19 Mechanical Circulatory Support
THE NUMBER OF INFANTS and children hospitalized with heart failure resulting from congenital or acquired heart disease continues to increase each year; according to a recent analysis of 15 million pediatric hospitalizations in the United States, this number grew by 25% between 2003 and 2006.1 Failure of the myocardium, despite maximal medical therapy, to provide sufficient cardiac output for adequate support of end-organ perfusion results in the need for mechanical support of the circulation, either as an adjunct to cardiopulmonary resuscitation (CPR) or as a bridge to myocardial recovery or cardiac transplantation. Data from the Randomized Evaluation of Mechanical Assistance for the Treatment of Congestive Heart Failure Trial (REMATCH) demonstrated prolonged survival and increased quality of life in adult patients with end-stage heart failure who received mechanical cardiac support (MCS).2 Although the number and type of MCS devices available for children remains comparatively limited, particularly for children weighing less than 20 kg in the United States, the use of extracorporeal life support in this population has continued to grow, with a 32% increase in the use of ventricular assist devices (VADs) between 2003 and 2006.3 Initiatives by the National Heart, Lung, and Blood Institute (NHLBI) supporting the development of MCS devices for infants and children offer promising future alternatives for children.4
Initiation of Mechanical Circulatory Support
Indications and Contraindications
Mechanical circulatory support in children is most often necessary due to intrinsic failure of the myocardium. Preoperative cardiopulmonary stabilization may be required in children with profound hypoxemia and/or cardiovascular collapse due to hypercyanotic spells, pulmonary hypertensive crises, obstructed total anomalous pulmonary venous return, occlusion of systemic-pulmonary shunts, or cardiogenic shock (Table 19-1). Extracorporeal membrane oxygenation (ECMO) was used to bridge to surgical repair or palliation in 26 children, with 62% surviving to discharge and no observed differences in outcome between single and biventricular patients.5 ECMO has also been successful in stabilizing children with refractory dysrhythmias.6–8 In a retrospective study, ECMO was used in nine infants with a variety of tachy- or bradydysrhythmias, with all nine surviving to discharge.9 ECMO has also been used to stabilize children in the cardiac catheterization laboratory, both preemptively before high-risk interventional procedures10 and as a rescue technique for catheter-induced complications, persistent low cardiac output, or hypoxemia.11
TABLE 19-1 Indications for Mechanical Circulatory Support
ALCAPA, Anomalous origin of the left coronary artery from the pulmonary artery.
Postcardiotomy myocardial dysfunction can manifest as either early (inability to wean from cardiopulmonary bypass [CPB]) or late failure (sustained postoperative low cardiac output syndrome), with poor end-organ function, persistently increased plasma lactate concentrations, low mixed venous oxygen saturations, and escalating inotropic support. To maximize survival, it is essential to rule out the presence of residual surgical lesions, coronary insufficiency secondary to surgical manipulation, and mechanical problems (e.g., cardiac tamponade) before initiating MCS.12 Both ECMO and left ventricular assist devices (LVADs) have been used for postoperative ventricular support in infants with anomalous origin of the left coronary artery from the pulmonary artery trunk (ALCAPA), either as a bridge to recovery or transplant.13,14 The routine use of MCS after a Stage I Norwood procedure has also been advocated in order to optimize postoperative cardiac output.15
Other cardiac pathophysiologic processes, such as acute myocarditis, coronary ischemia, graft rejection after cardiac transplantation, or end-stage heart failure due to chronic cardiomyopathies, dysrhythmias, or congenital heart defects, may also warrant the use of MCS.16–18 The steadily enlarging population of adults with congenital heart disease who develop heart failure will pose special challenges due to their complex anatomy and prior surgical palliations.
Noncardiac indications for MCS include severe hypothermia, drug toxicity, and near-drowning. ECMO can also provide short-term respiratory support for tracheobronchial reconstruction in infants and children with critical airways, when conventional mechanical ventilation is not feasible or has not been successful.19 Although septicemia was initially considered a contraindication to MCS, a recent review of 45 children who required ECMO for hemodynamic support due to septic shock reported a 47% survival to discharge.20 ECMO can provide a bridge to lung transplantation or retransplantation, and posttransplantation can be used in cases of severe primary graft dysfunction, although survival in these patient groups remains less overall than for other indications.21–23
Timing and Preparation for the Initiation of Support
As experience with the use of pediatric circulatory support continues to grow, it has become increasingly evident that early institution of support better preserves end-organ function and maximizes the opportunity for recovery or bridge to transplantation. The following criteria for implementation of MCS have been used in one institution: (1) cardiac index less than 2 with inotropic dependence; (2) poor peripheral perfusion with metabolic acidosis and mixed venous oxygen saturation less than 40%; (3) signs of impending respiratory, renal, or hepatic failure; and (4) increased or rapidly increasing B-type natriuretic peptide (BNP) concentrations (Table 19-2).24
TABLE 19-2 Suggested Clinical Criteria for Mechanical Cardiac Support Implementation
CI, Cardiac index.
Modified from Hetzer R, Potapov EV, Alexi-Meskishvili V, et al. Single center experience with treatment of cardiogenic shock in children by pediatric ventricular assist devices. J Thorac Cardiovasc Surg 2011;141:616-23; and Potapov EV, Stiller B, Hetzer R. Ventricular assist devices in children: current achievements and future perspectives. Pediatr Transplant 2007;11:241-55.
During routine preparation for MCS, baseline laboratory hematologic studies should be obtained, including a complete blood cell count (CBC), platelet count, prothrombin time (PT), activated partial thromboplastin time (aPTT), activated clotting time (ACT), plasma hemoglobin, antithrombin III, fibrinogen, and thromboelastogram (TEG). A minimum of two units of packed red blood cells (PRBCs) should be available (preferably cytomegalovirus-negative, leukocyte-reduced, and irradiated), because all such children should be considered potential cardiac transplant candidates (note that the potassium concentration of irradiated PRBCs may be greatly increased, resulting in acute hyperkalemia if administered rapidly) (see also Chapter 10). In infants, ultrasonography of the head is useful before considering institution of MCS. Anesthesiologists are frequently involved during the cannulation procedure, and all arterial and central venous lines, as well as the tracheal tube, should be well secured and the ventilator easily accessible.
The emergent use of ECMO for children during in-hospital cardiac arrest with failure of conventional resuscitation methods has become increasingly common. An analysis of data from the National Registry of CardioPulmonary Resuscitation (NRCPR) database of outcomes from children less than 18 years of age who received extracorporeal cardiopulmonary resuscitation (ECPR) for cardiac arrest that was refractory to conventional CPR demonstrated a 43.7% survival to discharge. Preexisting conditions of septicemia, pneumonia, and renal insufficiency correlated with an increased risk of mortality. Children with a cardiac disease diagnosis showed slightly improved odds of survival to discharge when compared with children without cardiac disease.25 In a review of pediatric cardiac patients from the Extracorporeal Life Support Organization (ELSO) registry who received ECPR, single ventricle physiology and a history of more complex cardiac surgery were negative predictors of survival.26 Renal dysfunction, pulmonary hemorrhage, neurologic injury, and the need for additional CPR during ECMO have also been associated with increased mortality.27
Acceptable neurologic outcomes have been described in children after CPR of up to 3 hours in duration before institution of ECMO.28 The duration of CPR before ECMO cannulation did not differ between survivors and nonsurvivors.29 Of the children in the ELSO registry who received ECPR, 22% of the 682 developed an acute neurologic injury, with an in-hospital mortality rate of 89%. The risk of neurologic injury in children with cardiac disease in this cohort was reduced.30
A dry circuit can be kept ready for rapid deployment, with crystalloid prime used during initiation of support and addition of blood products (PRBCs and fresh frozen plasma) as soon as they become available. During resuscitative efforts before the institution of mechanical support, multiple doses of vasoconstrictors should be avoided, if possible, acidosis should be corrected, and ice may be placed around the head to provide cerebral protection. Ultimately, the restoration of cardiac output, even with a low hematocrit, is the most important factor for successful resuscitation and long-term survival.31,32
Mechanical Circulatory Support Devices
In pediatric practice, the size of the child and the type of support required (cardiopulmonary vs. cardiac) are the most important considerations when choosing an MCS device (Table 19-3). Secondary factors are the indication for, and expected duration of, support and the desired end point: bridge to bridge, procedure (surgery or catheterization), recovery, or transplantation. Certain devices, such as the intra-aortic balloon pump (IABP), ECMO, and centrifugal pump, are best used for short-term support (less than 2 to 4 weeks) and may occasionally serve as a “bridge to bridge” for institution of a long-term mechanical support device.33
TABLE 19-3 Mechanical Circulatory Support Devices: Advantages and Disadvantages
Device | Advantages | Disadvantages |
---|---|---|
ECMO |
BSA, Body surface area; ECMO, extracorporeal membrane oxygenation; VAD, ventricular assist device.
From Diaz LK, Andropoulos DB. New developments in pediatric cardiac anesthesia. Anesthesiol Clin North Am 2005;23:655-76.
A VAD may be defined most simply as a mechanical pump attached between the heart and either the aorta or pulmonary artery to circulate blood when one or both ventricles are no longer capable of adequately maintaining circulation. In short, a VAD is a pump supporting a failing ventricle, whether right, left, or biventricular (BiVAD). Isolated ventricular dysfunction with adequate oxygenation, as seen in acute myocarditis, acute rejection after cardiac transplantation, or dilated cardiomyopathy, is the ideal cardiac pathology for VAD support. ECMO, on the other hand, provides full cardiopulmonary support and may be preferable to VAD support for children with pulmonary hypertension, complex congenital heart lesions involving intracardiac shunts, severe hypoxemia, or respiratory failure (Fig. 19-1).
Short-Term Support
Intra-Aortic Balloon Pump
Anatomic contraindications to the use of an IABP include patent ductus arteriosus, aortic insufficiency, aortic aneurysm, and recent aortic surgery. Technical limitations in children consist of size constraints, the increased distensibility and compliance of the aorta, and the difficulty of synchronizing pump function with an infant or child’s rapid heart rate,34 although use of M-mode echocardiography-timed IABP in infants and children to assist with cardiac synchronization has been described.35 IABP use in an adult patient with Fontan physiology and severe ventricular failure has been reported, with improvement in end-organ function and successful weaning.36 IABP support must be initiated early, while ventricular function is still capable of sustaining adequate cardiac output,37 because the balloon pump only augments, and does not replace, ventricular output. It can only support the left ventricle.
Extracorporeal Membrane Oxygenation
Whereas adults generally have isolated left ventricular failure, children more often require cardiopulmonary support due to hypoxemia, pulmonary hypertension, or concurrent right ventricular failure. For infants and children who require short-term or urgent cardiopulmonary support, ECMO remains the modality of choice. Initially reported for the treatment of cardiac failure in children in the 1970s,38 ECMO was subsequently used for mechanical support during interhospital transport.39 Since the ELSO registry began in 1989, ECMO has been the MCS modality with the most pediatric usage, with more than 7500 neonates and children using it for cardiac indications.40
A typical ECMO circuit is composed of a pump (either a roller pump with a servoregulatory mechanism for controlling circuit flow or a centrifugal pump); a hollow fiber or membrane oxygenator; a heat exchanger; and cannulas (either venoarterial or venovenous). A modified ECMO circuit composed of a heparin-coated circuit, Bio-Medicus centrifugal pump (Medtronic, Minneapolis), hollow fiber membrane oxygenator, flow probe, and hematocrit/oxygen saturation monitor, allowing the circuit to be set up and primed in 5 minutes for rapid resuscitation, has been described.41 Most hospitals supporting such a service have readily available trained personnel to assist with implementing and maintaining ECMO therapy. Versatility is one of the advantages of ECMO; venoarterial cannulation in postcardiotomy patients may be either transthoracic via the right atrial appendage and aorta, transcervical via the right internal jugular vein and common carotid artery, or femoral via the femoral artery and vein in larger patients. Heparin-bonded circuitry is often used to minimize surface-induced complement activation, platelet dysfunction, and anticoagulation requirements.42
Other advantages of ECMO include the lack of patient size limitations, the ability to institute support either in the pediatric intensive care unit or the operating room without the need for CPB, the ability to provide ultrafiltration or hemodialysis for children during mechanical support, and the ability to provide biventricular cardiopulmonary support even in very small children. In a review of 27 children who underwent venoarterial ECMO for cardiac indications, both nonsurgical and postcardiotomy, the overall survival rate was 59%; of these, 56% were undergoing CPR at the time ECMO support was instituted. Of the latter group, 73% survived.43 Hemodynamic benefits of ECMO include decreased right ventricular preload and pulmonary artery pressures. Due to reentry of blood into the aorta an increase in afterload often occurs and may require pharmacologic afterload reduction therapy, such as milrinone, nitroprusside, hydralazine, or phenoxybenzamine.
Several management options exist for children with single-ventricle physiology and shunt-dependent pulmonary circulation who require ECMO support. The survival rate of 10 children who underwent single-ventricle palliation and subsequently required ECMO support was greater in those in whom the aortopulmonary shunt was left open during ECMO.44 Adequate alveolar ventilation must be provided, however, and greater ECMO flow rates are generally required to maintain adequate pulmonary and systemic circulations. In children with low pulmonary vascular resistance, pulmonary blood flow may prove to be excessive and limitation of shunt flow with surgical clips may become necessary. Children with single-ventricle physiology have comparable survival rates after ECMO support compared with other cardiac patients.43 ECMO has been particularly successful in treating children with single-ventricle physiology who develop acute shunt thrombosis or transient depression of ventricular function.45 Of 44 children with shunted single-ventricle physiology who required ECMO support, the indication for support was the strongest predictor of survival to discharge, with 81% of those cannulated due to hypoxemia surviving, but only 29% of those cannulated for hypotension surviving to discharge.46 Patients with Fontan physiology who require ECMO have a significantly greater mortality rate (65%), possibly the result of longstanding ventricular dysfunction that is not easily reversible.47
Disadvantages of ECMO include complex circuitry, the need for greater levels of systemic anticoagulation than required by VADs, the necessity for both blood prime and frequent transfusions, and decreased pulmonary blood flow. Compared with other support modalities, ECMO circuitry is complex and requires full-time supervision by trained personnel. Left atrial decompression may occasionally be inadequate, requiring either the placement of a left atrial vent or an atrial septostomy. Inadequate unloading of the left atrium can lead to mitral regurgitation and pulmonary edema or hemorrhage, and can also minimize the chances of myocardial recovery when the left ventricle is not sufficiently unloaded. Moderate levels of ventilatory support must be maintained to ensure that well-oxygenated blood is provided to the coronary arteries, and the child must remain intubated and sedated throughout the period of ECMO support.48
Although effective for rapid rescue and short-term support, ECMO support is generally maintained only for 1 to 3 weeks before the increasing risk of significant complications limits its usefulness.49 In children requiring postcardiotomy ECMO support, the need for prolonged support, renal failure, and low pH in the first 24 hours of support have been associated with a higher mortality.50 A review of combined data between 2004 and 2009 from the ELSO registry and the Organ Procurement Transplant Network database showed that over half the children bridged with ECMO to heart transplant failed to survive to hospital discharge, illustrating that ECMO is not able to reliably provide intermediate to long-term mechanical support to bridge children safely to transplantation.51 Survivors of ECMO support also have greater rates of neurologic impairment than those supported with VADs, with poorer outcomes noted in younger children with more complex disease.52
Centrifugal Pumps
Currently used centrifugal pumps include the Bio-Medicus Bio-Pump (Medtronic, Minneapolis), CentriMag (Levitronix GmbH, Zurich), RotaFlow (Maquet Cardiovascular, Wayne, N.J.), Capiox (Terumo Cardiovascular Systems Corporation, Ann Arbor, Mich.) and TandemHeart (CardiacAssist, Inc., Pittsburgh). The CentriMag pump is available as an investigational device only in the US. The term centrifugal pump is not always synonymous with VAD, because centrifugal pumps may also be used with an oxygenator to construct an ECMO circuit. Without an oxygenator, they may be used for right, left, or biventricular support (except in infants, when size limitations can preclude the presence of two pumps53) and offer the advantage of excellent ventricular unloading and decreased wall stress, optimizing the chances of myocardial remodeling and recovery. Unloading the left ventricle can also decrease left ventricular cavity size and improve septal configuration, resulting in improved tricuspid valve function and right ventricular inflow.54 When used as a VAD without an oxygenator and heat exchanger, reduced systemic anticoagulation is required, compared with ECMO usage.
Centrifugal pumps offer the advantage of decreased trauma to red blood cells and a less pronounced systemic inflammatory response compared with roller pumps.55 A centrifugal VAD spins, creating a vortex, with negative pressure at the inlet drawing blood into the cone and positive pressure at the outlet allowing nonpulsatile ejection at the base. The RotaFlow pump (Fig. 19-2) has a rotating mechanism that is levitated in three magnetic fields with one point bearing, allowing laminar flow and reducing mechanical friction, heat production and clotting potential compared to the Bio-Medicus pump.56 Cardiac output from a centrifugal pump depends on preload, afterload, and the rotational speed of the pump. Because increases or decreases in preload and afterload can affect pump flow without changes in rotational speed, a flow probe is necessary. Excessive negative inlet pressures (hypovolemia) must be avoided because air can be entrained into the circuit.
The TandemHeart system is a low-prime centrifugal pump capable of flows of 0 to 5 L/min, with a hydrodynamic fluid bearing supporting the spinning rotor (Fig. 19-3). Although size requirements (greater than 40 kg) preclude its use in most children, it is advantageous in that it can be placed percutaneously through the femoral vessels, with a transseptal extended flow cannula allowing entry from the femoral vein into the left atrium. The arterial cannula may be placed into the femoral artery, or in patients less than 80 kg, a vascular graft to the femoral artery may be cannulated to avoid lower extremity vascular compromise.57 A pediatric TandemHeart pump with a priming volume of 4 mL for children from 2 to 40 kg is currently undergoing in vitro testing.58
Compared with ECMO, centrifugal pumps are less expensive and can be set up more rapidly, they have reduced priming volumes, and reduced anticoagulation requirements. Disadvantages of centrifugal pumps include the potential for circuit thrombus formation, nonpulsatile flow, and limited duration of usage (usually less than 3 weeks). Excellent outcomes with centrifugal VAD support have been reported in children who required postcardiotomy ventricular MCS of limited duration, such as those with ALCAPA, or ventricular failure due to cardiomyopathy.59 In addition, fewer post-support neurologic complications were noted in children who received VAD support compared with those who received ECMO for similar indications.60
Intermediate- to Long-Term Support
Pulsatile Pumps
Pulsatile pumps are VADs that facilitate chronic support of the circulation while also allowing tracheal extubation, the use of enteral nutrition, and ambulation for the child. They are paracorporeal and either pneumatically or electromechanically driven. Like centrifugal pumps, pulsatile VADs enjoy several advantages over ECMO: they are simpler in design, less expensive, require lower levels of anticoagulation, and may be used for left, right, or biventricular support of the circulation. Unlike the previously discussed devices, pulsatile pumps are suitable for intermediate- to long-term mechanical circulatory support, but until the advent of the Berlin Heart Excor, their use in infants and children was severely limited by patient size constraints. Internationally, the Berlin Heart Excor and the Medos HIA-VAD pulsatile systems (Berlin Heart GmbH, Berlin, and Medos Medizintechnik AG, Stolberg, Germany, respectively) have been successfully used in children of all ages.61,62 Insertion of a pulsatile VAD requires the use of CPB and closure of any existing septal defects.
Thoratec
The Thoratec pulsatile ventricular assist system (Thoratec Corporation, Pleasanton, Calif.) is a pneumatically driven pump with a 65-mL blood sac, Björk-Shiley tilting disc valves, and exteriorized inflow and outflow cannulas (Fig. 19-4). Inflow may be from either the left atrium or left ventricular apex, with outflow to the aorta. Pump output, depending on the cannula size and length, may vary from 5 to 7 L/min.63 The Thoratec pulsatile VAD may be operated in three modes: synchronized to the child’s underlying heart rate, asynchronous, with a set rate programmed into the device, or fill-to-empty, in which the device fills to a set volume before ejection. Although use of the Thoratec has been described in children as small as 17 kg,64 the risk of thromboembolic events is greater in smaller children, because lower blood flow in the relatively oversized device can lead to stasis and thrombus formation.65 Children with congenital heart disease and/or left atrial cannulation are at greater risk for neurologic complications during Thoratec support.66 The use of a fixed-rate mode, with an increased stroke rate of 80 to 90 per minute and partial stroke volumes, is currently recommended because it is associated with fewer cerebrovascular events. Anticoagulation for the Thoratec VAD initially consists of a heparin infusion, whereas long-term protocols generally use warfarin (Coumadin) and aspirin, with maintenance of an international normalized ratio (INR) of 2.5 to 3.5.67
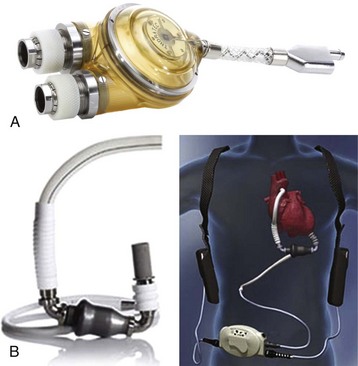
FIGURE 19-4 A, Thoratec pulsatile ventricular assist device. B, Thoratec HeartMate II axial pump.
(Courtesy Thoratec Corporation, Pleasanton, Calif.)
The worldwide experience with the Thoratec paracorporeal VAD in 209 children younger than 18 years of age, ranging from 17 to 118 kg and with a body surface area (BSA) of 0.7 to 2.3 m2, has been summarized. Overall survival to discharge was 68%, but subgroups with cardiomyopathies and myocarditis had greater survival rates than did children with congenital heart disease. Device configuration and the type of support used (LVAD vs. BiVAD) were not correlated with negative patient outcomes.68
Berlin Heart Excor
First used in adults in 1987,69 the Berlin Heart Excor is a pulsatile, paracorporeal pump currently manufactured in three pediatric sizes (10, 25, and 30 mL) and three adult sizes (50, 60, and 80 mL) (Fig. 19-5, A). The pediatric version was first used in 199270 and has since been successfully employed in neonates and infants with a BSA as low as 0.2 m2.61 An investigational device exemption trial was begun in the United States in 2007; before this, the Berlin Heart was used nearly 100 times in North America at 29 different institutions, under compassionate use regulations. A recently published review of 73 of these initial patients (weight 3 to 87.6 kg) revealed a 77% success rate in bridging to either transplant or recovery, with a median support time of 1.6 months. Younger age and the need for BiVAD support were risk factors for increased mortality.71
The Berlin Heart Excor design has not changed since 2001, and consists of a pneumatically driven translucent polyurethane pump, trileaflet polyurethane inlet and outlet valves, and silicone inflow and outflow cannulas. All blood-contacting surfaces, including the polyurethane valves, are heparin-coated (Carmeda AB, Upplands Väsby, Sweden). A flexible diaphragm in three layers divides the pump chamber into an air chamber and a blood chamber (Fig. 19-5, B), with the two diaphragm layers facing the air chamber serving as driving membranes and the third seamless blood membrane passively moved by the driving membranes.72 The Berlin Heart Excor has been successfully used to provide univentricular (left or right) or biventricular support, even in infants, and may be operated in a synchronous, asynchronous, or fill-to-empty mode. A rechargeable battery is available that can provide up to 5 hours of independent power supply for adult-sized pumps, but power requirements are greater for pediatric pump operation, owing to the greater flow resistance with small-diameter cannulas and greater pump rates.73
Although initial outcomes were not as favorable in infants and children with congenital heart disease,74 survival rates of 70% have now been reported for children younger than 1 year of age, even those requiring prolonged support. Improvements in survival have been attributed to the development of optimized miniaturized apical cannulas, the addition of heparin-coated tubing allowing lower anticoagulation levels, the use of dipyridamole and aspirin after week 1 of support, and continued experience with surgical and intensive care management with use of TEGs and platelet function tests to guide the modification of anticoagulation.61 In a single-center review of their Berlin Heart Excor experience, comparing the 1990-to-2001 experience with the current era (from 2002 onward), the percent of infants less than one year of age discharged to home after transplantation or weaning increased from 17% in the early era to 93% in the current era. The majority of children successfully weaned from support had a diagnosis of myocarditis, with no long-term complications after removal of the left ventricular cannula reported. Major causes of mortality in both eras were cerebrovascular accidents and multiorgan failure.24
Major benefits of Berlin Heart Excor support include the ability to extubate the trachea of children, encourage enteral nutrition, and optimize patient mobility during chronic support. In addition, the transfusion of blood products during MCS is less in children supported with the Berlin Heart Excor when compared with those supported with ECMO. In a study comparing 30 children receiving Berlin Heart Excor support to 34 children on ECMO support, transfusion requirements for platelets, red blood cells, and fresh frozen plasma were significantly less in Berlin Heart Excor patients. The overall mortality rate was also noted to be lower in Berlin Heart Excor patients.75 Anticoagulation is currently initiated with unfractionated heparin, maintaining aPTT at 60 to 80 seconds. TEG is also used, along with platelet aggregation tests, to monitor the use of aspirin and dipyridamole. Antithrombin III concentrations are closely monitored and substituted if the concentrations fall below 70%.72 Low-molecular-weight heparin (LMWH), with monitoring of anti–factor Xa concentrations, has been used since 2007.24 Pump exchange may be necessary if thrombus formation occurs in the valves, although one group reported no complications from this procedure during 15 years of adult and pediatric experience.76
Medos System
The Medos HIA-VAD was first used for children in 1997.77 The Medos HIA-VAD is a pulsatile, paracorporeal pump available in several different sizes; it differs from Excor in that the left ventricular pumps offer 10% greater stroke volumes than the corresponding right ventricular pumps.78 Survival has been reported to be 57% in children (ranging in age from less than 1 year to 16 years) awaiting cardiac transplantation.79
Continuous Flow Pumps
Continuous flow pumps can be either axial or centrifugal, depending on the design of the impeller, and are designed to limit the interaction of moving parts. The MicroMed DeBakey VAD Child (MicroMed Cardiovascular, Inc., Houston) is an axial flow device currently available for use in the United States for children 5 to 16 years of age, through a US Food and Drug Administration (FDA) human device exemption. It was first introduced for use in March 2004, making it the first FDA-approved intracorporeal device for provision of left ventricular MCS as a bridge to transplantation in the pediatric population. Children must have New York Heart Association class IV end-stage heart failure that is refractory to medical management, be currently listed for cardiac transplantation, and have a BSA between 0.7 and 1.5 m2 to be considered candidates for DeBakey VAD Child support. The MicroMed DeBakey VAD Child is placed via median sternotomy using CPB. A titanium inlet cannula is sutured into the apex of the left ventricle via a circular core ventriculotomy, and a 12-mm gel-weave outflow graft is then anastomosed to the ascending aorta.80 A percutaneous cable containing the pump power and flow probe cables passes through the skin to the clinical data acquisition system. A separate unit, the “VADPAK,” contains a pump controller and two 12-volt batteries in a carrying case that can allow 5 to 8 hours of patient mobility.81
The Thoratec HeartMate II (see Fig. 19-4, B) is an intracorporeal, axial flow device that has been approved by the FDA for use as bridge-to-transplant and destination therapy in adults. Although recommended for use in patients with a BSA greater than 1.5 m2, its use has been reported in adolescents with a BSA as low as 1.3 m2. The HeartMate II has a low thromboembolic risk compared to other devices, and anticoagulation is managed using vitamin K antagonism and antiplatelet therapy (aspirin and dipyridamole). The portable system controller is also small enough to wear on a belt, allowing unencumbered movement for several hours, and potentially even discharge home.82 The VentrAssist (Ventracor Limited, Chatswood, Australia) was another small, implantable, continuous-flow device that had been successfully used in patients with a BSA as small as 1.1 m2 for prolonged support, allowing both bridge to recovery and to transplantation.83 Unfortunately this device is currently unavailable.
Outcomes
According to the Healthcare Cost and Utilization Project Kids’ Inpatient Database, 187 children underwent VAD implantation in 2006 in 67 different U.S. hospitals. High-volume large teaching hospitals reported significantly greater survival rates and reduced costs compared with other institutions.3 Regardless of the type of MCS chosen, it has become increasingly clear that the indication for and timing of initiation of support are major factors in determining outcome. Universally, survival has been greater in children who required support secondary to acute myocarditis or dilated cardiomyopathy, as compared with children who received support due to congenital heart defects or postcardiotomy failure.84
When feasible, VADs offer several major advantages over ECMO. First, the lack of an oxygenator simplifies the circuit, lessening anticoagulation requirements, as well as trauma to blood elements. Second, ECMO-supported patients have been shown to suffer greater rates of neurologic impairment than those supported with VADs, particularly in younger children with more complex heart disease.52 Additionally, evidence suggests that VAD support provides superior ventricular decompression and physiologic rest, promoting myocardial recovery in children with acute myocarditis or dilated cardiomyopathy by allowing normalization of ventricular geometry and reverse remodeling.85–87 A pilot study in children suggests that even short-term LVAD support of 1 to 2 weeks can reverse molecular remodeling.88
Children with chronic heart failure awaiting transplantation who experience progressive multiorgan dysfunction have been shown to benefit from the use of pulsatile VADs with potential recovery of pulmonary, renal, and hepatic function.89 A review of 55 pediatric patients revealed significantly increased survival rates in children who received Berlin Heart Excor support compared with those who received ECMO, despite a mean duration of support that was nearly three times as great in the Excor group.90 A multiinstitutional study reviewing the outcomes of 99 children bridged to cardiac transplantation with MCS, compared them with 2276 children listed for transplantation during the same era who did not require MCS. Significant findings included a lack of difference in survival rates between VAD-supported and non–VAD-supported children, along with similar survival rates for children who required BiVAD support versus LVAD support. An increasing trend in the number of children undergoing transplantation who required pretransplantation VAD support was also observed, with an increase in the use of long-term MCS devices in the most recent era. Children with long-term devices were significantly more likely to survive to transplantation than those with short-term devices. Ten children were “double bridged” from ECMO to VAD support before transplantation, with nine then undergoing successful transplantation. Not surprisingly, smaller, younger patients and those with congenital heart disease had less successful outcomes.91
Use of MCS in single-ventricle patients presents special challenges, particularly in patients with cavopulmonary anastomoses; the reported use of VADs in these patients remains limited compared to ECMO.47 Ventricular dysfunction in the failing Fontan may be addressed with VAD support of the systemic ventricle as long as pulmonary vascular resistance is not significantly increased.92 Management of anticoagulation may also prove difficult in this population because of liver dysfunction and coagulation factor abnormalities.93
In children with a cardiac etiology for MCS, irrespective of the type of mechanical support employed, if significant improvement in myocardial function has not been observed within 48 to 72 hours of institution of support, the child should be evaluated for potential cardiac transplantation.94 In the current era, earlier consideration for implementation of mechanical support with a staged approach, first using ECMO installation, with a transition to VAD therapy if necessary, has led to markedly improved survival rates.24
Perioperative Management and Complications of Mechanical Circulatory Support
Successful management of critically ill children on MCS requires the expertise of many groups of practitioners. The Hospital for Sick Children, Toronto, Ontario, developed an Interprofessional VAD Support Team involved in clinical care, education, and family support for patients receiving Berlin Heart MCS. In addition to physicians (cardiac surgeons, cardiac intensivists, heart failure and transplant cardiologists, hematologists and psychiatrists) and nurses (cardiac and critical care), team members include pharmacists, respiratory therapists, dieticians, social workers, physiotherapists, biomedical engineers, and perfusionists.95 Development of a team approach and use of interdisciplinary guidelines for care of these children can enhance communication, family support, and outcomes.96
Hemodynamics
During ECMO support, central venous pressure should remain low if adequate venous drainage is being achieved. Left atrial pressure should be closely monitored and can be estimated via echocardiographic evaluation of atrial septal position. An increase in left atrial pressure may indicate incomplete unloading of the left atrium and ventricle, potentially requiring a blade and/or balloon atrial septostomy97 or surgical placement of a left atrial vent. Anatomic issues, such as the presence of aortopulmonary collateral vessels, aortic insufficiency, or a patent ductus arteriosus, can also result in a persistently increased left atrial pressure.
Unlike ECMO support, support of the left ventricle with a VAD requires maintenance of effective right ventricular output to provide adequate left ventricular preload. Right ventricular failure, pulmonary hypertension, and arrhythmias can all limit left ventricular filling and must, therefore, be aggressively treated.98 Other potential causes of inadequate left ventricular filling include cannula malposition or low intravascular volume. Evaluation of central venous pressure can be used to evaluate volume status, and serial echocardiograms are useful for ongoing assessment of right ventricular function and estimation of right ventricular and pulmonary artery pressures. Clinical signs of right-sided heart failure include increased central venous or right atrial pressures, hepatomegaly, peripheral edema, and decreasing hemoglobin-oxygen saturations. Right ventricular function can be augmented pharmacologically with drugs such as milrinone or isoproterenol. Increased pulmonary artery pressures are best initially treated by ensuring adequate alveolar ventilation, and subsequently by using inhaled nitric oxide, if necessary. Mechanical issues, such as cardiac tamponade, can also negatively affect hemodynamics and can be diagnosed at the bedside with echocardiography. Inadequate left ventricular decompression can cause resultant increases in left atrial and pulmonary artery pressures and may be treated by augmenting LVAD flow; if necessary, biventricular support can be initiated or the child can be placed on ECMO support.99
Respiratory Considerations
Children on VADs receive only cardiac support. In the case of centrifugal VAD support, the trachea generally remains intubated and the lungs mechanically ventilated. Paracorporeal pulsatile VADs and implantable VADs, on the other hand, allow children to be weaned to extubation. Anatomic issues, such as an atrial septal defect, should be recognized as potential causes of hypoxemia due to right-to-left shunting.100 Children who receive left ventricular support via either a centrifugal or pulsatile VAD may also require inhaled nitric oxide to decrease pulmonary vascular resistance and improve right ventricular function.
Hematologic Considerations
Managing the anticoagulation continues to be one of the most challenging clinical issues in caring for children who require MCS, because of the risk of hemorrhage and/or thromboembolic complications.101 Ongoing hemorrhage around cannulation sites is frequent, particularly for those on ECMO. Of greater concern is hemorrhage in the gastrointestinal tract, the lungs, or, more devastatingly, intracranial hemorrhage; infarctions or thromboembolic events may also occur. Serial ultrasonography of the head may be used to monitor for intracranial bleeding in infants and neonates, and, when possible, computed tomography may be used in older children.
Although ECMO protocols vary at individual centers, ACTs are generally maintained between 180 and 200 seconds via use of a heparin infusion at 10 to 50 units/kg/hour. The use of heparin-bonded tubing can reduce the need for greater ACTs. The hematocrit is generally maintained between 40% and 50%, although efforts are made to limit transfusions, because most such children are potential candidates for cardiac transplantation. When necessary, leukocyte-reduced, cytomegalovirus-negative, irradiated red cells are administered. Platelet counts greater than 100,000/mm3 and fibrinogen concentrations greater than 100 mg/dL are maintained to minimize bleeding complications.102 In the presence of continued bleeding, cryoprecipitate and/or fresh frozen plasma may be added to the circuit prime and TEG may be helpful in assisting with appropriate use of blood products. In cases of persistent hemorrhage refractory to blood component administration, recombinant activated factor VII has been shown to significantly decrease bleeding and aid in normalizing the TEG profile,103–105 although disastrous clotting of circuitry has also been reported with its use.106 Hemolysis may be monitored by measurement of plasma-free hemoglobin, with increasing concentrations potentially representing the development of thrombus in the circuit.
Without an oxygenator in the circuit, anticoagulation requirements are not as stringent for children on VADs, and their ACTs may be maintained between 140 and 180 seconds. After placement of a Berlin Heart Excor, unfractionated heparin infusions are initially used with a target aPTT of 60 to 70 seconds. The use of argatroban has been described in children with heparin-induced thrombocytopenia type II.107 Longer term anticoagulation includes the use of LMWH with anti–factor Xa activity monitoring.108,109 After normalization of the platelet count and function, aspirin and dipyridamole are started, with monitoring via TEG and platelet aggregation tests, with a target activation of 30%. Once a child is tolerating enteral feedings, a transition to warfarin may occur. Although embolic and bleeding complications remain problematic, with this management strategy a marked increase in survival has been noted in children undergoing Berlin Heart Excor support compared to earlier regimens.110
Although hemolysis and thrombosis may be less frequent with continuous flow devices, the frequent development of acquired von Willebrand syndrome has been described in adult patients, most likely occurring secondary to the shear stress of the pump, with unfolding and cleavage of the high-molecular-weight multimers of von Willebrand factor. This is also thought to account for the increased incidence of gastrointestinal bleeding noted in these patients, though not all patients with documented acquired von Willebrand syndrome display increased bleeding tendencies.111,112
Prevention of Infection
Infection is a constant concern, particularly in children requiring intermediate- to long-term VAD support. Devices with larger surface area and areas of turbulent flow have been correlated with greater infection rates due to increased adherence of blood-borne pathogens; therefore, smaller, fully implantable devices and devices with improved flow dynamics may continue to facilitate decreases in the incidence of infection.113,114 Devices, such as the Berlin Heart Excor, that facilitate removal of tracheal tubes, indwelling catheters and intravenous lines, and minimize the need for blood transfusions, also offer advantages in minimizing the risk of device-related infections.75,115 Deep wound complications of inflow and outflow cannulas have been successfully treated with vacuum-assisted wound closure systems.116
The use of mechanical support devices can result in immunologic dysfunction, further increasing infection risk.117 Signs and symptoms of infection can be subtle, and a need for increasing inotropic support to maintain mean arterial pressure can often be a harbinger of infection. Endogenous reactions to infection can also result in activation of the coagulation cascade, increasing the difficulty of anticoagulation management.118 Many children prophylactically receive antibiotics with gram-positive coverage, as well as oral nystatin for fungal prophylaxis.
Anesthetic Considerations
In preparing for the anesthetic care of infants and children, when ECMO or VAD support is being used or when implementation of MCS is imminent, initial planning centers on two main areas of concern: the child’s current status and the location of the proposed procedure (Table 19-4). The need for emergency surgery, the child’s condition at the time of surgery, the presence of preoperative multiorgan failure, and the need for CPB with cross-clamping of the aorta have all been shown to increase perioperative risk.119
TABLE 19-4 Anesthetic Considerations
Preoperative laboratory evaluation should include CBC with platelet count, electrolytes, blood urea nitrogen and creatinine concentrations, PT and partial thromboplastin time, and liver function tests. Concentrations of BNP, produced in the myocardium, serve as a marker of ventricular overloading and have been shown in adult and pediatric patients with congenital heart disease to correlate with the degree of ventricular dysfunction.120,121 Serial BNP concentrations in pediatric patients supported with ECMO have also been used to predict clinical outcomes, with greater BNP concentrations noted after termination of ECMO support in nonsurvivors than in children who ultimately survived.122
For patients undergoing placement of a VAD, etomidate is generally an advantageous drug for induction of anesthesia because it does not result in myocardial depression at clinically relevant concentrations, even in children with severely compromised ventricular function.123 Judicious doses of opioids and benzodiazepines may be added, while the choice of a neuromuscular blocking agent is often determined by the presence of compromised hepatic or renal function. Adequate depth of anesthesia should be ensured before tracheal intubation, to avoid abrupt increases in pulmonary vascular resistance, particularly in children with marginal right ventricular function. In children undergoing device placement, induction drugs should be given incrementally, because the onset time may be abnormally slow owing to depressed ventricular function. Children may exhibit decreased responsiveness to β-adrenergic agonists due to depletion of myocardial catecholamines.124 Hypotension on induction of anesthesia and decreased responsiveness to catecholamines may also be observed in children who have been chronically receiving angiotensin-converting–enzyme inhibitors for afterload reduction preoperatively.125–127 Opioids, benzodiazepines, and neuromuscular blocking agents are generally used for anesthetic maintenance before ECMO cannulation or initiation of CPB for VAD implementation, with the express goal of maintaining adequate cardiac output and resultant systemic perfusion to end organs. Trends in serum lactate concentrations, mixed venous hemoglobin-oxygen saturations, and the presence or absence of metabolic acidosis are useful indices for evaluating the adequacy of cardiac output.
Not surprisingly, with the steadily increasing use of VAD support in infants and children, a growing demand for anesthesia for noncardiac surgeries during the period of support has also occurred. Transport and care of these children often requires the assistance of respiratory therapists and the availability of a pump specialist. Temporary conversion from warfarin or LMWH to unfractionated heparin infusion should be discussed with the team caring for the patient, including the hematologist, before surgery, and appropriate blood products should be ordered. For children on VAD support, appropriate pump function will continue independently of the induction drugs used, as long as adequate preload is maintained and no acute decrease in systemic vascular resistance occurs. In a series of children with in situ Berlin Heart Excor support undergoing noncardiac surgeries, anesthetic induction with ketamine was found to be less likely to induce hypotension requiring treatment with fluid bolus or α-adrenergic agonists.128 Inspection of the pump chamber for wrinkling of the membrane assists in deciding whether the use of a fluid bolus or an α-adrenergic agonist is more appropriate. In children who are only on LVAD support, care should be taken to avoid increases in pulmonary vascular resistance, as the likeliest cause of decompensation in such patients is right ventricular failure. Nitric oxide and appropriate vasoactive drugs should be readily available for use if needed. When possible, the use of spontaneous ventilation is thought to improve venous return and hemodynamic stability.57,129
Future Directions
The Pediatric Circulatory Support Program (PCSP) of the NHLBI was established to foster development of circulatory support devices suitable for children from 2 to 25 kg. Five contracts were awarded in early 2004 and concluded in 2009, with devices under development including a next-generation ECMO system (Pediatric Cardiopulmonary Assist System, Ension, Inc., Pittsburgh), an implantable VAD (PediPump, The Cleveland Clinic Foundation, Cleveland), an intraventricular pump (Pediatric Jarvik 2000, Jarvik Heart Inc., New York), a pulsatile VAD (Pediatric Ventricular Assist Device, The Pennsylvania State University, Hershey, Pa.), and a miniaturized blood pump (PediaFlow VAD, University of Pittsburgh) (Fig. 19-6). In 2008 the NHLBI requested proposals for the Pumps for Kids, Infants, and Neonates (PumpKIN) program, with three of the five contractors from the NHLBI PCSP program receiving program awards in January 2010. Clinical trials are anticipated to begin in 2013.130
Adachi I, Fraser C. Mechanical circulatory support for infants and small children. Semin Thorac Cardiovasc Surg Pediatr Card Surg Annu. 2011;14:38–44.
Almond C, Singh T, Gauvreau K, et al. Extracorporeal membrane oxygenation for bridge to heart transplantation among children in the United States: analysis of data from the Organ Procurement and Transplant Network and Extracorporeal Life Support Organization Registry. Circulation. 2011;123:2975–2984.
Baldwin J, Borovetz H, Duncan B, et al. The National Heart, Lung, and Blood Institute Pediatric Circulatory Support Program: a summary of the 5-year experience. Circulation. 2011;123:1233–1240.
Barrett C, Bratton S, Salvin J, et al. Neurological injury after extracorporeal membrane oxygenation use to aid pediatric cardiopulmonary resuscitation. Pediatr Crit Care Med. 2009;10:445–451.
Blume E, Naftel D, Bastardi H, et al. Outcomes of children bridged to heart transplantation with ventricular assist devices: a multi-institutional study. Circulation. 2006;113:2313–2319.
Hetzer R, Potapov E, Alexi-Meskishvili V, et al. Single-center experience with treatment of cardiogenic shock in children by pediatric ventricular assist devices. J Thorac Cardiovasc Surg. 2011;141:616–623.
Jefferies J, Price J, Morales D. Mechanical support in childhood heart failure. Heart Fail Clin. 2010;6:559–573.
Mossad E, Motta P, Rossano J, et al. Perioperative management of pediatric patients on mechanical cardiac support. Paediatr Anaesth. 2011;21:585–593.
1 Rossano J, Zafar F, Graves D, et al. Prevalence of heart failure hospitalizations and risk factors for mortality in pediatric patients: an analysis of a nationwide sampling of hospital discharges in the United States (abstract). Circulation. 2009;120:S586.
2 Rose E, Gelijns A, Moskowitz A, et al. Long-term mechanical left ventricular assistance for end-stage heart failure. N Engl J Med. 2001;345:1435–1443.
3 Morales D, Zafar F, Rossano J, et al. Use of ventricular assist devices in children across the United States: analysis of 7.5 million pediatric hospitalizations. Ann Thorac Surg. 2010;90:1313–1318.
4 Baldwin J, Borovetz H, Duncan B, et al. The National Heart, Lung, and Blood Institute Pediatric Circulatory Support Program. Circulation. 2006;113:147–155.
5 Bautista-Hernandez V, Thiagarajan R, Fynn-Thompson F, et al. Preoperative extracorporeal membrane oxygenation as a bridge to cardiac surgery in children with congenital heart disease. Ann Thorac Surg. 2009;88:1306–1311.
6 Walker G, McLeod K, Brown K, et al. Extracorporeal life support as a treatment of supraventricular tachycardia in infants. Pediatr Crit Care Med. 2003;4:52–54.
7 Tsai F, Wang Y, Huang Y, et al. Extracorporeal life support to terminate refractory ventricular tachycardia. Crit Care Med. 2007;35:1673–1676.
8 Jhang W, Lee S, Seo D, et al. Mechanical circulatory support to control medically intractable arrhythmias in pediatric patients after cardiac surgery. Korean Circ J. 2010;40:471–474.
9 Dyamenahalli U, Tuzcu V, Fontenot E, et al. Extracorporeal membrane oxygenation support for intractable primary arrhythmias and complete congenital heart block in newborns and infants: Short-term and medium-term outcomes. Pediatr Crit Care Med. 2012;13:47–52.
10 Carmichael T, Walsh E, Roth S. Anticipatory use of venoarterial extracorporeal membrane oxygenation for a high-risk interventional cardiac procedure. Respir Care. 2002;47:1002–1006.
11 Allan C, Thiagarajan R, Armsby L, et al. Emergent use of extracorporeal membrane oxygenation during pediatric cardiac catheterization. Pediatr Crit Care Med. 2006;7:212–219.
12 Langley S, Sheppard S, Tsang V, et al. When is extracorporeal life support worthwhile following repair of congenital heart disease in children? Eur J Cardiothorac Surg. 1998;13:520–525.
13 Imamura M, Dossey A, Jaquiss R. Reoperation and mechanical circulatory support after repair of anomalous origin of the left coronary artery from the pulmonary artery: a twenty-year experience. Ann Thorac Surg. 2011;92:167–173.
14 Stege D, Kiski D, Tjan T, et al. 1-year left ventricular assist device (LVAD) experience as bridge to heart transplantation in an infant with Bland-White-Garland syndrome. Thorac Cardiovasc Surg. 2010;58(Suppl 2):S167–S169.
15 Ungerleider R, Shen I, Yeh T, et al. Routine mechanical ventricular assist following the Norwood procedure-improved neurologic outcome and excellent hospital survival. Ann Thorac Surg. 2004;77:18–22.
16 Rajagopal S, Almond C, Laussen P, et al. Extracorporeal membrane oxygenation for the support of infants, children, and young adults with acute myocarditis: a review of the Extracorporeal Life Support Organization registry. Crit Care Med. 2010;38:382–387.
17 Teele S, Allan C, Laussen P, et al. Management and outcomes in pediatric patients presenting with acute fulminant myocarditis. J Pediatr. 2011;158:638–643.
18 Tissot C. Outcome of extracorporeal membrane oxygenation for early primary graft failure after pediatric heart transplantation. JACC. 2009;54:730–737.
19 Huang S, Wu E, Chi N, et al. Perioperative extracorporeal membrane oxygenation support for critical pediatric airway surgery. Eur J Pediatr. 2007;166:1129–1133.
20 Maclaren G, Butt W, Best D, et al. Extracorporeal membrane oxygenation for refractory septic shock in children: one institution’s experience. Pediatr Crit Care Med. 2007;8:447–451.
21 Puri V, Epstein D, Raithel S, et al. Extracorporeal membrane oxygenation in pediatric lung transplantation. J Thorac Cardiovasc Surg. 2010;140:427–432.
22 Jackson A, Cropper J, Pye R, et al. Use of extracorporeal membrane oxygenation as a bridge to primary lung transplant; 3 consecutive, successful cases and a review of the literature. J Heart Lung Transplant. 2008;27:348–352.
23 Shigemura N, Bermudez C, Bhama J, et al. Successful lung retransplantation after extended use of extracorporeal membrane oxygenation as a bridge. Transplant Proc. 2011;43:2063–2065.
24 Hetzer R, Potapov E, Alexi-Meskishvili V, et al. Single-center experience with treatment of cardiogenic shock in children by pediatric ventricular assist devices. J Thorac Cardiovasc Surg. 2011;141:616–623.
25 Raymond T, Cunnyngham C, Thompson M, et al. Outcomes among neonates, infants, and children after extracorporeal cardiopulmonary resuscitation for refractory inhospital pediatric cardiac arrest: a report from the National Registry of Cardiopulmonary Resuscitation. Pediatr Crit Care Med. 2010;11:362–371.
26 Chan T, Thiagarajan R, Frank D, et al. Survival after extracorporeal cardiopulmonary resuscitation in infants and children with heart disease. J Thorac Cardiovasc Surg. 2008;136:984–992.
27 Thiagarajan R, Laussen P, Rycus P, et al. Extracorporeal membrane oxygenation to aid cardiopulmonary resuscitation in infants and children. Circulation. 2007;116:1693–1700.
28 Kelly R, Porter P, Neier A, et al. Duration of cardiopulmonary resuscitation before extracorporeal rescue: how long is not long enough? ASAIO J. 2005;51:665–667.
29 Morris M, Wernovsky G, Nadkarni V. Survival outcomes after extracorporeal cardiopulmonary resuscitation instituted during active chest compressions following refractory in-hospital pediatric cardiac arrest. Pediatr Crit Care Med. 2004;5:440–446.
30 Barrett C, Bratton S, Salvin J, et al. Neurological injury after extracorporeal membrane oxygenation use to aid pediatric cardiopulmonary resuscitation. Pediatr Crit Care Med. 2009;10:445–451.
31 Duncan B. Mechanical circulatory support for infants and children with cardiac disease. Ann Thorac Surg. 2002;73:1670–1677.
32 Duncan B. Pediatric mechanical circulatory support. ASAIO J. 2005;51:ix–xiv.
33 Smedira N, Blackstone E. Postcardiotomy mechanical support: risk factors and outcomes. Ann Thorac Surg. 2001;71:S60–S66.
34 Pantalos G, Minich L, Tani L, et al. Estimation of timing errors for the intraaortic balloon pump use in pediatric patients. ASAIO J. 1999;45:166–171.
35 Pinkney K, Minich L, Tani L, et al. Current results with intraaortic balloon pumping in infants and children. Ann Thorac Surg. 2002;73:887–891.
36 Moran A, Blume E, Quinn R. Intra-aortic balloon pump use in the failing Fontan circulation. Congenit Heart Dis. 2008;3:60–62.
37 Akomea-Agyin C, Kejriwal N, Franks R. Intraaortic balloon pumping in children. Ann Thorac Surg. 1999;67:1415–1420.
38 Bartlett R, Gazzaniga A, Fong S, et al. Extracorporeal membrane oxygenator support for cardiopulmonary failure. Experience in 28 cases. J Thorac Cardiovasc Surg. 1977;73:375–386.
39 Cabrera A, Prodhan P, Cleves M, et al. Interhospital transport of children requiring extracorporeal membrane oxygenation support for cardiac dysfunction. Congenit Heart Dis. 2011;6:202–208.
40 Haines N, Rycus P, Zwischenberger J, et al. Extracorporeal Life Support Registry report 2008: neonatal and pediatric cardiac cases. ASAIO J. 2009;55:111–116.
41 Jacobs J, Ojito J, McConaghey T, et al. Rapid cardiopulmonary support for children with complex congenital heart disease. Ann Thorac Surg. 2000;70:742–750.
42 Muehrcke D, McCarthy P, Stewart R, et al. Extracorporeal membrane oxygenation for postcardiotomy cardiogenic shock. Ann Thorac Surg. 1996;61:684–691.
43 Thourani V, Kirshbom P, Kanter K, et al. Venoarterial extracorporeal membrane oxygenation (VA-ECMO) in pediatric cardiac support. Ann Thorac Surg. 2006;82:38–44.
44 Jaggers J, Forbess J, Shah A, et al. Extracorporeal membrane oxygenation for infant postcardiotomy support: significance of shunt management. Ann Thorac Surg. 2000;69:1476–1483.
45 Ravishankar C, Dominguez T, Kreutzer J, et al. Extracorporeal membrane oxygenation after stage I reconstruction for hypoplastic left heart syndrome. Pediatr Crit Care. 2006;7:319–323.
46 Allan C, Thiagarajan R, DelNido P, et al. Indication for initiation of mechanical circulatory support impacts survival of infants with shunted single-ventricle circulation supported with extracorporeal membrane oxygenation. J Thorac Cardiovasc Surg. 2007;133:660–667.
47 Rood K, Teele S, Barrett C, et al. Extracorporeal membrane oxygenation support after the Fontan operation. J Thorac Cardiovasc Surg. 2011;142:504–510.
48 Shen I, Levy F, Benak A, et al. Left ventricular dysfunction during extracorporeal membrane oxygenation in a hypoxemic swine model. Ann Thorac Surg. 2001;71:868–871.
49 Aharon A, Drinkwater D, Churchwell K, et al. Extracorporeal membrane oxygenation in children after repair of congenital cardiac lesions. Ann Thorac Surg. 2001;72:2095–2101.
50 Kumar T, Zurakowski D, Dalton H, et al. Extracorporeal membrane oxygenation in postcardiotomy patients: factors influencing outcome. J Thorac Cardiovasc Surg. 2010;140:330–336. e2
51 Almond C, Singh T, Gauvreau K, et al. Extracorporeal membrane oxygenation for bridge to heart transplantation among children in the United States: analysis of data from the Organ Procurement and Transplant Network and Extracorporeal Life Support Organization Registry. Circulation. 2011;123:2975–2984.
52 Ibrahim A, Duncan B, Blume E, et al. Long-term follow-up of pediatric cardiac patients requiring mechanical circulatory support. Ann Thorac Surg. 2000;69:186–192.
53 Williams M, Quaegebeur J, Hsu D, et al. Biventricular assist device as a bridge to transplantation in a pediatric patient. Ann Thorac Surg. 1996;62:578–580.
54 Pavie A, Leger P. Physiology of univentricular versus biventricular support. Ann Thorac Surg. 1996;61:347–349.
55 Morgan I, Codispoti M, Sanger K, et al. Superiority of centrifugal pump over roller pump in paediatric cardiac surgery: prospective randomised trial. Eur J Cardiothorac Surg. 1998;13:526–532.
56 Bennett M, Horton S, Thuys C, et al. Pump-induced haemolysis: a comparison of short-term ventricular assist devices. Perfusion. 2004;19:107–111.
57 Mossad E, Motta P, Rossano J, et al. Perioperative management of pediatric patients on mechanical cardiac support. Paediatr Anaesth. 2011;21:585–593.
58 Svitek R, Smith D, Magovern J, et al. In vitro evaluation of the TandemHeart pediatric centrifugal pump. ASAIO J. 2007;53:747–753.
59 Del Nido P, Duncan B, Mayer J, et al. Left ventricular assist device improves survival in children with left ventricular dysfunction after repair of anomalous left coronary artery from the pulmonary artery. Ann Thorac Surg. 1999;67:169–172.
60 Duncan B, Hraska V, Jonas R, et al. Mechanical circulatory support in children with cardiac disease. J Thorac Cardiovasc Surg. 1999;117:529–542.
61 Stiller B, Weng Y, Hubler M, et al. Pneumatic pulsatile ventricular assist devices in children under 1 year of age. Euro J Cardiothorac Surg. 2005;28:234–239.
62 Kaczmarek I, Mair H, Groetzner J, et al. Mechanical circulatory support in infants and adults with the MEDOS/HIA assist device. Artif Organs. 2005;29:857–860.
63 DiGiorgi P, Rao V, Naka Y, et al. Which patient, which pump? J Heart Lung Transplant. 2003;22:221–235.
64 Copeland J, Arabia F, Smith R. Bridge to transplantation with a Thoratec left ventricular assist device in a 17-kg child. Ann Thorac Surg. 2001;71:1003–1004.
65 Ishino K, Loebe M, Uhlemann F, et al. Circulatory support with paracorporeal pneumatic ventricular assist device (VAD) in infants and children. Euro J Cardiothorac Surg. 1997;11:965–972.
66 Reinhartz O, Copeland J, Farrar D. Thoratec ventricular assist devices in children with less than 1.3 m2 of body surface area. ASAIO J. 2003;49:727–730.
67 Hill J, Reinhartz O. Clinical outcomes in pediatric patients implanted with Thoratec ventricular assist device. Semin Thorac Cardiovasc Surg Pediatr Card Surg Annu. 2006;9:115–122.
68 Reinhartz O, Hill J, Al-Khaldi A, et al. Thoratec ventricular assist devices in pediatric patients: update on clinical results. ASAIO J. 2005;51:501–503.
69 Hetzer R, Henning E, Schiessler A, et al. Mechanical circulatory support and heart transplantation. J Heart Lung Transplant. 1992;11(4 Pt 2):S175–S181.
70 Hennig E. Design criteria for pediatric mechanical support systems. Ann Concert Action Heart. 1992:39–46.
71 Morales D, Almond C, Jaquiss R, et al. Bridging children of all sizes to cardiac transplantation: the initial multicenter North American experience with the Berlin Heart EXCOR ventricular assist device. J Heart Lung Transplant. 2011;30:1–8.
72 Hetzer R, Potapov E, Stiller B, et al. Improvement in survival after mechanical circulatory support with pneumatic pulsatile ventricular assist devices in pediatric patients. Ann Thorac Surg. 2006;82:917–925.
73 Merkle F, Boettcher W, Stiller B, et al. Pulsatile mechanical cardiac assistance in pediatric patients with the Berlin Heart ventricular assist device. J Extra Corpor Technol. 2003;35:115–120.
74 Hetzer R, Loebe M, Potapov E, et al. Circulatory support with pneumatic paracorporeal ventricular assist device in infants and children. Ann Thorac Surg. 1998;66:1498–1506.
75 Stiller B, Lemmer J, Merkle F, et al. Consumption of blood products during mechanical circulatory support in children: comparison between ECMO and a pulsatile ventricular assist device. Intensive Care Med. 2004;30:1814–1820.
76 Hetzer R, Alexi-Meskishvili V, Weng Y, et al. Mechanical cardiac support in the young with the Berlin Heart EXCOR pulsatile ventricular assist device: 15 years’ experience. Semin Thorac Cardiovasc Surg Pediatr Card Surg Annu. 2006;9:99–108.
77 Herwig V, Severin M, Waldenberger F, et al. Medos/HIA-assist system: first experiences with mechanical circulatory assist in infants and children. Int J Artif Organs. 1997;20:692–694.
78 Konertz W, Hotz H, Schneider M, et al. Clinical experience with the MEDOS HIA-VAD system in infants and children: a preliminary report. Ann Thorac Surg. 1997;63:1138–1144.
79 Kaczmarek I, Sachweh J, Groetzner J, et al. Mechanical circulatory support in pediatric patients with the MEDOS assist device. ASAIO J. 2005;51:498–500.
80 Fraser C, Carberry K, Owens W, et al. Preliminary experience with the MicroMed DeBakey pediatric ventricular assist device. Semin Thorac Cardiovasc Surg Pediatr Card Surg Annu. 2006;9:109–114.
81 Wieselthaler G, Schima H, Hiesmayr M, et al. First clinical experience with the DeBakey VAD continuous-axial-flow pump for bridge to transplantation. Circulation. 2000;101:356–359.
82 Owens WR, Bryant R, 3rd., Dreyer WJ, Price JF, Morales DL. Initial clinical experience with the HeartMate II ventricular assist system in a pediatric institution. Artif Organs. 2010;34:600–603.
83 Ruygrok P, Esmore D, Alison P, et al. Pediatric experience with the VentrAssist LVAD. Ann Thorac Surg. 2008;86:622–626.
84 Stiller B, Hetzer R, Weng Y, et al. Heart transplantation in children after mechanical circulatory support with pulsatile pneumatic assist device. J Heart Lung Transplant. 2003;22:1201–1208.
85 Duncan B, Bohn DJ, Atz A, et al. Mechanical circulatory support for the treatment of children with acute fulminant myocarditis. J Thorac Cardiovasc Surg. 2001;122:440–448.
86 Levin H, Oz M, Chen J, et al. Reversal of chronic ventricular dilation in patients with end-stage cardiomyopathy by prolonged mechanical unloading. Circulation. 1995;91:2717–2720.
87 Burkhoff D, Klotz S, Mancini D. LVAD-induced reverse remodeling: basic and clinical implications for myocardial recovery. J Card Fail. 2006;12:227–239.
88 Mohapatra B, Vick G, Fraser C, et al. Short-term mechanical unloading and reverse remodeling of failing hearts in children. J Heart Lung Transplant. 2010;29:98–104.
89 El-Banayosy N, Arusoglu L, Kleikamp G, et al. Recovery of organ dysfunction during bridging to heart transplantation in children and adolescents. Int J Artif Organs. 2003;26:395–400.
90 Imamura M, Dossey A, Prodhan P, et al. Bridge to cardiac transplant in children: Berlin Heart versus extracorporeal membrane oxygenation. Ann Thorac Surg. 2009;87:1894–1901.
91 Blume E, Naftel D, Bastardi H, et al. Outcomes of children bridged to heart transplantation with ventricular assist devices: a multi-institutional study. Circulation. 2006;113:2313–2319.
92 Russo P, Wheeler A, Russo J, et al. Use of a ventricular assist device as a bridge to transplantation in a patient with single ventricle physiology and total cavopulmonary anastomosis. Paediatr Anaeasth. 2008;18:320–324.
93 VanderPluym C, Rebeyka I, Ross D, et al. The use of ventricular assist devices in pediatric patients with univentricular hearts. J Thorac Cardiovasc Surg. 2011;141:588–590.
94 Del Nido P, Armitage J, Fricker F, et al. Extracorporeal membrane oxygenation support as a bridge to pediatric heart transplantation. Circulation. 1994;90:II66–II69.
95 Furness S, Hyslop-St George C, Pound B, et al. Development of an interprofessional pediatric ventricular assist device support team. ASAIO J. 2008;54:483–485.
96 Staveski S, Avery S, Rosenthal D, et al. Implementation of a comprehensive interdisciplinary care coordination of infants and young children on Berlin Heart ventricular assist devices. J Cardiovasc Nurs. 2011;26:231–238.
97 Seib P, Faulkner S, Erickson C, et al. Blade and balloon atrial septostomy for left heart decompression in patients with severe ventricular dysfunction on extracorporeal membrane oxygenation. Catheter Cardiovasc Interv. 1999;46:179–186.
98 Van Meter C. Right heart failure: best treated by avoidance. Ann Thorac Surg. 2001;71:S220–S222.
99 Klotz S, Naka Y, Oz M, et al. Biventricular assist device-induced right ventricular reverse structural and functional remodeling. J Heart Lung Transplant. 2005;24:1195–1201.
100 Heath M, Dickstein M. Perioperative management of the left ventricular assist device recipient. Prog Cardiovasc Dis. 2000;43:47–54.
101 Amir O, Bracey A, Smart F, et al. A successful anticoagulation protocol for the first HeartMate II implantation in the United States. Tex Heart Inst J. 2005;32:399–401.
102 Dela Cruz T, Stewart D, Winston S, et al. Risk factors for intracranial hemorrhage in the extracorporeal membrane oxygenation patient. J Perinatol. 1997;17:18–23.
103 Wittenstein B, Ng C, Ravn H, et al. Recombinant factor VII for severe bleeding during extracorporeal membrane oxygenation following open heart surgery. Pediatr Crit Care Med. 2005;6:473–476.
104 Davis M, Andersen N, Johansson P, et al. Use of thromboelastograph and factor VII for the treatment of postoperative bleeding in a pediatric patient on ECMO after cardiac surgery. J Extracorpor Technol. 2006;38:165–167.
105 Heise D, Brauer A, Quintel M. Recombinant activated factor VII (Novo7) in patients with ventricular assist devices: case report and review of the current literature. J Cardiothorac Surg. 2007;2:47.
106 Swaminathan M, Shaw A, Greenfield R, et al. Fatal thrombosis after Factor VII administration during extracorporeal membrane oxygenation. J Cardiothorac Vasc Anesth. 2008;22:259–260.
107 Schmitz M, Massicotte P, Faulkner S, et al. Management of a pediatric patient on the Berlin Heart Excor ventricular assist device with argatroban after heparin-induced thrombocytopenia. ASAIO J. 2008;54:546–547.
108 Camboni D, Schmid C, Rellensmann G, et al. Enoxiparin for long-term anticoagulation with the pediatric EXCOR left ventricular assist device. Interact Cardiovasc Thorac Surg. 2005;4:561–562.
109 Mildh L, Tynkkynen P, Mattila I, et al. Initial experience of enoxaparine as anticoagulant during mechanical circulatory support in children. Interact Cardiovasc Thorac Surg. 2006;5:499–501.
110 Drews T, Stiller B, Hubler M, et al. Coagulation management in pediatric mechanical circulatory support. ASAIO J. 2007;53:640–645.
111 Crow S, Chen D, Milano C, et al. Acquired von Willebrand syndrome in continuous-flow ventricular assist device recipients. Ann Thorac Surg. 2010;90:1263–1269.
112 Slaughter M. Hematologic effects of continuous flow left ventricular assist devices. J Cardiovasc Trans Res. 2010;3:618–624.
113 Baddour L, Bettman M, Bolger A, et al. Nonvalvular cardiovascular device-related infections. Circulation. 2003;108:2015–2031.
114 Goldstein D. Worldwide experience with the MicroMed DeBakey ventricular assist device as a bridge to transplantation. Circulation. 2003;108:II272–II277.
115 Chinn R, Dembitsky W, Eaton L, et al. Multicenter experience: prevention and management of left ventricular assist device infections. ASAIO J. 2005;51:461–470.
116 Kouretas P, Burch P, Kaza A, et al. Management of deep wound complications with vacuum-assisted therapy after Berlin Heart EXCOR ventricular assist device placement in the pediatric population. Artif Organs. 2009;33:922–925.
117 Itescu S, Ankersmit J, Kocher A, et al. Immunobiology of left ventricular assist devices. Prog Cardiovasc Dis. 2000;43:67–80.
118 Potapov E, Stiller B, Hetzer R. Ventricular assist devices in children: current achievements and future perspectives. Pediatr Transplant. 2007;11:241–255.
119 Schindler E, Muller M, Kwapisz M, et al. Ventricular cardiac-assist devices in infants and children: anesthetic considerations. J Cardiothorac Vasc Anesth. 2003;17:617–621.
120 Law Y, Keller B, Feingold B, et al. Usefulness of plasma B-type natriuretic peptide to identify ventricular dysfunction in pediatric and adult patients with congenital heart disease. Am J Cardiol. 2005;95:474–478.
121 Heise G, Lemmer J, Weng Y, et al. Biomarker responses during mid-term mechanical cardiac support in children. J Heart Lung Transplant. 2008;27(2):150–157.
122 Huang S, Wu E, Ko W, et al. Clinical implication of blood levels of B-type natriuretic peptide in pediatric patients on mechanical circulatory support. Ann Thorac Surg. 2006;81:2267–2272.
123 Sprung J, Ogletree-Hughes M, Moravec C, et al. The effects of etomidate on the contractility of failing and nonfailing human heart muscle. Anesth Analg. 2000;91:68–75.
124 Bristow M, Hershberger R, Port J, et al. Beta 1- and beta 2-adrenergic receptor-mediated adenylate cyclase stimulation in nonfailing and failing human ventricular myocardium. Mol Pharmacol. 1989;35:295–303.
125 Licker M, Neidhart P, Lustenberger S, et al. Long-term angiotensin-converting enzyme inhibitor treatment attenuates adrenergic responsiveness without altering hemodynamic control in patients undergoing cardiac surgery. Anesthesiology. 1996;84:789–800.
126 Colson P, Saussine M, Seguin J, et al. Hemodynamic effects of anesthesia in patients chronically treated with angiotensin-converting enzyme inhibitors. Anesth Analg. 1992;74:805–808.
127 Behnia R, Molteni A, Igic R. Angiotensin-converting enzyme inhibitors: mechanisms of action and implications in anesthesia practice. Curr Pharm Des. 2003;9:763–776.
128 Cave D, Fry K, Buchholz H. Anesthesia for noncardiac procedures for children with a Berlin Heart EXCOR pediatric ventricular assist device: a case series. Paediatr Anaesth. 2010;20:647–659.
129 Pratap J, Wilmshurst S. Anesthetic management of children with in situ Berlin Heart EXCOR. Paediatr Anaesth. 2010;20:812–820.
130 Baldwin J, Borovetz H, Duncan B, et al. The national heart, lung, and blood institute pediatric circulatory support program: a summary of the 5-year experience. Circulation. 2011;123:1233–1240.