CHAPTER 14 Microfracture
Articular cartilage lesions are a commonly encountered condition seen during arthroscopic surgery (Fig. 14-1)1,2 and may be encountered incidentally in spite of improvements with magnetic resonance imaging (MRI). The limited repair capabilities of articular cartilage chondrocytes and the avascular nature of hyaline cartilage do not allow for any intrinsic healing capacity of full-thickness articular cartilage lesions that do not violate the subchondral bone.3,4 Different techniques have been used in an attempt to create reparative or transfer cartilaginous tissue into the defect with the goal of improved pain relief, activity tolerance, and avoidance of long-term degenerative changes to the lesion or adjacent cartilage.5 Multiple procedures have been used in the past several decades to promote reparative “regenerate” cartilaginous tissue ingrowth into the lesion.6–8
In the mid-1980s, Rodrigo and colleagues9 and Steadman and associates10 adapted the Pridie drilling technique by using an awl to create perforations within the subchondral bone layer. The technique was refined with the knowledge of the importance of the removal of the calcified cartilage layer, overlying the subchondral bone, to improve adherence to the subchondral bone.11
After penetration of the subchondral bone, bleeding from the bone marrow creates a fibrin clot within the defect. Marrow-derived pluripotential cells progressively differentiate into fibroblasts, osteoblasts, chondroblasts, and chondrocytes, without contribution from the adjacent articular cartilage. Shapiro and coworkers12 have demonstrated after cellular labeling via autoradiography that the repopulation is mediated entirely by the proliferation and differentiation of mesenchymal cells from the bone marrow. The collagen matrix formed did not integrate into the adjacent native cartilage and this weak link is theorized to be the potential source of long-term repair tissue degradation. The repair tissue fibrocartilage is also primarily composed of type I collagen, which has decreased resilience and stiffness compared with the type II collagen seen in normal hyaline cartilage.
The risk of articular cartilage defect progression to degenerative arthritis is multifactorial and defect size may play a significant role in this process. A critical size of articular defect that is potentially damaging to adjacent cartilage is not known; the literature shows wide variability in defect size for which treatment had been indicated. Guettler and colleagues13 have evaluated pressure readings from the peripheral rim of articular defects created at various sizes, ranging from 5 to 20 mm. Rim stress concentration was seen in defects of 10 mm and larger, suggesting this as a critical size defect, which may increase the risk of progressive deterioration of the adjacent cartilage.
TREATMENT
Indications and Contraindications
Indications
Microfracture treatment is ideally indicated as a first-line treatment of well-contained Outerbridge grade III or IV cartilage lesions less than 4 cm2. Treatment of larger lesions has been described but most studies included lesions with a mean defect size of less than 4 cm2.14 Unstable chondral flaps that extend to the subchondral bone layer are also lesions amenable to this technique.
Secondary to its technical ease and limited surgical morbidity, microfracture marrow stimulation can be applied to cartilage lesions detected preoperatively by MRI or those found incidentally at the time of arthroscopic evaluation. We have also treated acute traumatic lesions in the polytrauma patient in the appropriate clinical setting (Fig. 14-2).
Patients sustaining acute, traumatic, chondral full-thickness lesions are best treated as soon as is practical logistically because duration of symptoms of less than 12 months has been associated with better postoperative outcome scores and improved microscopic repair cartilage grading.15–17 Patients with chronic or degenerative lesions are initially treated with nonsurgical measures, including activity modification, oral anti-inflammatory medication, physical therapy, dietary modifications, and weight loss, as well as potential articular injections such as corticosteroids or hyaluronic acid. Patients who fail nonoperative treatment are potential candidates for microfracture.
Age younger than 40 years, has been associated with better clinical outcome scores and better repair cartilage fills.18,19 Increased body mass index has been demonstrated to be universally correlated with poor outcomes after microfracture treatment, with worst outcomes seen in those with a body mass index (BMI) more than 30 kg/m2.15
These indications need to be compared with the limited knowledge we have concerning the natural history of nonoperative treatment of articular cartilage lesions and the lack of control groups in trials evaluating operative treatment of these lesions.20 Some studies have demonstrated no difference in outcome for nonoperative management of these lesions compared with operative interventions.21,22 Patient selection also needs to be considered in view of the intensive postoperative rehabilitation program that is usually prescribed following microfracture surgery. Weight-bearing limitations in the postoperative rehabilitation program result in the interruption of normal lifestyle and often induce disuse muscle atrophy.
Contraindications
Axial malalignment of more than 5 degrees for lesions in the tibiofemoral joint is an indication for simultaneous or staged corrective osteotomy (Fig. 14-3). Not only is joint malalignment detrimental to normal articular cartilage, but uncorrected malalignment can result in significantly increased contact pressure in the affected compartment, resulting in increased load and shear on the treated lesions.23
High-grade ligamentous instability will also result in increased shear force across the treated lesion and is a contraindication to microfracture treatment unless surgically treated simultaneously.23
Patient Evaluation Prior to Treatment
The evaluation of the patient for treatment of an articular cartilage lesion requires the assessment of a large number of variables. The history of present illness should include assessment of the mechanism of injury, with documentation of the acuity and cause of the lesion. Patients who have undergone previous nonsurgical and/or surgical treatment need to be questioned about the types of treatments and their response, because these will play a role in future treatment options. Patients previously having undergone surgical treatment should have a careful evaluation of their preoperative imaging, arthroscopic images, and operative reports. Articular cartilage lesions that have undergone previous treatment that violates the subchondral bone layer are unlikely to gain improvement via microfracture.
Physical examination includes documentation of height and weight for determination of BMI. Standing alignment and gait assessment should be included in the examination. The varus thrust in stance phase or varus recurvatum gait would be consistent with a double- and triple-varus knee,24 respectively, and should be considered contraindications to treatment of a medial compartment articular cartilage lesion, unless addressed prior to considering microfracture. Ligamentous integrity of the cruciate and collateral ligaments must be determined, because a deficiency results in abnormal knee translation and resultant excessive shear force on the articular cartilage. Assessment of residual loss of motion or muscular weakness is important because these may play a role in abnormal joint forces and resultant symptomatology. Previous incisions should be evaluated for neuroma formation.
Our radiologic evaluation includes weight-bearing images, including anteroposterior (AP), lateral, Rosenberg (posteroanterior taken at 45-degree flexion), and axial patellar views (Laurin) (Fig. 14-4). A full-length hip to ankle weight-bearing alignment film should also be obtained in the patient undergoing assessment of an articular cartilage lesion. The weight-bearing line (WBL) is measured by drawing a straight line from the center of the femoral head to the center of the tibiotalar joint.25 The mechanical axis is measured by the angle created from a line drawn from the center of the femoral head to the midpoint between the tibial spines in comparison to a line from the center of the tibial spines to the center of the tibiotalar joint. Simultaneous or staged corrective osteotomy should be considered for patients who have greater than 5 degrees of tibiofemoral varus or valgus, based on the involved compartment. Patellofemoral alignment is evaluated via the axial patellar view and trochlear and patellar morphology seen by MRI.
MRI is useful for the preoperative assessment of the size, depth, and location of the articular cartilage lesion.20,26,27 Several acquisition protocols have been recommended by the International Cartilage Repair Society (ICRS).28 Spoiled gradient-recalled echo (SPGR) and fast spin-echo (FSE) techniques are the most accurate and widely used.29,30 New pulse sequences and image acquisition methods are being investigated for improved diagnosis of articular cartilage abnormalities, disease progression, and biologic response to treatment strategies.29–31 The status of the ipsilateral meniscus is important to assess, particularly in a patient having undergone prior surgical treatment and partial meniscectomy. Adjacent and opposing articular surfaces also need to be carefully evaluated. The subchondral and metaphyseal bone are assessed for cyst formation, bone loss, or cavitation. Loss of osseous integrity or sphericity may play a role in impaired fibrocartilaginous ingrowth. The peripheral aspect of the lesion is also evaluated to determine whether there is containment of the lesion, because this is important to the success of microfracture treatment.
Treatment Options
Direct arthroscopic evaluation of articular cartilage lesions is considered the most accurate and reliable means of assessing chondral lesions in regard to size, depth, and location. The lesion size is measured with a calibrated probe. These variables are important in conjunction with preoperative imaging for determining the most appropriate treatment option to address the articular cartilage lesion. Many classification systems exist; the most recent Cartilage ICRS system (Fig. 14-5) uses predébridement and postdébridement measurements of lesion size. However, not included in this or other classification systems is documentation of the condition of the subchondral bone.
Historically, the Outerbridge system (Box 14-1) was the most commonly used classification system for articular cartilage lesions. A limitation of this classification is that the intermediate grades (grades II and III) are only differentiated based on size (more or less than 0.5 inch in diameter) and there is no quantification of the depth of the lesion. Lesions with exposure of subchondral bone are considered to be Outerbridge grade IV.32,33
Arthroscopic Technique
We perform the microfracture procedure with the patient in the supine position using a well-padded pneumatic tourniquet on the patient’s upper thigh, an arthroscopic leg holder, and general or regional anesthesia. A complete arthroscopic examination of the knee is performed using standard medial and lateral anterior portals. The articular surfaces are observed and palpated with an arthroscopic probe. The status and integrity of the menisci are also evaluated and documented. The ligamentous integrity of the knee is verified, particularly the lateral ligaments, using the arthroscopic gap test, with the knee positioned at 0 and 30 degrees of flexion (Fig. 14-6).
The articular defect is assessed for size and location (Fig. 14-7). Associated articular flaps are removed and the margins are débrided back to a peripheral rim with edges perpendicular to the subchondral bone using a curette or arthroscopic knife (Fig. 14-8). Debris is evacuated with the arthroscopic shaver. After débridement, the size of the lesion is again measured with a calibrated probe and recorded. In addition, the arc of motion in which the lesion articulates with the opposing joint surface is recorded. The range of motion of contact will be used for planning the postoperative rehabilitation program (Fig. 14-9).
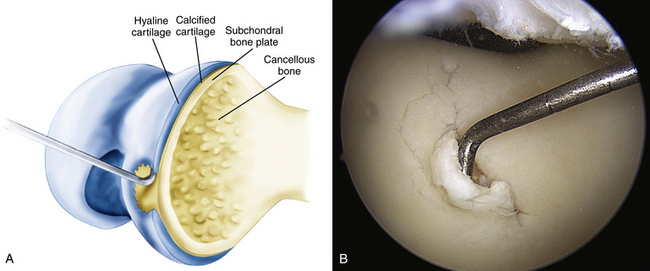
FIGURE 14-7 The lesion is evaluated for size, depth, and margin integrity. A, B.
(Adapted from Mithoefer K, Williams RJ 3rd, Warren RF, et al. The microfracture technique for the treatment of articular cartilage lesions in the knee. A prospective cohort study. J Bone Joint Surg Am. 2005;87:1911-1920.)
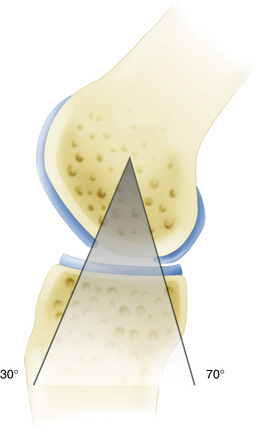
FIGURE 14-9 The lesion is evaluated for range of motion where it articulates with the opposing joint surface. This information is documented and used to guide the postoperative physical therapy program.
(Adapted from Mithoefer K, Williams RJ 3rd, Warren RF, et al. The microfracture technique for the treatment of articular cartilage lesions in the knee. A prospective cohort study. J Bone Joint Surg Am. 2005;87:1911-1920.)
A curette is used to remove the calcified cartilage layer that is just superficial to the subchondral bone layer (Fig. 14-10). Débridement of this calcified cartilage layer has been shown to improve the bonding of the mesenchymal clot to the subchondral bone.23,34 Care is also taken to avoid excessive débridement and subsequent thinning of the subchondral bone; this could stimulate subchondral bone overgrowth, with subsequent relative thinning of the overlying repair cartilage layer and, therefore, subsequent inferior biologic and biomechanical repair tissue quality.15,35 Arthroscopic shaver use is minimized to avoid thinning of the subchondral bone.
Commercially available awls are used to create multiple perforations in the subchondral bone approximately 3 to 4 mm apart (Fig. 14-11).10 The peripheral margin of the lesion is treated first, progressing toward the center of the lesion. Adequate spacing between the perforations is important to minimize the risk of subchondral bone collapse and subsequent cavitation. Perforation of the subchondral bone is performed with the awl tip aligned perpendicular to the subchondral bone layer. The knee is flexed or extended to optimize instrument positioning (Fig. 14-12). Microfracture awls are available in different tip angulations (30, 45, and 90 degrees) with the 30- or 45-degree awl used most commonly (Fig. 14-13). Care is taken to avoid skiving of the awl during impact. Skiving can create a large area of subchondral bone disruption or can break into an adjacent hole. Skiving can be avoided by toeing in the instrument tip and by impaction as close to perpendicular as possible by instrument angulation or knee position.
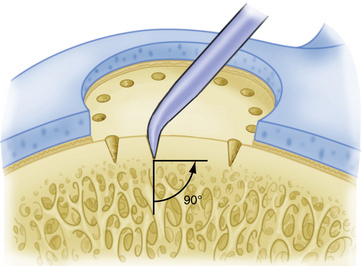
FIGURE 14-11 A 3- to 4-mm-wide bone bridge is maintained between perforations to maintain biomechanical integrity.
(Adapted from Mithoefer K, Williams RJ 3rd, Warren RF, et al. The microfracture technique for the treatment of articular cartilage lesions in the knee. A prospective cohort study. J Bone Joint Surg Am. 2005;87:1911-1920.)
Osseous debris is removed from the microfracture perforations with an arthroscopic shaver. Confirmation of adequate subchondral penetration is accomplished by observation of the release of blood and marrow fat droplets after lowering the arthroscopic intra-articular pump pressure. A drain is not used so as not to remove the mesenchymal clot and hematoma from within the joint (Fig. 14-14). Postoperative intraarticular anesthetic (1% lidocaine) is used without epinephrine. We have also more recently discontinued the use of bupivacaine secondary to reports of chondrotoxicity.36–38 A compression dressing is used as well as cryotherapy for control of postoperative swelling and inflammation.
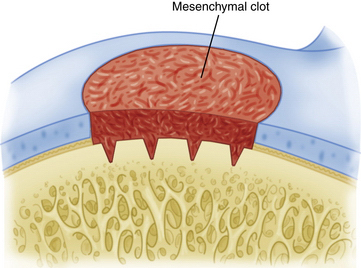
FIGURE 14-14 The arthroscopic pump pressure is lowered and release of marrow fat droplets and blood is verified.
(Adapted from Mithoefer K, Williams RJ 3rd, Warren RF, et al. The microfracture technique for the treatment of articular cartilage lesions in the knee. A prospective cohort study. J Bone Joint Surg Am. 2005;87:1911-1920.)
PEARLS& PITFALLS
PEARLS
POSTOPERATIVE REHABILITATION
Systematic analysis of the literature has demonstrated the variability of rehabilitation protocols after microfracture treatment.14 Limited data exist on rehabilitation protocols after microfracture or the effect of continuous passive range of motion (CPM) and weight-bearing status. Basic science studies have demonstrated beneficial effects of continuous motion on cartilage nutrition, metabolism, and marrow stimulation procedures. In his animal model, Salter9 has demonstrated improved metaplasia of repair tissue within created articular cartilage defects when CPM was used compared with those who did not undergo CPM. Rodrigo and associates9 have also demonstrated an improved visual grading of cartilage repair tissue quality in those patients who used CPM compared with patients who were unable to comply with the postoperative CPM machine (55% vs. 85%). However, Marder and coworkers39 have found no significant difference in outcome with or without the use of CPM or protective weight bearing in lesions smaller than 1 cm2.
Tibiofemoral Lesions.
Our postoperative weight-bearing protocol is predicated on lesion location. Patients with tibiofemoral lesions are advised to maintain foot-flat touchdown weight bearing (approximately 25%) for 6 to 8 weeks. CPM is initiated in the recovery room and its use is encouraged for 6 to 8 hours daily for approximately 6 weeks. The ROM initiated is typically from 0 to 60 degrees and is progressively increased in 10-degree increments until full passive ROM is achieved. The rate is set at one cycle/min but can be altered based on patient comfort. If a CPM unit is not covered by insurance carriers and is cost-prohibitive for patient rental, we instruct the patient to undergo approximately 500 cycles of passive ROM in three sessions daily. This is most easily performed by the patient in a closed-chain fashion doing rolling chair seated flexion (Fig. 14-15).
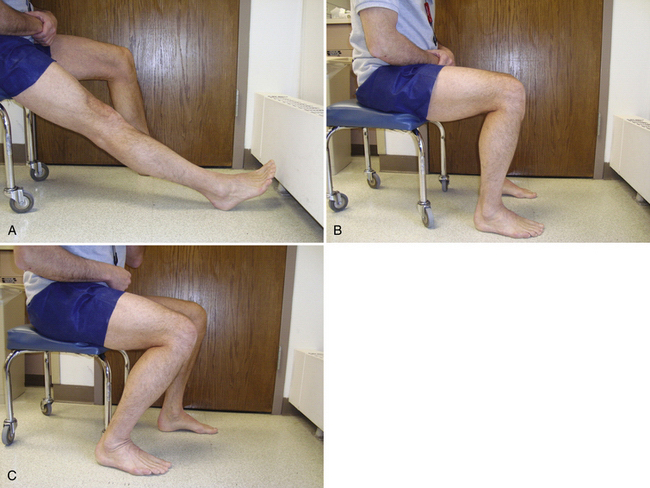
FIGURE 14-15 Rolling chair, closed-chain seated flexion to allow for controlled passive range of motion. A,; B,; C,.
We usually encourage the patient to perform home exercises for the first 1 to 2 weeks after surgery, with an emphasis on rest, elevation, and cryotherapy to lessen the postsurgical effusion.40
Patellar or Trochlear Lesion Microfracture.
The rehabilitation protocol following patellar or trochlear lesion microfracture differs from the above tibiofemoral protocol.10,41,42 The patients are initiated at partial weight bearing in full extension using a knee immobilizer. They are progressed to full weight bearing more rapidly based on their quadriceps reactivation and control. However, ambulation is performed in the knee immobilizer to allow for limited flexion of less than 20 degrees. This is done to eliminate the shear force across the patellofemoral region that would occur with concentric and eccentric quadriceps contraction during ambulation. CPM is also used for patellofemoral lesions, initiated in low ranges of motion (0 to 20 degrees), progressing toward full motion without restriction.
The beneficial effects of joint motion on the healing of articular cartilage lesions are well documented. These include enhanced articular cartilage nutrition and metabolic activity and stimulation of pluripotential mesenchymal cells to differentiate into fibrocartilage. Pressure-dependent matrix flow from surrounding articular surfaces may influence chondrocyte metaplasia.43
Repair Cartilage Fill
Previous experimental studies using an equine model have demonstrated the volume of repaired tissue averaged approximately 64% (Fig. 14-16). A similar finding was seen by Mithoefer and colleagues15 in that most patients demonstrated a persistent depression of the defect; however, all patients with a fill volume of more than two thirds of the defect demonstrated significant improvement in knee function after the microfracture procedure. They also demonstrated persistent gaps between the native and repair cartilage in 92% of patients. This observation was also seen in previous clinical laboratory studies and in alternative articular cartilage repair strategies, including autologous chondrocyte implantation and mosaicplasty.44,45 The failure of peripheral integration is thought to increase the shear stresses on the lesion, potentially leading to micromotion and degeneration.12
COMPLICATIONS
Complications related to the surgical procedure have been rarely reported.9,44–48 Postoperative hemarthrosis or transient swelling is controlled with a postoperative RICE protocol (rest, ice, compression, elevation).8 We try to minimize the use of anti-inflammatory medication in the early postoperative course, if possible. We use active, passive, and pharmacologic adjuncts to minimize postoperative deep venous thrombosis (DVT) risk (e.g., muscle isometrics of the quadriceps, hamstrings, and gastrocnemius-soleus, compression stockings, and aspirin).39, 44,45,47 Supplemental pharmacologic DVT prophylaxis is used on a patient-specific basis.
1. Curl WW, Krome J, Gordon ES, et al. Cartilage injuries: a review of 31,516 knee arthroscopies. Arthroscopy. 1997;13:456-460.
2. Hjelle K, Solheim E, Strand T, et al. Articular cartilage defects in 1,000 knee arthroscopies. Arthroscopy. 2002;18:730-734.
3. Buckwalter JA. Evaluating methods of restoring cartilaginous articular surfaces. Clin Orthop Relat Res. 1999;(367 suppl):S224-238.
4. Buckwalter JA, Mankin HJ. Instructional Course Lectures, The American Academy of Orthopaedic Surgeons—Articular Cartilage. Part II: Degeneration and Osteoarthritis, Repair, Regeneration, and Transplantation. J Bone Joint Surg Am. 1997;79:612-632.
5. Magnussen RA, Dunn WR, Carey JL, Spindler KP. Treatment of focal articular cartilage defects in the knee: a systematic review. Clin Orthop Relat Res. 2008;(466):952-962.
6. Insall JN. The Pridie debridement operation for osteoarthritis of the knee. Clin Orthop Relat Res. 1974;(101):61-67.
7. Magnuson PB. The classic: joint debridementsurgical treatment of degenerative arthritis. Clin Orthop Relat Res. 1974;(101):4-12.
8. Pridie KH. A method of resurfacing osteoarthritic knee joints. J Bone Joint Surg Br. 1959;41:618-619.
9. Rodrigo JJ, Steadman JR, Silliman JJ, Fulstone HA. Improvement of full-thickness chondral defect healing in the human knee after debridement and microfracture using continuous passive motion. Am J Knee Surg. 1994;7:109-116.
10. Steadman JR, Rodkey WG, Rodrigo JJ. Microfracture: surgical technique and rehabilitation to treat chondral defects. Clin Orthop Relat Res. 2001;(391 suppl):S362-S369.
11. Frisbie DD, Morisset S, Ho CP, et al. Effects of calcified cartilage on healing of chondral defects treated with microfracture in horses. Am J Sports Med. 2006;34:1824-1831.
12. Shapiro F, Koide S, Glimcher MJ. Cell origin and differentiation in the repair of full-thickness defects of articular cartilage. J Bone Joint Surg Am. 1993;75:532-553.
13. Guettler JH, Demetropoulos CK, Yang KH, Jurist KA. Osteochondral defects in the human knee: influence of defect size on cartilage rim stress and load redistribution to surrounding cartilage. Am J Sports Med. 2004;32:1451-1458.
14. Mithoefer K, McAdams T, Williams RJ, et al. Clinical efficacy of the microfracture technique for articular cartilage repair in the knee: an evidence-based systematic analysis. Am J Sports Med. 2009;37:2053-2063.
15. Mithoefer K, Williams RJ 3rd, Warren RF, et al. The microfracture technique for the treatment of articular cartilage lesions in the knee. A prospective cohort study. J Bone Joint Surg Am. 2005;87:1911-1920.
16. Mithoefer K, Williams RJ 3rd, Warren RF, et al. Chondral resurfacing of articular cartilage defects in the knee with the microfracture technique. Surgical technique. J Bone Joint Surg Am. 2006;88(suppl 1):294-304.
17. Mithoefer K, Williams RJ 3rd, Warren RF, et al. High-impact athletics after knee articular cartilage repair: a prospective evaluation of the microfracture technique. Am J Sports Med. 2006;34:1413-1418.
18. Kreuz PC, Erggelet C, Steinwachs MR, et al. Is microfracture of chondral defects in the knee associated with different results in patients aged 40 years or younger. Arthroscopy. 2006;22:1180-1186.
19. Kreuz PC, Steinwachs MR, Erggelet C, et al. Results after microfracture of full-thickness chondral defects in different compartments in the knee. Osteoarthritis Cartilage. 2006;14:1119-1125.
20. McCauley TR, Disler DG. Magnetic resonance imaging of articular cartilage of the knee. J Am Acad Orthop Surg. 2001;9:2-8.
21. Messner K, Maletius W. The long-term prognosis for severe damage to weight-bearing cartilage in the knee: a 14-year clinical and radiographic follow-up in 28 young athletes. Acta Orthop Scand. 1996;67:165-168.
22. Shelbourne KD, Jari S, Gray T. Outcome of untreated traumatic articular cartilage defects of the knee: a natural history study. J Bone Joint Surg Am. 2003;85(suppl 2):8-16.
23. Frisbie DD, Oxford JT, Southwood L, et al. Early events in cartilage repair after subchondral bone microfracture. Clin Orthop Relat Res. 2003;(407):215-227.
24. Noyes FR, Barber-Westin SD, Simon R. The role of high tibial osteotomy in the anterior cruciate ligament-deficient knee with varus alignment. In: DeLee JC, Miller MD, editors. Orthopaedic Sports Medicine Principles and Practice. 2nd ed. Philadelphia: WB Saunders; 2003:1900-1936.
25. Dugdale TW, Noyes FR, Styer D. Preoperative planning for high tibial osteotomy. The effect of lateral tibiofemoral separation and tibiofemoral length. Clin Orthop Relat Res. 1992;(274):248-264. Jan 1992
26. Potter HG, Linklater JM, Allen AA, et al. Magnetic resonance imaging of articular cartilage in the knee. An evaluation with use of fast-spin-echo imaging. J Bone Joint Surg Am. 1998;80:1276-1284.
27. Sonin AH, Pensy RA, Mulligan ME, Hatem S. Grading articular cartilage of the knee using fast spin-echo proton density-weighted MR imaging without fat suppression. AJR Am J Roentgenol. 2002;179:1159-1166.
28. Brittberg M, Winalski CS. Evaluation of cartilage injuries and repair. J Bone Joint Surg Am. 2003;85(suppl 2):58-69.
29. Black BR, Chong le R, Potter HG. Cartilage imaging in sports medicine. Sports Med Arthrosc. 2009;17:68-80.
30. Recht MP, Goodwin DW, Winalski CS, White LM. MRI of articular cartilage: revisiting current status and future directions. AJR Am J Roentgenol. 2005;185:899-914.
31. Potter HG, Chong le R. Magnetic resonance imaging assessment of chondral lesions and repair. J Bone Joint Surg Am. 2009;91(suppl 1):126-131.
32. Noyes FR, Stabler CL. A system for grading articular cartilage lesions at arthroscopy. Am J Sports Med. 1989;17:505-513.
33. Outerbridge RE. The etiology of chondromalacia patellae. J Bone Joint Surg Br. 1961;43:752-757.
34. Frisbie DD, Trotter GW, Powers BE, et al. Arthroscopic subchondral bone plate microfracture technique augments healing of large chondral defects in the radial carpal bone and medial femoral condyle of horses. Vet Surg. 1999;28:242-255.
35. Brown WE, Potter HG, Marx RG, et al. Magnetic resonance imaging appearance of cartilage repair in the knee. Clin Orthop Relat Res. 2004;(422):214-223. May 2004
36. Dragoo JL, Korotkova T, Kanwar R, Wood B. The effect of local anesthetics administered via pain pump on chondrocyte viability. Am J Sports Med. 2008;36:1484-1488.
37. Gomoll AH, Kang RW, Williams JM, et al. Chondrolysis after continuous intra-articular bupivacaine infusion: an experimental model investigating chondrotoxicity in the rabbit shoulder. Arthroscopy. 2006;22:813-819.
38. Gomoll AH, Yanke AB, Kang RW, et al. Long-term effects of bupivacaine on cartilage in a rabbit shoulder model. Am J Sports Med. 2009;37:72-77.
39. Marder RA, Hopkins GJr, Timmerman LA. Arthroscopic microfracture of chondral defects of the knee: a comparison of two postoperative treatments. Arthroscopy. 2005;21:152-158.
40. Noyes FR, Berrios-Torres S, Barber-Westin SD, Heckmann TP. Prevention of permanent arthrofibrosis after anterior cruciate ligament reconstruction alone or combined with associated procedures: a prospective study in 443 knees. Knee Surg Sports Traumatol Arthrosc. 2000;8:196-206.
41. Solomon DJ, Williams RJIII, Warren RF. Marrow stimulation and microfracture for the repair of articular cartilage lesions. In: Williams RJIII, editor. Cartilage Repair Strategies. Totowa NJ: Humana; 2007:69-84.
42. Terry MA, Steadman JR, Rodkey WG, Briggs KK. Microfracture for chondral lesions. In: El Attrache NS, Harner CD, Mirzayan R, Sekiya JK. Surgical Techniques in Sports Medicine. Philadelphia: Lippincott Williams & Wilkins; 2007:565-571.
43. Salter RB. The biologic concept of continuous passive motion of synovial joints. The first 18 years of basic research and its clinical application. Clin Orthop Relat Res. 1989;(242):12-25.
44. Knutsen G, Drogset JO, Engebretsen L, et al. A randomized trial comparing autologous chondrocyte implantation with microfracture. Findings at five years. J Bone Joint Surg Am. 2007;89:2105-2112.
45. Knutsen G, Engebretsen L, Ludvigsen TC, et al. Autologous chondrocyte implantation compared with microfracture in the knee. A randomized trial. J Bone Joint Surg Am. 2004;86:455-464.
46. Bae DK, Yoon KH, Song SJ. Cartilage healing after microfracture in osteoarthritic knees. Arthroscopy. 2006;22:367-374.
47. Gobbi A, Nunag P, Malinowski K. Treatment of full thickness chondral lesions of the knee with microfracture in a group of athletes. Knee Surg Sports Traumatol Arthrosc. 2005;13:213-221.
48. Gudas R, Kalesinskas RJ, Kimtys V, et al. A prospective randomized clinical study of mosaic osteochondral autologous transplantation versus microfracture for the treatment of osteochondral defects in the knee joint in young athletes. Arthroscopy. 2005;21:1066-1075.