfunction.24 Even for patients who are critically ill, the goal of mobilization is to evaluate the patient’s responses with respect to oxygen transport reserve capacity and use this as a basis for estimating the limits of a patient’s physiological capacity to be mobilized, with a view to returning the patient to living within the community. The more critically ill the patient, the greater the need to assess the relationship between the patient’s and oxygen delivery (DO2) (see Chapter 2);25 this ensures that the patient’s capacity for oxygen transport is not exceeded.
Unlike exercise testing and prescription for the patient with chronic cardiovascular and pulmonary conditions, the patient with acute dysfunction is not exercise-tested in the conventional manner because of understandable risk and the patient’s incapacity to perform such a test. Given the profound and direct effects of mobilization and exercise on cardiovascular and pulmonary function, the physical therapist needs to identify the specific effects of exercise required and define the optimal therapeutic stimulus (i.e., the stimulus that yields the maximal benefit to oxygen transport with the least risk). The parameters for the mobilization prescription are based on factors other than a maximal or peak effort (see Chapters 16, 34, and 35).
Acute and chronic cardiovascular and pulmonary pathology have the additional challenges of compromising functional capacity in two ways. First, in acute illness, the patient tends to be recumbent in bed a great proportion of the time; second, in both acute and chronic illnesses, the patient’s physical activity is reduced. Recumbency and restricted mobility have physiologically distinct effects on oxygen transport that contribute jointly to bed rest deconditioning; recumbency, however, has been considered to be the primary determinant of bed rest deconditioning.26–28 The impact of these factors is exaggerated in smokers, young people, older adults, obese individuals, and patients who are mechanically ventilated.
The goals of mobilization and exercise are directed at correcting impairments and enhancing the patient’s capacity for activity and participation (see overview of the WHO International Classification of Function, Disability and Health in Chapters 1 and 17). Outcomes related to improving abilities and participation include self-care, home management, return to work, and resumption of avocational activities. Return to work warrants special mention. Return to work is often related to oxygen transport capacity in terms of aerobic and muscle power. However, there are economic, psychosocial, and environmental factors that need to be considered. These factors, too, should be part of the overall assessment and incorporated when setting overall goals and establishing the appropriate outcomes for a given patient.
Preventive Effects of Exercise
The preventive effects of exercise are evident in prehabilitation. The term prehabilitation can be used to refer to patient conditioning before a surgical intervention or hospital admission.29 Individuals can be prescribed exercise programs commensurate with their age, functional status, and anticipated procedure or surgery. The purpose is to reduce complications, speed recovery, minimize the time until discharge, and promote a complete return to daily living as soon as possible.
When prescribing exercise to elicit its optimal preventive effects, the physical therapist needs to consider the same variables as when prescribing exercise for its beneficial acute and long-term effects—that is, the patient’s age, capabilities, premorbid functional work capacity, and the type, distribution, and severity of the patient’s underlying condition(s). Such preventive mobilization or exercise must be prescribed in such a way as to avoid having any deleterious effects on the patient’s overall condition. Even after highly invasive investigative procedures such as heart catheterization, patients can benefit from safe early ambulation.30
Prescription of Prehabilitation Exercise
Although much is known about the protective benefits of cardiovascular and pulmonary fitness and conditioning and about the negative effects of bed rest and restricted mobility (discussed later in this section), little has been documented about prescribing exercise to counter orthostatic intolerance in a given patient.31 The characteristics of deconditioning or detraining in a given individual are determined by age, sex, conditioning status before bed rest, underlying pathophysiology, fluid and electrolyte balance, medications, and the duration of bed rest.32
The preventive effects of an exercise stimulus can be defined as that exercise dose that will maintain the patient’s conditioning level and prevent deterioration. At present, the prescription of preventive mobilization and exercise is nonspecific. Aerobic exercise and resistance muscle training have differential effects in countering the effects of restricted mobility.33,34 Although the upright position is the primary means of countering orthostatic changes associated with recumbency, exercise may have a limited role.35 Even though high-intensity, short-duration, isotonic cycle ergometry training (ITE) and intermittent resistive isokinetic training (IKE) have no effect on orthostatic intolerance associated with recumbency in healthy people, ITE maintains plasma volume, red blood cells, and positive fluid balance, and IKE has no effect on plasma loss but mitigates red blood cell loss.
These differential training effects are of considerable clinical interest; however, they are the result of high-intensity training, and further recumbent exercise should not be considered a substitute for the gravitational stress associated with the upright position. Although patients who are severely ill are not usually capable of performing intense exercise, it is important to note that the responses to upright exercise by healthy people after 5 days of bed rest can be maintained with a daily 30-minute session of intense interval upright exercise training.36 Further, moderately intense exercise performed against lower body negative pressure to simulate an upright 1-g environment fails to counter orthostatic intolerance after 15 days of bed rest.37 Also, aerobic pedaling exercise in the supine position during bed rest may be inadequate to maintain hemodynamic function and .38 With respect to the parameters for prescription of mobilization, the intensity of training appears to be singularly important in attenuating the deconditioning effects associated with bed rest. In terms of importance, intensity is followed by frequency.39
Resistance training fails to counter the cardiovascular deconditioning associated with bed rest.40 Some evidence suggests that isometric exercise during bed rest can attenuate the decrement in and maintain muscle integrity better than isotonic exercise.41 Markers of lactic dehydrogenase, hence anaerobic threshold, increase with both types of exercise. Over time, however, reduced hydrostatic stress contributes to reduced lactic dehydrogenase in both types of training.
What preventive mobilization/exercise is best for which individuals and under what conditions (i.e., what are the principles of prescription)?
How do different types of exercise compare with respect to their preventive effects when all else is constant (i.e., patient’s age and demographic profile, smoking history, general health, presenting illness and severity, body weight, nutritional status, aerobic condition, stress levels, cognitive status, and medication schedule)?
Does exercising in some body positions elicit better preventive effects than exercise in other body positions?
What principles establish the mobilization/exercise intensity, duration, and frequency that are optimal for a given individual whose mobility is restricted?
What types of exercise are best suited for cross-transfer of their effects between the ipsilateral and contralateral limbs, to limit deconditioning when one limb is immobilized?
What are the parameters that should guide physical activity during the day to limit prolonged periods of sitting (i.e., maximal duration of periods of inactivity, degree of physical activity needed to offset the deleterious effects of sedentary periods)?
What constitutes an adequate stimulus to maximize the preventive effects of exercise for a given patient, particularly a patient who is critically ill?
Hazards of Sedentary Activity
Cardiorespiratory conditioning or fitness is related to the volume of regular physical activity; however, its relationship to health measures is less strong.42 Physical inactivity has been causally implicated in lifestyle-related conditions (Chapter 1), but in general, it is not known whether a precise dose of activity or exercise exists that can provide protection against these conditions. One exception to this occurs with ischemic heart disease, in which a threshold intensity of 6 metabolic equivalents (METs) in conjunction with a minimal volume of regular physical activity is recommended for optimal cardiovascular health.43 The precise dose for prevention of hypertension and stroke is less clear.44 Moderate as opposed to heavy exercise has been recommended.43 The choice of relative or absolute intensity of effort depends on the desired preventive outcomes, as well as the patient’s specific characteristics and history.
Although a person may exercise an hour a day, the question arises about the remaining 23 hours. Over the past decade, investigators have focused on the health consequences not only of physical inactivity but of prolonged periods of sedentary behavior as well. Research has shown that in addition to regular physical exercise, breaks during periods of sedentary activity (including screen-based activities) are essential to offset the effects of chronic inactivity.45,46 With the advent of screen-based entertainment, children today are at serious risk for the consequences of hours of sedentary activity; furthermore, sedentary children are more likely to become sedentary adults. Conversely, children who are active and move frequently are more likely to do so as adolescents and adults.47
Chronic inactivity increases the risk for chronic lifestyle-related conditions and promotes premature aging. Alternatively, a physically active lifestyle promotes health and well-being, even offsetting lifestyle-related chronic conditions, as people age.48
Physiological Effects of Bed Rest
Humans are designed to be upright and moving; indeed “upright and moving” can be described as the normal physiological position. The deconditioning associated with restricted activity in patients is comparable to detraining in athletes,32,39 yet is distinct from bed rest deconditioning, which is associated with recumbency as well as restricted mobility.
When people are recumbent and inactive, gravitational stress (the vertical gravitational gradient) and exercise stress are removed. Bed rest, which requires patients to remain in this recumbent and inactive state, is one of the most commonly used yet seldom questioned therapeutic practices. Despite its widespread acceptance, the body positions it promotes are nonphysiological (Chapter 20). The harmful sequelae of this posture have been well documented;24,39,49–56 yet the more severely ill the patient is, the more confined that individual is to the bed—thereby the greater the risk for multisystem complications (Table 18-2).
Table 18-2
Physiological Consequences of Bed Rest
Bed Rest Consequence | Potential Mechanisms |
I.Fluid volume redistribution |
Adapted from Woods SL: Cardiac nursing, ed 5, Philadelphia, 2010, JB Lippincott, Williams & Wilkins.
The classic study by Saltin and colleagues (1968)57 demonstrated marked multisystem deterioration within 3 weeks of bed rest in five healthy young men and the restoration of aerobic capacity with strenuous training afterwards. In a follow-up of the same subjects 30 years later,58 all were found to have an age-related decrement in aerobic power. When their exercise responses were compared over that time frame, it was revealed that bed rest had had a more profound impact on physical work capacity than 30 years of aging.
Of clinical importance is the fact that deterioration of cardiovascular and pulmonary function occurs at a faster rate than musculoskeletal deterioration and that the rate of recovery from the negative effects of bed rest is generally slower than the rate of impairment.59,60 These effects of bed rest are accentuated in older adults61 and are likely to further compound the oxygen transport and other deficits of patients with pathology.
Oxidative stress has been implicated in age-related physiological changes.62 Disuse deconditioning has been implicated as an oxidative stressor contributing to aging, especially when combined with the effects of smoking and a Western-type diet (see Chapter 1). In fact, half of the physical decline associated with aging has been attributed to lack of physical activity.63 With regular physical activity and prescribed aerobic and strengthening programs, these changes can be substantially offset. As a result, functional capacity is maximized and, in the event of illness, the individual has better physiological reserve to deal with the pathophysiological insult. Regardless of age, however, exercise-induced physiological and health advantages are lost following cessation of exercise.64 Restricted mobility in older adults has been also associated with clinical depression.65 Thus the evidence supports the need for sustained physical activity and exercise throughout life to derive their functional and health benefits.
The recumbent, immobile positions associated with bed rest adversely affect most organ systems by means of an apparent down-regulation of the oxygen transport system.66 This is clinically important because in conventional patient management, there is a direct relationship between how sick the patient is and the amount of time she or he is confined to bed. In addition, the musculoskeletal, neurological, gastrointestinal, and genitourinary systems are adversely affected.
Cardiovascular and Pulmonary Effects
A primary effect of mobilization and exercise on the cardiovascular and pulmonary systems is enhanced mucociliary transport and airway clearance.12 Frequent changes in body position augment airway clearance and minimize the pooling and stagnation of bronchial secretions, hence reducing airway obstruction and airflow resistance (Chapter 20). The benefits of frequent body position changes to prevent the accumulation of secretions or resolve them can be augmented when combined with mobilization.
The cardiovascular deconditioning resulting from bed rest includes the loss of fluid-volume and pressure regulating mechanisms, the loss of plasma volume, and diuresis.67–70 In turn, the hematocrit is increased, and the risk for developing deep vein thromboses and thromboemboli is increased. This is exacerbated by increases in blood viscosity, platelet count, platelet stickiness, plasma fibrinogen,71 and stasis of venous blood flow.
Venous thromboembolic disease is preventable with conservative management, including prophylactic anticoagulation in some instances.72 In addition to restricted mobility, other risk factors include being older, undergoing venous catheterization, and hormone replacement therapy. Conditions that are associated with risk include previous thromboembolus, myocardial infarction, heart failure, severe lung disease, cancer, obesity, and paralytic conditions such as stroke. After acute stroke, patients are particularly at risk due to paresis, recumbency, restricted mobility, and circulatory stasis.73 The work of the heart is increased in a patient who is immobile and recumbent, as is the work of breathing. The work of the heart is greater as a result of the increased filling pressures and heart rate associated with chronic recumbency and the increased blood viscosity. The work of breathing is increased because of reduced lung volumes secondary to visceral encroachment on the underside of the diaphragm, increased intrathoracic blood volume, and restricted chest wall motion.
Pulmonary sequelae of recumbency include reduced lung volumes and capacities, particularly functional residual capacity (FRC), residual volumes (RV), and forced expiratory volumes.74–78 A reduction in FRC in the supine position compared with the sitting position has been attributed to both a decrease in thoracic volume and an increase in thoracic blood volume, thereby increasing pulmonary venous engorgement.79 Thus alveolar-arterial oxygen difference and arterial oxygen tension are reduced during bed rest.80–82 Closing volumes are increased, precipitating arterial desaturation in recumbent positions and subsequent complications.
With bed rest, the blood vessels of the muscles and splanchnic circulation dilate. With prolonged bed rest, they may lose their ability to constrict. The ability of these vessels to constrict is essential for preventing the pooling of blood and maintaining circulating blood volume when the patient assumes the upright position. Following bed rest, a patient may feel lightheaded or dizzy and may faint. Individual differences in terms of orthostatic intolerance have been reported and need to be assessed.67,68,83,84 Individuals with greater venous compliance in the legs recruit mechanisms to compensate, such as activation of the renin-angiotensin-aldosterone system, activation of the sympathetic nervous system, and inhibition of the parasympathetic nervous system, so they tolerate orthostatic challenges better.
Musculoskeletal Effects
With only a few days of bed rest, muscle atrophy is initiated, leading to weakness, discoordination, and balance difficulty.57,85 With severe weakness, excessive strain may be placed on ligaments and joints. Muscles and their inert structures are differentially affected. In the knee extensors, for example, tendon stiffness is reduced and their hysteresis characteristics become exaggerated, whereas this does not occur in the plantar flexors.86 Muscle mass and strength may be lost, and muscle and ligament shortening may occur along with joint contractures, skin lesions, and decubitus ulcers.87,88
At the cellular level, disuse atrophy begins within 4 hours of bed rest. Compared with type IIa muscle fibers in vastus lateralis, type I fibers have been reported to be more seriously affected by bed rest, and they respond less favorably to muscle-resistance training.89 Differences in fiber type may explain why muscles in the lower limbs are differentially affected; for example, the plantar flexors are particularly affected.90 Also, sex differences in muscle strength loss with bed rest have been reported.91 Muscle imbalance may result from poor postural alignment.
Skeletal muscle protein synthesis is compromised by bed rest, aging, nutritional deficit, uncontrolled diabetes mellitus, and sepsis.92 Disuse osteoporosis results from reduction of the mechanical stress caused by gravity and from lack of active muscle contraction across joints. Prevention is a primary goal because recovery of mineral loss in bone is prolonged and its loss is largely irreversible.93 Bone mass is restored later than muscle mass and function after a period of restricted mobility, which contributes to fracture risk.94
The limited positioning alternatives in bed may contribute to poor postural alignment, stiffness, and soreness. Bed rest allocates weight bearing to various body parts not adapted for weight bearing. Skin breakdown most commonly occurs over bony prominences, such as the sacrum, trochanters, elbows, scapulae, and heels. Risk factors for pressure ulcers include age, prolonged hospitalization, restricted mobility and general debility, low body weight, low diastolic BP, and surgical intervention.95
Immobile patients are at risk for bone demineralization. An issue of particular importance in older populations, patients with disabilities, postmenopausal women, and patients on steroids is calcium loss. Prevention of disuse osteoporosis is the primary aim because remineralization of bone, even with aggressive exercise, body positioning, electrical stimulation, and possible pharmaceutical agents, is unlikely.93 In patients who are critically ill, cytokines have been implicated in inactivity-related inflammation and muscle injury and atrophy. Activity has been proposed as a countermeasure.96
Renal Effects
Bed rest–induced diuresis increases renal load. Patients with renal insufficiency are particularly seriously affected by this effect, as well as by other sequelae of bed rest.97 As a result, they may exhibit dysrhythmias, muscle wasting, weakness, neuropathy, glucose intolerance, and reduced bone density.
Central Nervous System Effects
With bed rest and recumbency, orthostatic intolerance in combination with resting tachycardia and reduced exercise capacity occur, reflecting diminished reflex activity of the sympathetic nervous system. Baroreceptor-mediated activity is blunted and appears to involve abnormal processing in the central nervous system at the level of the ventrolateral medulla.98 CNS changes include slowed electrical activity of the brain, emotional and behavioral changes, slowed reaction times, sleep disturbances, and impaired psychomotor performance.88,99 Diminished sympathetic activity is the primary factor responsible for orthostatic intolerance along with hypovolemia; the net result is bed rest deconditioning.100
Metabolic Effects
Metabolic changes that occur during periods of bed rest and the relative absence of muscle contraction include glucose intolerance and reduced insulin sensitivity;101 increased calcium excretion due to bone loss; and increased nitrogen excretion secondary to protein loss from atrophying muscle.102,103 In sedentary people, plasma lipids are elevated and skeletal muscle becomes insulin resistant.104 Insulin sensitivity and triglyceride levels are highly responsive to changes in diet, activity level, and thermogenic state. Fit people are highly insulin sensitive, whereas unfit sedentary people tend to be insulin insensitive. Reversal and the normalizing of insulin sensitivity are dependent on increasing muscle contraction.
Alternatives to the Nonspecific Use of Bed Rest
The evidence supports the following:
1. Bed rest as an effective intervention is lacking.
2. The use of bed rest remains nonspecific and excessive.
3. Bed rest has multisystemic negative effects and thus must be prescribed as specifically and judiciously as medication.
4. Methods other than bed rest to manage very ill patients such as those in the intensive care unit (ICU) must be developed.
Kinetic and rotating beds are electromechanical beds that turn the patient through an arc of about 30 degrees to both sides from the supine position over several minutes.109,110 These beds are used for heavy-care patients who are critically ill and are unable or difficult to turn. Even though studies have shown these beds can enhance oxygenation in severely compromised patients such as those with acute respiratory distress syndrome,111,112 they need to be used selectively judiciously.113 Reliance on passive positioning by the bed should not substitute for more active and active-assisted patient positioning. Such interventions constitute the initial stages of mobilization and ambulation.
Even in patients who are seriously acutely ill (e.g., those in the ICU), body positioning and mobilization are essential to offset the deleterious effects of loss of gravitational stimulation from being upright and loss of exercise stimulation from restricted mobility (Chapters 33, 34, 35, and 36).114
Indications for Bed Rest
Despite the universal acceptance of bed rest as a medically therapeutic intervention, indications for its therapeutic use have not been well documented, and its effectiveness continues to be questioned.39 Further, much more is known about the adverse and potentially life-threatening hazards of bed rest than about its benefits. Bed rest should clearly be used as selectively and prescriptively as other medical interventions to ensure that specific benefits are being derived and that the multisystemic negative sequelae are minimized.
Conditions that are associated with edema require minimizing the effect of gravity on the affected limbs. Whether edema can be controlled by localized elevation rather than bed rest must be established. Certain conditions may require some activity restriction, particularly in their acute stages in order to reduce the work of the heart, as in cases of myocardial infarction. However, 50 years ago, restriction to a chair was reported to be more beneficial than restriction to bed in patients with heart disease.115 Despite this finding, many patients continue to recline in beds rather than sit upright in chairs and initiate ambulation. Mobilizing patients and permitting bathroom privileges has also been reported to be less stressful than having to use a bedpan.116
Acute Effects of Mobilization and Exercise
Physiology of Acute Exercise
A comprehensive list of the physiological benefits of acute exercise is presented in Table 18-3. The principal benefits include:
Table 18-3
Acute Physiological Effects of Mobilization and Exercise
Body System | Effects |
Pulmonary | |
Cardiovascular | |
Lymphatic | |
Hematological | |
Neurological | |
Neuromuscular | |
Endocrine | |
Genitourinary | |
Gastrointestinal | |
Integumentary | |
Multisystemic Effects |
After decades of research in exercise science, based in particular on college-aged male students, an increasing number of studies have identified sex differences in exercise responses. Higher peak and muscle strength are found in men compared with women even when adjusted for body weight. These are explained primarily by hormonal differences, specifically estrogen and progesterone in women and testosterone and androgen in men. Differences in autonomic function contribute to the reduced risk by women for heart disease and to their increased longevity.117 Women have greater parasympathetic control of the heart and less sympathetic activity.
Cardiovascular and Pulmonary Effects
Respiratory muscle fitness may affect exercise performance in healthy individuals. Isolated respiratory muscle training increases respiratory muscle endurance and endurance time in response to whole-body exercise.118–120 Although is unaffected,
and blood lactate decrease after training. In addition, respiratory muscle training can attenuate breathlessness in healthy people during exercise. However, improved respiratory muscle strength and endurance with specific training have been reported not to transfer to
in athletes.121 Although optimizing respiratory muscle fitness through whole-body functional exercise is the goal in patient care, these findings have some clinical implications.
In healthy people and those with heart disease, RPP is highly correlated with myocardial and thus with myocardial work122),
, and carbon dioxide production (
). Generally, SV increases disproportionately more than HR at low intensities of exercise to effect CO. With increasing intensity, SV contributes proportionately less to HR, which continues to increase until the maximum HR is achieved with incremental exercise. In moderately active young women, however, SV plateaus through moderate to heavy exercise intensity and then undergoes a secondary increase at very heavy work loads.123 With endurance training, SV is increased, largely reflecting increased diastolic filling and emptying rates and an increase in blood volume. Older people may not increase their maximal CO and SV in response to training, in favor of peripheral adaptation.124 The area of greatest ventilation-to-perfusion matching in the midzones of the lungs, zone 2, is increased secondary to increased dilation and recruitment of pulmonary capillaries.125
The hemodynamic benefits of exercise are maximized in the upright position (as opposed to recumbent positions) because exercise alone fails to counter the loss of volume-regulating mechanisms associated with recumbency.126 Most important is the role of gravitational stress on maintaining BP control and reducing orthostatic intolerance (see Chapter 19 for the effects of position changes on hemodynamics). During exercise, end-diastolic volume and SV have been reported to be greater in the upright position than the supine position in endurance athletes, which supports greater reliance on the Frank-Starling law.127 Thus body position determines the relative contributions of HR, myocardial contractility, and the Frank-Starling mechanism to CO during exercise. Patients with impaired venous return and myocardial contractility may benefit from moderate-intensity recumbent cycling in which central circulation and local vasodilation are favored.128 Plasma volume increases with acute intense exercise, and this has been shown to be position-dependent.129 Plasma albumen content increases in upright compared with supine positions, and this is thought to be responsible for the increase in plasma volume. By coupling upright positioning with exercise, the physical therapist may directly help to normalize fluid balance and hemodynamics in patients whose fluid homeostasis is threatened.
The effect of acute exercise on blood coagulation and platelet aggregability is of particular interest in individuals with existing risk factors for clotting. The risk for stroke, for example, is of clinical importance in people with atrial fibrillation. Moderate levels of activity are associated with minimal procoagulatory effects compared with high-intensity exercise, which increases platelet activity.130 Whether this increased platelet activity constitutes a risk factor clinically has yet to be determined.
The role of exercise in preventing deep vein thrombosis is well established. Although controversy has existed regarding its role in managing deep vein thrombosis, aggressive approaches, including walking based on judicious assessment, have been proposed.131 Recent evidence has shown that leg compression coupled with walking is superior to bed rest in the management of acute deep vein thrombosis in outpatients.132 Further, a systematic review and metaanalysis have corroborated that mobilization does not increase the rate of pulmonary embolism or complications above that found with bed rest.133
Endocrine Effects
Mobilization and exercise stimulate the endocrine system. Catecholamines, released to support the cardiovascular system, sustain a given exercise work rate. Increased sympathetic activity secondary to mobilization can help reduce the patient’s need for sympathomimetic pharmacological agents,134 an important physical therapy outcome. Sympathetic nerve stimulation is increased, resulting in sympathetic neurotransmitters being processed more efficiently (i.e., synthesized and biodegraded). This is a significant effect that can be used as a goal when prescribing mobilization. When exogenous catecholamines are used to augment DO2 (optimally to 600 mL/min/m2) in patients in surgical ICUs, the survival rate is improved and there are no increases in cardiac events over those in control patients.135 Whether this effect, which is probably mediated by improved cardiac reserve, could be achieved by exercise-induced sympathetic stimulation warrants study. An additional benefit of sympathetic arousal in patients who are critically ill may include antiinflammatory effects associated with increased catecholamines.136
Musculoskeletal Effects
Resistance muscle training in patients who are critically ill has been shown to have general and local benefits in terms of acute and long-term effects, as well as its preventive effects. Peripheral and respiratory muscles are important targets of exercise prescription in this patient population, and such training may have implications for avoiding mechanical ventilation or, if mechanical ventilation is indicated, facilitating weaning.137 Resistance exercise is an effective countermeasure to muscle atrophy when a patient is recumbent.33,138
Resistance muscle training has been a mainstay of physical therapy for motor recovery and conditioning effects in patient populations. There has been an increasing interest in the hemodynamic effects of resistance training and their interactions. Notably, after a resistance training program, healthy older adults show improved aerobic exercise responses. Cardiovascular responses to exertion are reduced, peak responses are delayed, and recovery from maximum exertion is faster.139 Training effect is determined by a dose-dependent relationship between intensity of resistance training and aerobic exercise responses, in addition to the individual’s pretraining status.140 These effects are of benefit to patients with primary cardiovascular and pulmonary dysfunction; thus modified resistance training has become an integral component of traditional cardiac and pulmonary rehabilitation programs (see Chapter 24). Resistance muscle training, however, is associated with increased arterial wall stiffness, hence reduced compliance and increased pulse pressure.141 Increased pulse pressure is a risk factor for ischemic heart disease, which suggests the need for caution in prescribing exercise that has a selective effect on pulse pressure.
Caution should be taken with high-resistance exercise, which is defined relative to the individual’s strength and endurance status. Sit-ups, for example, require relatively strong abdominal contraction and can elicit a heavy resistance contraction or strong isometric effort in weak individuals. In turn, intrathoracic pressure increases, SV decreases, and vascular injury may be precipitated. There have been reports of catastrophic neurological consequences (stroke and spinal epidural hematoma) in two healthy young men.142 Thus before abdominal exercise is prescribed, patients should be screened for risk factors. Breathing control should be incorporated to reduce intrathoracic and intraabdominal pressure. The physical therapist needs to be vigilant about detecting neurological signs and symptoms early.
Upper-body and lower-body work have distinct physiological characteristics.143 These responses may have to be avoided (as in the hemodynamic stress of upper-body work in an individual with myocardial dysfunction) or exploited (as in an individual with lower-body paralysis). Oxygen kinetics differ for the two types of work, as do their hemodynamic responses. kinetics are prolonged in arm cranking compared with leg cycling. This change is consistent with increased type II fiber recruitment. Type II fibers are metabolically inefficient compared with fast, glycolytic, type I fibers.
Central Nervous System Effects
CNS responses to mobilization include arousal via activation of the reticular activating system and priming of the various organ systems involved.144 With respect to autonomic function, parasympathetic inhibition occurs at the start of exercise, followed by sympathetic activation to augment the force and rate of myocardial contraction. Substrate utilization and transfer to working tissue, as well as the capacity for oxygen to be supplied to muscle, are precisely regulated through coordinated control of body temperature, breathing, cardiac function, and vasoactivity, both systemically and locally; at the tissue level, they are regulated by the control of local metabolism and the production of vasoactive and chemoactive substances.
Metabolic Effects
The metabolic effects of acute exercise, in particular, on glucose metabolism and growth hormone synthesis are of considerable clinical relevance because these functions are fundamental to health and recovery. Restricted physical activity causes hyperinsulinemia and hyperglycemia and reduces growth hormone synthesis.145 Thus the acute effects of exercise are instrumental in offsetting these changes.
Immunological Effects
Acute exercise has profound effects on the immune system. Even a single bout of moderate exercise has a positive effect on immunity.146 Whether there is a dose-dependent effect is not known. Also unknown is whether there is a cumulative effect of short periods of less intense exercise, such as for the patient who is acutely ill or who has a low functional capacity.
Exercise induces white blood cell production, so it may optimize natural immunity.147 Generally, moderate levels of regular aerobic exercise are associated with improved immune function.148 Within the first 10 minutes of intense exercise, leukocytosis increases, as do the thrombocytes responsible for increasing platelet production. These changes are not associated with exercise-related hypovolemia or hyperthermia. Whether leukocytosis and exercise are dependent on dose or whether there is a critical exercise intensity that has to be achieved to stimulate leukocytosis has yet to be established. Prolonged exhaustive exercise has been associated with compromised immunity, which may be avoided with optimal rest and recovery, along with good nutrition and possibly vitamin C supplements.149 After prolonged heavy exercise in athletes, an “open window” of susceptibility to infection lasting 3 to 72 hours after exercise has been described.150 The risk for infection may be exacerbated by lack of rest and sleep, inappropriate diet, weight loss, and mental strain, and it may be prevented or reversed by better rest and diet and exercise.151 Training strategies recommended for athletes to optimize their immunocompetence have relevance for clinical populations. Exercise sessions are monitored for individuals’ susceptibility to infection, their general health, and the intensity and duration of the exercise session, as well as recovery, rest, optimal diet, and stress management.152,153
Psychological Effects
Acute exercise has also been reported to improve psychological well-being and mood.154 Despite the well-established benefits of exercise on mental health (e.g., reducing anxiety and tension and relieving depression),155 it is not utilized often enough. To take full advantage of these nonpharmaceutical cost-effective benefits, exercise needs to be a more frequent recommendation.
Prescribing Exercise and Mobilization
Once the necessary specific effects of acute mobilization or exercise are identified and matched to the patient’s underlying pathophysiology (Box 18-1), the mobilization or exercise stimulus can be prescribed. Rather than a rigidly structured regimen, the prescription must evolve as the patient progresses in order to maximize its benefits (Table 18-4).
Table 18-4
A Increase the intensity of the mobilization stimulus, the duration of the session, or both, commensurate with the patient’s capacity to maintain optimal oxygen transport when confronted with an increased mobilization stressor, and in the absence of distress; monitored variables should remain within the predetermined threshold range.
B Adjust the frequency based on patient response—in general, shorter treatments and increased frequencies (see Table 18-7).
*All sitting positions require upright erect sitting. Propped up, semirecumbent, slouched, or slumped positions, although approximating the upright position, are not physiologically comparable.
†No amount of movement or activity is too small to derive acute or long-term benefits.
There are two primary components in assessing acute exercise responses:
1. Establishing a stable baseline founded on standardizing the preexercise conditions; and
2. Determining the appropriateness of the quantitative and qualitative changes in the objective and subjective responses during the onset of activity, the steady-state phase of activity, cool-down, and recovery.
Recovery is an important part of the exercise response assessment; postexercise hypotension in a healthy person, for example, is greater and longer after intense exercise.156 After the cessation of intense exercise, CO is initially maintained by increased SV; after low-intensity exercise, it is maintained by HR.
reflects the sum of the conductances of an integrated system of oxygen transport from the inspired air to the mitochondria.
CO is in part passively regulated by the elastic characteristics of the peripheral circulation,157 so its anatomic and physiological integrity is essential to DO2.
The diffusive conductance of muscle is a particularly important component of oxygen transport and has become a focus of research related to impaired exercise capacity in patients with chronic heart, lung, and renal disease.158 This may explain why the correction of central cardiovascular defects in oxygen transport can fail to restore exercise capacity in these patients.
To evaluate an apparently healthy individual who is beginning an exercise program, the Physical Activity Readiness Questionnaire (PAR-Q) is an established screening device (Figure 18-4). The answers help establish whether a progressive exercise program is indicated or whether there are contraindications to the individual’s undertaking a progressive exercise program. The person may require a medical workup if cardiovascular and pulmonary risk factors or musculoskeletal limitations exist. Underlying conditions may have to be addressed beforehand or concurrently.
The prescription of mobilization and exercise to stimulate their acute benefits parallels that of the prescription of exercise for its long-term, central, and peripheral aerobic effects. The exercise parameters for achieving long-term adaptations in healthy people have been defined14 (Table 18-5) and are generally well accepted: the individual engages in aerobic exercise at a heart rate intensity of 40%-85% of heart rate reserve (HRR) for 20 to 40 minutes, 3 to 5 days a week (HRR based on the Karvonen formula: HRrest + 40%-85% [HRpeak/HRmax − HRrest]). The traditional formula of training exercise (HR 70%-85% of age-predicted maximum or tested maximum, for 20 to 40 minutes, 3 to 5 days a week) may have greater utility in people with mild chronic conditions. Aerobic training effects are usually observed within 2 months.
Table 18-5
Exercise Prescription Parameters to Elicit Long-Term Aerobic Benefits in Generally Healthy People
Parameter | Specification |
Exercise type | Rhythmic activity involving the large muscle groups—legs, arms, or both |
Intensity | 40% to 85% of HRR using predicted HRmax or observed HRmax (based on Karvonen formula) |
Duration | 20 to 40 minutes |
Frequency | 3 to 5 days/week |
Course | 6 to 8 weeks |
HRR, Heart rate reserve, which equals (HRmax − HRrest) × desired exercise intensity + HRrest.
Modified from the American College of Sports Medicine: Guidelines for exercise testing and prescription, ed 8, Philadelphia, 2010, Lippincott, Williams & Wilkins.
Early mobilization has been reported not only to reduce the risk for restricted mobility, but also to initiate the rehabilitation process along its continuum toward return to daily function. After an acute stroke, for example, patients undergo threat to oxygen transport in addition to the risks for restricted mobility during the acute period. A recent review of patients during this period reported low activity levels.159
Differences in acute aerobic exercise responses exist between men and women; one example is in those with hypertension.160 The lower in women has been attributed to both central and peripheral factors. The higher SVO2 observed in women contributes to their reduced arteriovenous oxygen difference. These findings can be explained by women’s smaller muscle mass, capillary density, and oxidative capacity. Older women show depressed cardiac function compared with young women; however, this difference is not observed between older and younger men.161 Specifically, the percentage of increase in ejection fraction and the decrease in vascular resistance are depressed. This may be explained by endothelial dysfunction in postmenopausal women, which impairs peripheral vascular function during exercise.
Acute aerobic exercise responses to resistance muscle training have become more interesting clinically because the aerobic responses may have to be used as a guide to the muscle training prescription, particularly in individuals with cardiovascular risk factors and in those who are severely ill. One study examined the metabolic and cardiorespiratory responses of healthy people to maximal intermittent knee isokinetic exercise at 60 degrees/sec and 180 degrees/sec.162 In the last 2 minutes of exercise, ,
, HR, and BP were comparable, consistent with a steady rate. The slope for the increase in
was steeper in the 60 degrees/sec protocol than in the 180 degrees/sec, whereas HR was unaffected by angular velocity. Neither systolic nor diastolic BP was related to exercise intensity or angular velocity of exercise. Although the metabolic and cardiorespiratory responses to intermittent isokinetic exercise of the knee mimic dynamic exercise, qualitative differences likely reflect an isometric component associated with postural stabilization. Thus the cardiorespiratory capacity of patients must be screened to ensure that they can safely meet this demand or, conversely, so that the resistance training program can secondarily augment cardiovascular conditioning.
The acute hemodynamic responses to isolated muscle testing and training are seldom considered when such training is prescribed for clinical populations such as people with low back pain. These patients tend to have weak muscle strength and poor physical conditioning.163 Repetitive McKenzie exercises are commonly used in management; these include forward flexion of the trunk in standing and lying and back extension in standing and lying. The hemodynamic responses to these exercises have been reported to be distinct.164 The hemodynamic stress (i.e., the effect on HR and BP) is greater in horizontal lying positions (trunk flexion greater than extension) than in the standing position (trunk flexion greater than extension). These differences may reflect the degree of postural stabilization associated with each exercise, thereby explaining the isometric muscle contraction and disproportionate changes in hemodynamic responses compared with aerobic exercise. Thus both the aerobic and isometric hemodynamic consequences of such isolated muscle exercises must be considered, regardless of whether the training is intended for managing back pain or heart disease.
Mobilization Testing for Progression
If stable, the patient can be exposed to a mobilization challenge test. The patient is monitored before, during, and after mobilization activities comparable to monitoring for a graded exercise test. Box 18-2 outlines levels of mobilization and devices that can be used (by the physical therapist or the patient) to augment the patient’s performance; these are factors included in the overall prescription and are likely to be revised frequently from one treatment session to the next, or even within a session. Relatively low-intensity activities, such as bed exercises, moving in bed, changing body position, sitting up, dangling over the bed’s side, transferring to a chair, doing chair exercises, and taking short walks with assistance, constitute a sufficient stimulus to elicit the acute effects of exercise, as well as gravitational stimulus. The degree of assistance required to perform the activity should be noted because it demonstrates the patient’s individual effort.
Patients who are acutely ill may benefit from recumbent exercise for mobilization testing and training if upright positioning is not tolerated. Body position affects exercise parameters, as well as resting parameters. End-diastolic and end-systolic left ventricular volumes and SVs are larger in the supine position than in a 70-degree upright tilt, at rest, or during exercise.165 CO was comparable, given an increase in HR in the semirecumbent position. When subjects were tested maximally, the left ventricular ejection fraction was greater in the semirecumbent position than in the supine position. Overall, exercise capacity was greater in the supine and full upright positions than in the semirecumbent position. Thus body position affects exercise responses directly by its effect on hemodynamics and cardiac function. Careful patient monitoring is essential, given that recumbent exercise can be more physiologically stressful than exercise conducted upright.
It is important to note for the purposes of progressing a patient’s mobilization and exercise prescription that differences between sexes exist in response to bed rest. The types of muscle-function changes that occur, for example, differ between men and women. Mechanical efficiency when cycling decreases in both healthy men and women after 20 days of horizontal bed rest,166 but delta efficiency (i.e., change in work output/change in energy expended) in upright exercise compared with supine exercise is greater for men and goes unchanged in women. This is thought to reflect a selective decrement in slow-twitch muscle fibers associated with a decrease in leg muscle mass in men during bed rest.
Monitoring for Progression
The RPP is an index of myocardial during exercise and an indicator of the work of the heart.167 In individuals with ischemic heart disease, the anginal threshold often can be defined by the RPP. After bed rest, healthy individuals have blunted sympathetic responses to isometric exercise in the antigravity muscles;168 thus patients who have had periods of recumbency and restricted activity may show similar abnormal responses to exercise. Subjective responses, including discomfort and pain, fatigue, exertion, and breathlessness, can be recorded as needed. Visual analog scales are commonly used, as are modified Borg scales: 0 (nothing at all) to 10 (maximal exertion).169 Subjective responses are more inherently variable and unreliable, therefore potentially less valid.170 Nonetheless, intrasubject reliability may be acceptable if measures are conducted in a standardized manner and thus can serve as supplementary outcome measures.
Prescription of a Mobilization Stimulus
Patients for whom mobilization is indicated have had variable periods of recumbency that can alter their hemodynamics and autonomic function, as well as their capacity to respond normally to mobilization.171 Hemodynamic responses even to static exercise can be blunted, and this may vary from day to day. Further, such responses can vary from hour to hour in acutely ill patients, and day to day in patients with chronic dysfunction. In patients who are severely and acutely ill, passive rather than active exercise may be the only feasible option. Little research has been done to examine the acute and chronic effects of such exercise, although when passive cycling is compared with active cycling (10% of ) in healthy people,
, HR, exertion, and electromyographic (EMG) activity are predictably lower.172 The shortening of the duration of the preejection period and the length of this period in relation to ejection time, however, is similar. Plasma catecholamines remain at resting levels for both types of exercise, but blood lactates increase in both, with higher levels occurring in active cycling. Thus the inotropic response to exercise probably depends on both skeletal muscle mechanoreceptors and the Frank-Starling effect (preload and shortening in the preejection period).
The optimal therapeutic dose of the acute mobilization stimulus, particularly the initial dose, is based on the patient’s presentation and history; the specific parameters are determined by the goals of the treatment and the acute effects of exercise that are indicated. The intensity of mobilization necessary for many patients will be the intensity that elicits the reactions outlined in Box 18-3.
The Mobilization Session
The scheduling of and preparation for a mobilization session is crucial to the response that can be expected. The conditions outlined in Table 18-6 should be adhered to as closely as possible, and their details should be recorded so that any factor that influences the response to treatment can be identified.
Table 18-6
Preparation Recommendation | Specific Considerations |
The patient should be physically prepared. | The patient should be rested, should not have eaten heavily in the previous couple of hours, should be as attentive and aroused as possible, and should be experiencing minimal pain, discomfort, or other distress. The patient’s clothing should not be restrictive. |
The patient’s medication schedule should be reviewed. | A review of a patient’s medication schedule will ensure that treatments are well timed with respect to analgesia and medications that interfere with an optimal treatment response, such as narcotics. |
Any equipment should be noted and positioned appropriately. | Equipment, monitors, or arterial and intravenous lines (including arterial lines, intravenous lines, and catheters) should be positioned to avoid disconnections or mishaps. Mobilizing a patient in intensive care requires the positioning of the mechanical ventilator and other supports before moving the patient. Portable ventilators facilitate mobilizing and ambulating patients.173 |
Move procedures and techniques should be discussed with the team before implementation. | The physical therapist must prepare assistants before moving a patient, particularly if assistance is required. Despite the number of people assisting, the goal is usually to have the patient perform as much of the mobilization activity as possible (within the margins of safety and maximal therapeutic response). To be beneficial, even small degrees of physical movement can provide sufficient stress to the cardiovascular and pulmonary systems. |
Circadian rhythms refer to physiological changes that occur in response to the fluctuating synthesis and secretion of hormones and other endocrine regulators over time (usually over 24 hours). These have a profound effect on physiological function. Circadian rhythms can be exploited in optimizing functional performance. Younger people may want to exercise later in the day, whereas older people might optimize their activity and exercise in the morning.174 These differences are important to the physical therapist concerned with optimizing performance in patient populations whose response to activity and functional capacities may be dependent on time of day. Growth hormone production is determined by circadian fluctuations, sleep, and exercise.175 Growth hormone is implicated in metabolism and physical performance throughout life. Thus exercise-induced growth hormone is an important effect of exercise that can optimize levels of growth hormone for general health, well-being, and successful aging. Circadian rhythms can be desynchronized during illness and, in particular, during hospitalization. That is why normal day and night cycles should be maintained, even in critical care units, so as to augment patients’ recovery.
The basic components of a mobilization session should approximate the components of an exercise session described by the American College of Sports Medicine for long-term training effects14 (Figure 18-5). Wherever possible, the session should include warm-up, steady-rate, cool-down, and recovery. These components are less distinct when a patient has minimal functional capacity or is being mobilized to remediate acute cardiovascular and pulmonary dysfunction. The rhythmic movement of large muscle groups is still ideal. Prolonged static maneuvers are usually avoided, particularly if the patient is severely ill, because of their disproportionate hemodynamic response. The movements that are usually considered when mobilizing a patient include turning, sitting up, shifting, transferring, standing, and taking some steps. The progression of the levels of mobilization and exercise for medical and surgical patients, based on a consideration of metabolic cost (up to light to moderate activity), is illustrated in Figure 18-6. This schema represents the low end of the incremental staircase of activities based on the MET level shown in Figure 18-7 for a broader range of physical activities (up to very heavy activity).
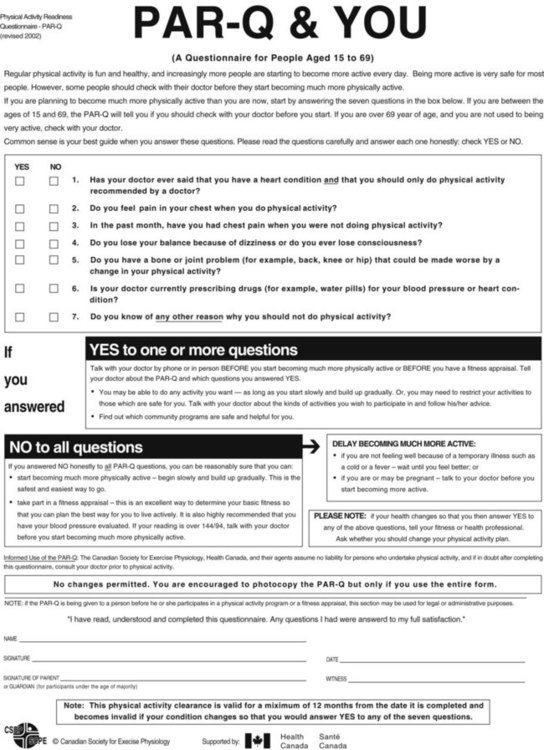
This chapter emphasizes the principles of mobilization and progression for the patient who is acutely ill but medically stable. Patients who are medically unstable, including those in the ICU, warrant special consideration, which is addressed in Chapters 33, 34, 35, and 36. Regardless of the clinical setting, however, the rate at which progression occurs typically depends on a patient’s response rather than a structured protocol.
Monitoring in the Mobilization Session
Monitoring should be performed continuously or at least frequently in patients for whom acute mobilization has been prescribed. First, a baseline of the patient’s resting metabolic state must be established. Second, the metabolic responses to stimuli that perturb oxygen transport and the lability of these responses must be established. Obtaining such a profile provides some indication of the upper limit for the target intensity range of mobilization. The target intensity range can be defined in terms of an upper and a lower level of various physiological variables. The variables to be measured depend on the patient (see Chapters 2 and 17). Most commonly, the values described in Box 18-4 are considered basic measures and indexes of exercise response that require no invasive procedures.
At the other end of the spectrum is the critically ill patient who has various invasive lines and monitoring equipment, including hemodynamic monitoring and intracranial pressure monitoring. This patient requires more specific and frequent measurement of oxygen transport status. Several measures in high-dependency care areas can be monitored continuously, such as cardiac rhythm, HR, and BP, and arterial saturation. The use of transcutaneous oxygen and carbon dioxide tensions as indexes of gas exchange has been considered limited in exercise testing; yet their use would overcome the need for arterial cannulation.176 The use of these measures as estimates of gas exchange has been shown to be reliable, provided the electrodes are heated and the workload changes are gradually increased to allow for latency in response time.
Monitoring during nursing procedures and other types of routine care provides an indication of the patient’s functional capacity without having to conduct a modified exercise test. Detailed monitoring is conducted during a mobilization or exercise challenge. Qualitative assessments can be made based on the physical therapist’s subjective impressions (Box 18-5). The patient’s self-reports are also important.
Adaptation to an Acute Mobilization Stimulus
When an individual is exposed to repeated exercise stress of a specific threshold intensity and for a response-dependent duration and frequency, there is a cumulative adaptive response to that repeated stimulation, or a long-term training response. The goal is to adapt the patient to multiple short mobilization sessions per day, as frequently as once per hour as indicated and as tolerated, and progressively increase the intensity of these sessions, reduce the duration, and correspondingly reduce the frequency of sessions. Table 18-7 illustrates the inverse relationship between exercise duration and frequency of the sessions.
Table 18-7
Incremental Activity | Session Duration | Session Frequency |
Mobilization activities (e.g., self-care, moving in bed, bed exercises, sitting up in bed, sitting on the edge of the bed, standing, transferring to chair, walking) | Short (5 min) | Once/1 to 2 hours |
Long (20 min) | 4 to 6 times/day | |
Interval continuous aerobic exercise | Short (5 min) | Once/1 to 2 hours |
Long (20 min) | 4 to 6 times/day | |
Continuous aerobic exercise (light) | Short (5 min) | Once/1 to 2 hours |
Long (20 min) | 4 to 6 times/day | |
Continuous aerobic exercise (moderate) | Short (10 min) | 2 to 3 times/day |
Long (30 min) | Once/1 to 2 days | |
Continuous aerobic exercise (heavy) | Short (20 min) | Daily |
Long (30 to 45 min) | 3 to 5 days/week |
Mobilization activities prescribed to enhance oxygen transport can be coupled with resistive muscle work to increase muscle strength and endurance (e.g., weights, use of a monkey bar for moving in bed and sitting up, manual resistive exercise, the use of wrist or ankle weights, ergometry, and walking). Coordinating postural changes, thoracic mobility, and upper-extremity exercises with inspiratory and expiratory efforts can be effective in stimulating increased VT and improving ventilation-perfusion matching. Chapters 22 and 23 illustrate how such movement and breathing patterns can be combined in order to maximize oxygen transport in patients with neurological conditions. Such coordinated exercise, however, can also be used in patients with acute cardiovascular and pulmonary conditions. Isometric exercises, postures that require increased muscle work for stabilization, and activities that elicit the Valsalva maneuver produce disproportionate hemodynamic responses, which could be detrimental. The capacity of the patient to respond appropriately to these loads must be assessed beforehand to minimize risk.
In deconditioned individuals and in those whose oxygen transport system has been pathologically compromised, the exercise intensity associated with relatively low-intensity mobilizing activities (such as bed exercises, moving in bed, sitting up, dangling over bedside, transferring to chair, chair exercises, and short walks with assistance) constitute sufficient progressive gravitational and exercise stimuli to initiate adaptation to being upright and moving. However, it is important to stress that optimal adaptation occurs only if these activities are prescribed at the requisite intensity, duration, and frequency, and the exercise prescription progresses commensurate with the individual’s adaptation. Mechanical ventilation is not a contraindication to mobilization; new generations of mechanical ventilators are portable and perform comparably to conventional ventilation. They are associated with minimal adverse effects on hemodynamic variables and oxygen transport173 and can facilitate mobilization and ambulation.
Long-Term Effects of Mobilization and Exercise
In health, the long-term effects of exercise occur in response to a specific and sufficient exercise stimulus to which the individual is exposed for a finite period. With respect to aerobic training or adaptation, the essential training parameters for generally healthy people are shown in Table 18-5. As central and peripheral adaptation to the exercise stimulus occurs, each step in the oxygen transport system becomes more efficient in facilitating DO2, , and extraction at the cellular level.6,15,19 The long-term effects of aerobic exercise are summarized in Table 18-8.
Table 18-8
Long-Term Physiological Effects of Mobilization and Exercise
Body System Affected | Specific Effects |
Pulmonary |
Increased myocardial muscle mass
Increased myocardial efficiency
Decreased stroke volume at rest and submaximal work rates
Decreased resting heart rate and blood pressure
Decreased submaximal heart rate, blood pressure, and rate pressure product
Decreased submaximal perceived exertion and breathlessness
Increased efficiency of thermoregulation
Decreased orthostatic intolerance when performed in the upright position
Cardiovascular and Pulmonary Effects
In addition to an increase in , training results in exercise-induced bradycardia and increased SV at rest and in reduction in the physiological demands of submaximal work rates. Specifically, ventilation and hemodynamic responses to submaximal exercise are decreased. Endurance training increases parasympathetic activity and decreases sympathetic activity at rest.177 Arterial stiffness is decreased,178 and microcirculatory function and tissue perfusion are improved.179 The subjective experience of exercise stress is also reduced. These training effects are commensurate with increases in the efficiency of oxygen transport at each step in the pathway. Unlike the effects in patients with low functional work capacity, however, exercise does not improve ventilatory performance, as is evidenced by the relationship between ventilation and the production of carbon dioxide:180
Because of the marked ventilatory reserve in health, it is normally CO, rather than ventilation, that limits exercise. However, there is some evidence that the respiratory system can limit endurance in healthy sedentary people, in athletes (especially women) with a higher fitness level, and in patients with chronic respiratory disease.181–183
Although change in is the gold standard for aerobic conditioning, theoretically, submaximal
values for given work rates do not change with training184 unless improvement in movement efficiency and economy has occurred. With training, an individual reduces the physiological responses to each exercise load on an incremental exercise test and so is able to achieve a higher work load, thus a higher
with training.
The theory that maximum HR (HRmax) is genetically determined and does not change with training has been challenged.185 Factors that down-regulate HR have been implicated in resetting maximum heart rate. Such factors include the baroreceptors, autonomic function, electrophysiology of the sinoatrial node, and a reduction in the number and density of beta-adrenergic receptors.
The rheology of blood plays an important role in cardiovascular disease. Platelet adhesiveness and aggregation are reduced with regular aerobic exercise.71 Optimal platelet adhesiveness along with blood volume maintain optimal blood viscosity, which is important for blood flow through the heart and blood vessels and for perfusion of peripheral tissue. Increased plasma viscosity and hematocrit have been attributed to exercise-induced hemoconcentration as a result of the shift of fluid from the blood to the interstitium.186 With long-term endurance training, the plasma volume expands, which may reduce the resistance of blood flow to working muscle.
Exercise is a powerful controller of normal BP and an effective intervention to control high BP in patients with hypertension. The antihypertensive effects of long-term aerobic exercise are mediated through a reduction in total peripheral resistance and altered blood rheology. In addition, exercise may lead to weight loss, which contributes to lower BP. Individuals who are normotensive experience no changes in BP with an aerobic exercise program, even though peripheral resistance is reduced.187
Aerobic exercise improves the hemodynamic responses and lipid profiles of individuals with cardiac disease (see Chapter 24). Walking, cycling, and running are common types of aerobic exercise. However, patients from other cultures and those who do not enjoy these types of exercise may prefer tai chi, which also has been shown to have beneficial effects on BP, lipid profiles, and relaxation.188,189
Hemoglobin concentration and the number of circulating red blood cells are essential to effecting optimal oxygen transport (see Chapters 2 and 13). A single bout of exercise, as well as long-term exercise, can influence the erythropoietin processes in bone marrow.190 In the extreme, exercise can interfere with these processes and result in “sports anemia.” This condition can reflect postexercise plasma expansion, increased hemolysis with intense exercise, iron deficiency, bleeding into the digestive and urinary systems, and abnormal erythropoiesis.
Changes in cardiac structure and function after prolonged exercise by healthy people have been of interest to researchers in their attempt to understand changes during ill health. Questions such as “Does the heart muscle show signs of fatigue and impaired function with such exercise?” have been posed. One study of highly trained male athletes showed some cardiac damage, along with reduced diastolic filling and contractility of the left ventricle, after completion of a half-ironman triathlon.191
Central Nervous System Effects
The responses of the CNS to training include autonomic nervous system conditioning that is associated with BP control and thermoregulation.192 Training is associated with a more sensitive vascular response to stress, which may reflect greater alpha-adrenergic sensitivity.193 The capacity to sweat is primed in response to exercise, which ensures that the muscle and internal organs remain at a temperature that is metabolically and energetically optimal for a given workload.
Metabolic Effects
The effect of exercise on glucose kinetics is clinically important for several reasons. First, illness creates metabolic stress on glucose metabolism; second, bed rest reduces insulin sensitivity; third, sedentary lifestyle and high-glycemic diets contribute to hyperglycemia and insulin insensitivity; and fourth, physical therapy’s primary drug, exercise, places stress on energy substrates, including glucose metabolism, commensurate with the type, intensity, and duration of exercise. The time course of changes in glucose metabolism is important to the physical therapist because the patient must be protected from hypoglycemia and the effects of training on the muscles’ sensitivity to insulin must be optimized. Even though these changes are well known to occur with multiple weeks of aerobic training, serial measures over the training period show that after 10 days there are benefits to glucose kinetics.194
Effects in Special Populations
Aggressive mobilization/exercise has been reported to have significant functional impact in the management of patients in the ICU. Prolonged intensive physical training, for example, has been reported to result in the regeneration of skeletal muscle after prolonged catabolism and weight loss.195 Despite studies supporting aggressive mobilization, the physical therapist needs to establish the optimal dose at any given time and to monitor to ensure the best response and the least risk.
Aerobic power declines with age. The capacity to train, however, is not age-limited; training effects have been reported in octogenarians.196 Often older people show greater gains in response to training because of their reduced levels of baseline conditioning. Oxygen kinetics may be slowed with aging. Training responses reflect an increasing contribution to aerobic training by peripheral as opposed to central adaptations.197 Furthermore, based on a 30-year follow-up study, age-related decline in aerobic power over the course of 30 years was reversible with aerobic training.197 Tasks and exercises that involve various physiological systems are differentially affected by aging as well as by training. Training profiles of young and middle-aged adults show an age-related performance decline in cardiorespiratory endurance but not in muscle endurance.198 This finding has implications for norms of fitness and prognosis for recovery. Finally, lack of exercise has been implicated in the incidence of cognitive impairment in older adults.199 Physiological changes associated with aging, particularly those related to exercise capacity, are often confounded by deconditioning and related physical deterioration in older adults rather than being an immutable consequence of aging.200