15 Minimally Invasive EC-IC Bypass Procedures and Introduction of the IMA-MCA Bypass Procedure
Minimally invasive superficial temporal artery to middle cerebral artery bypass through a bur hole
STA-MCA bypasses are established EC-IC bypasses for low to moderate flow cerebral revascularization. A STA-MCA, low-flow, EC-IC bypass traditionally requires a large frontotemporal craniotomy to expose the distal Sylvian fissure for the anastomotic site. We describe a less-invasive procedure using a single 2- to 2.5-cm enlarged bur hole, in place of the standard craniotomy, through which the recipient and donor vessels were identified and the anastomosis performed.1
This procedure was performed for multiple patients with recurrent cerebrovascular ischemic episodes. One patient was awake for the procedure, with local anesthesia and propofol sedation, due to his multivessel occlusive disease and compromised vascular reserve, in an effort to avoid general anesthesia-related hypotension. With the aid of a stereotactic neuronavigation system, we minimized the size of the skin incision and the craniotomy such that the procedure could be performed effectively via an enlarged bur hole or small craniotomy (2- to 2.5-cm diameter). A CT angiogram was used preoperatively to select the donor vessel, recipient vessel, and anastomosis site. The STA was visualized, along with its frontal and parietal branches. The diameters of the branches were measured in order to identify the optimal donor vessel (Figure 15–1A). A linear skin incision was then made overlying the donor vessel. Bur hole/craniotomy placement can be planned preoperatively using a stereotactically reconstructed model based on the CT angiogram (Figure 15–1B). The recipient vessel is chosen according to its caliber and superficial location in the Sylvian fissure. The optimal recipient vessel is identified on review of the CT angiogram (Figure 15–1C). The exact location of the bur hole/craniotomy is then planned using CT angiography–based neuronavigation, and overlies the selected recipient vessel in immediate proximity to the chosen donor vessel.
The incision is performed under the microscope where the temporalis muscle is split vertically directly below the selected donor branch of the STA. A bur hole is made and enlarged to the size of a small craniotomy (∼2 to 2.5 cm) under the microscope, and then 1 cm of the recipient vessel is exposed in the Sylvian fissure (Figures 15–2 and 15–3). A rubber dam is applied and the anastomosis is performed with a 9-0 nylon suture in a running fashion. The back wall is anastomosed before the front wall. Temporary clips are applied on the recipient vessel, the M4 branch of the MCA, during the anastomosis. Postoperatively, the patients were followed up with angiography or CT angiography, and clinically, disease progression was halted (Figures 15-4 through 15-7).
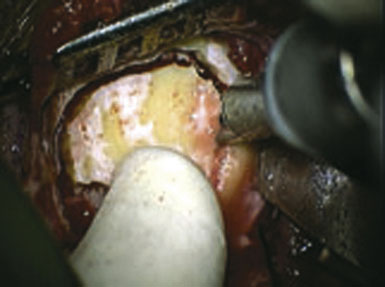
Figure 15–2 Intraoperative photograph obtained through the microscope while the bur hole/craniotomy was being performed.
(From Coppens JR, Cantando JD, Abdulrauf SI, Minimally invasive superficial temporal artery to middle cerebral artery bypass through an enlarged bur hole: the use of computed tomography angiography neuronavigation in surgical planning, J Neurosurg 2008;109(3):553–558, with permission.)
Since the publication of this minimally invasive procedure, Fischer et al.2 have described a similar minimally invasive procedure with the use of 3D virtual planning with the Dextroscope and magnetic resonance angiography in place of intraoperative stereotaxy that could serve as an alternative to the technique described above.
Minimally invasive high-flow bypass technique: internal maxillary artery to middle cerebral artery ec-ic bypass
High-flow cerebral revascularization currently requires graft vessel harvesting, cervical incision for the proximal anastomosis, craniotomy for distal anastomosis, and parent vessel occlusion. This conventionally necessitates a large craniotomy and extensive cervical incision. Instead, we illustrate a technique that avoids a long cervical incision and allows for purely intracranial extradural access to the internal maxillary artery (IMAX) to perform a short segment high-flow anastomosis from it to the MCA, using a radial artery graft.3
In recent years a number of important variations have been introduced to make the high-flow EC-IC bypasses less invasive. The contributions of C. A. F. Tulleken in devising the non-occlusive, laser-assisted anastomosis have to be commended (see Chapter 14). A case report was published using the Cardica C-Port xA Distal Anastomosis System for an automated high-flow bypass. This involves an automated end-to-side anastomosis with simultaneous arteriotomy and insertion of 13 microclips into the graft and recipient vessels. This latter technique however, is very limiting because of the size and configuration of the device and is unlikely to be used widely due to this important limitation.4
In this section we describe our development of the IMAX-MCA bypass technique, which we believe is much less invasive than the standard high-flow EC-IC bypass. Prior to clinical application of our bypass, we performed a series of six adult cadaveric dissections. The IMAX was dissected via a middle fossa extradural approach in the cadaveric specimens in order to confirm the middle fossa surface landmarks, location, and depth of the artery (Figure 15–8). This methodology was subsequently implemented in a clinical case as outlined below.
We drew a straight line extending anteriorly from the V2/V3 apex along the inferior edge of V2. The distance from the V2/V3 apex to the lateral rotundum was 7.8 mm ± 3.033 mm. The distance from the lateral edge of the foramen rotundum to the medial extent of the IMAX was 8.6 mm ± 2.074 mm anteriorly. We then drilled to a depth of 4.2 mm ± 1.304 mm into the greater wing of the sphenoid bone, ultimately exposing an average of 7.8 mm ± 1.643 mm of the maxillary artery (Table 15–1). The IMAX was consistently found running just anterior and parallel to a line between the foramina rotundum and ovale.
Table 15–1 Measurements with mean and standard deviation values obtained from cadaveric IMAX dissections.
The high-flow bypass was conducted via an extradural middle fossa approach for a patient with bilateral transitional (cavernous and clinoidal) internal carotid artery (ICA) aneurysms (Figures 15–9 and 15–10). Prior to the procedure, balloon test occlusion (BTO) was performed in order to assess the quality of the collateral vasculature (Figure 15–11). This showed that the collateral system was insufficient to support cerebral perfusion without an EC-IC bypass. Intraoperatively, the anterolateral triangle between V2 and V3 was identified. Using the aforementioned measurements taken in the laboratory, the anterior loop of the IMAX was found just anterior and parallel to a line running between the foramen rotundum and the foramen ovale. The greater wing of the sphenoid bone was drilled at this location and the anterior loop of the internal maxillary artery was identified.
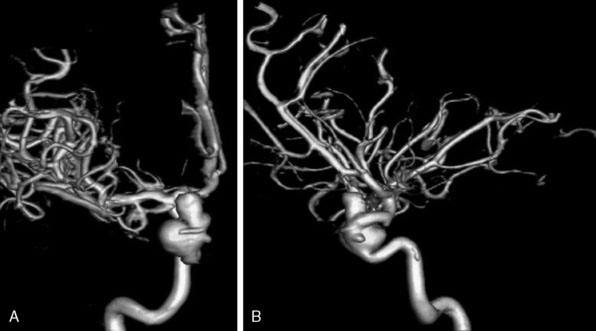
Figure 15–10 DSA three-dimensional reconstruction of a complex transitional segment ICA aneurysm, AP (A) and lateral (B) views.
Simultaneous radial artery harvest was undertaken. Temporary clips were placed on the IMAX (Figure 15–12). An end-to-side anastomosis of the radial artery graft to the IMAX was performed (Figure 15–13), followed by an end-to-side anastomosis to the recipient M2 branch within the Sylvian fissure (Figure 15–14). Intraoperative ICG angiography confirmed a patent graft (Figure 15–15). The ICA was occluded both proximally and just distal to the aneurysm in the supraclinoidal segment with aneurysm clips. The proximal clipping of the ICA, in this particular case, required a very small high cervical incision (2 cm) to avoid clipping the ICA within the cavernous segment, which we hope to avoid in future cases.
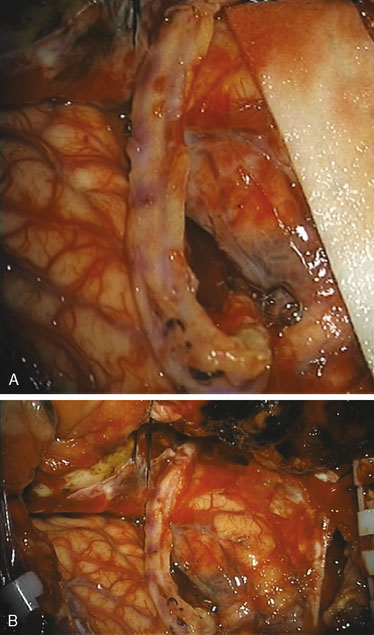
Figure 15–14 A and B, Radial artery interposition graft between the IMAX and the M2 segment of the MCA.
Postoperatively, the patient did well, without any new neurological deficits, and underwent formal angiography ensuring graft patency (Figures 15–16 and 15–17). The patient was discharged home on postoperative day 5; at the 3-week follow-up visit she had resumed normal activity without any restrictions.
1 Coppens J.R., Cantando J.D., Abdulrauf S.I. Minimally invasive superficial temporal artery to middle cerebral artery bypass through an enlarged bur hole: the use of computed tomography angiography neuronavigation in surgical planning. J Neurosurg. 2008;109(3):553-558.
2 Fischer G., Stadie A., Schwandt E., et al. Minimally invasive superficial temporal artery to middle cerebral artery bypass through a minicraniotomy: benefit of three-dimensional virtual reality planning using magnetic resonance angiography. Neurosurg Focus. 2009;26(5):E20.
3 Abdulrauf S.I., Sweeney J.M., Mohan Y.S., et al. Short Segment Internal Maxillary Artery to Middle Cerebral Artery Bypass: A Novel Technique for Extracranial-to-Intracranial Bypass. Saint Louis, MO: Saint Louis University School of Medicine, 2010.
4 Dacey R.G.Jr, Zipfel G.J., Ashley W.W., et al. Automated, compliant, high-flow common carotid to middle cererbal artery bypass. J Neurosurg. 2008;109:559-564.
Abdulrauf S.I. Extracranial-to-intracranial bypass using radial artery grafting for complex skull base tumors: technical note. Skull Base. 2005;15:207-213.
Arbag H., Cicekcibasi A.E., Uysal I.I., et al. Superficial temporal artery graft for bypass of the maxillary to proximal middle cerebral artery using a transantral approach: an anatomical and technical study. Acta Otolaryngol. 2005;125(9):999-1003.
Arbag H., Ustun M.E., Buyukmumcu M., et al. A modified technique to bypass the maxillary artery to supraclinoid internal carotid artery by using a radial artery graft: an anatomical study. J Laryngol Otol. 2005;119(7):519-523.
Baron J.C., Bousser M.G., Rey A., et al. Reversal of focal “misery perfusion syndrome” by extra-intracranial arterial bypass in hemodynamic cerebral ischemia. A case study with 150 positron emission tomography. Stroke. 1981;12:454-459.
Buyukmumcu M., Ustun M.E., Seker M., et al. Maxillary-to-pertrous internal carotid artery bypass: an anatomical feasibility study. Surg Radiol Anat. 2003;25(5–6):368-371.
Charbel F.T., Meglio G., Amin-Hanjani S. Superficial temporal artery-to-middle cerebral artery bypass. Neurosurgery. 2005;56(Suppl 1):186-190.
Drake C.G. Giant intracranial aneurysms: experience with surgical treatment in 174 patients. Clin Neurosurg. 1979;26:12-95.
EC/IC Bypass Study Group. Failure of external carotid–internal carotid arterial bypass to reduce the risk of ischemic stroke. Results of an international randomized trial. The EC/IC Bypass Study Group. N Engl J Med. 1985;313:1191-1200.
Erickson D.L. Revascularization for the Ischemic Brain. Armonk, NY: Futura Publishing, 1988.
Grubb R.L.Jr, Powers W.J., Derdeyn C.P., et al. The Carotid Occlusion Surgery Study. Neurosurg Focus. 2003;14(3):E9.
Hanjani A.S., Butler W.E., Ogilvy C.S., et al. Extracranial-intracranial bypass in the treatment of occlusive cerebrovascular disease and intracranial aneurysms in the United States between 1992 and 2001: a population-based study. J Neurosurg. 2005;103(5):794-804.
Haque R., Kellner C., Soloman R.A. Spontaneous thrombosis of a giant fusiform aneurysm following extracranial-intracranial bypass surgery. J Neurosurg. 2009;110(3):469-474.
Hauck E.F., Samson D. A1-A2 interposition grafting for surgical treatment of a giant “unclippable” A1 segment aneurysm. Surg Neurol. 2009;71(5):600-603.
Karabulut A.K., Ustun M.E., Uysal I.I., et al. Saphenous vein graft for bypass of the maxillary to supraclinoid internal carotid artery: an anatomical short study. Ann Vasc Surg. 2001;15(5):548-552.
Kikuta K.I., Takagi Y., Fushimi Y., et al. “Target bypass”: a method for preoperative targeting of a recipient artery in superficial temporal artery-to-middle cerebral artery anastomoses. Neurosurgery. 2006;59(Suppl 4):ONS320-ONS327.
Lawton M.T., Hamilton M.G., Morcos J.J., et al. Revascularization and aneurysm surgery: current techniques, indications, and outcome. Neurosurgery. 1996;38(1):83-92. discussion 92-4
Lougheed W.M., Marshall B.M., Hunter M., et al. Common carotid to intracranial internal carotid bypass venous graft. Technical note. J Neurosurg. 1982;56(1):205-215.
Meyer F.B., Bates L.M., Goerss S.J., et al. Awake craniotomy for aggressive resection of primary gliomas located in eloquent brain. Mayo Clin Proc. 2001;76:677-687.
Newell D.W. Superficial temporal artery to middle cerebral artery bypass. Skull Base. 2005;15:133-141.
Nussbaum E.S., Erickson D.L. Extracranial-intracranial bypass for ischemic cerebrovascular disease refractory to maximal medical therapy. Neurosurgery. 2000;46:37-43.
O’Shaughnessy B.A., Salehi S.A., Mindea S.A., et al. Selective cerebral revascularization as an adjunct in the treatment of giant anterior circulation aneurysms. Neurosurg Focus. 2003;14(3):e4.
Powers W.J., Martin W.R.W., Herscovitch P., et al. Extracranial-intracranial bypass surgery: hemodynamic and metabolic effects. Neurology. 1984;34:1168-1174.
Sekhar L.N., Duff J.M., Kalavakonda C., et al. Cerebral revascularization using radial artery grafts for the treatment of complex intracranial aneurysms: techniques and outcomes for 17 patients. Neurosurgery. 2001;49(3):646-658. discussion 658-9
Sundt T.M.Jr., Piepgras D.G., Hansen K.K. Saphenous vein bypass grafts for giant aneurysms and intracranial occlusive disease. J Neurosurg. 1986;65(4):439-450.
Tulleken C.A., Verdaasdonk R.M., Berendsen W., et al. Use of excimer laser in high-flow bypass surgery of the brain. J Neurosurg. 1993;78(3):477-480.
Ulku C.H., Ustun M.E., Buyukmumcu M., et al. Radial artery graft for bypass of the maxillary artery to proximal posterior cerbral artery: an anatomical and technical study. Acta Otolaryngol. 2004;124(7):858-862.
Ustun M.E., Buyukmumcu M., Ulku C.H., et al. Radial artery graft for bypass of the maxillary artery to proximal middle cerebral artery: an anatomic and technical study. Neurosurgery. 2004;54(3):667-670. discussion 670-1
Vrionis F., Cano W., Heilman C.B. Microsurgical anatomy of the infratemporal fossa as viewed laterally and superiorly. Neurosurgery. 1996;39(4):777-785. discussion 785-6
Wanebo J.E., Zabramski J.M., Spetzler R.F. Superficial temporal artery-to-middle cerebral artery bypass grafting for cerebral revascularization. Neurosurgery. 2004;55:395-399.
Webster M.W., Makaroun M.S., Steed D.L., et al. Compromised cerebral blood flow reactivity is a predictor of stroke in patients with symptomatic carotid artery occlusive disease. J Vasc Surg. 1995;21:338-345.
Yamauchi H., Fukuyama H., Nagahama Y., et al. Evidence of misery perfusion and risk for recurrent stroke in major cerebral arterial occlusive diseases from PET. J Neurol Neurosurg Psychiatry. 1996;61:18-25.
Yamauchi H., Fukuyama H., Nagahama Y., et al. Significance of increased oxygen extraction fraction in five-year prognosis of major cerebral arterial occlusive diseases. J Nucl Med. 1999;40:1992-1998.
Yaşargil M.G., Krayenbuhl H.A., Jacobson J.H. Microneurosurgical arterial reconstruction. Surgery. 1970;67:221-223.
Yaşargil M.G. Microsurgery Applied to Neurosurgery. Stuttgart: Georg Thieme, 1969.
Yonas H., Smith H.A., Durham S.R., et al. Increased stroke risk predicted by compromised blood flow reactivity. J Neurosurg. 1993;79:483-489.
Zhang YJ, Barrow DL, Cawley CM, et al: Neurosurgical management of intracranial aneurysms previously treated with endovascular therapy, Neurosurgery 52(2):283–293, 200; discussion 293-5