34 Management of Acute Ischemic Stroke
Stroke is currently recognized as the third most common cause of death and the leading cause of adult morbidity in the United States, affecting nearly 795,000 people annually.1 Acute ischemic stroke is a true medical emergency and must be treated in a swift yet pragmatic approach. The rationale for acute ischemic stroke treatment is based on the concept of the ischemic penumbra. When an arterial occlusion occurs, an area of irreversibly infarcted brain (i.e., core infarct) is surrounded by a region that has reduced blood flow impairing function (i.e., ischemic penumbra), although not of sufficient severity to result in irreversible infarction. If adequate blood flow can be restored within a critical time frame, this area of at-risk tissue may be salvageable and return to normal function. Experimental models of stroke indicate that lower levels of blood flow are tolerated for brief periods, whereas slightly higher blood flow can be maintained for several hours without developing infarction.2,3 The precise relationships between blood flow levels and duration for human stroke are still being elucidated, but the prevailing concept is that the more quickly restoration of blood flow occurs, the greater the probability that the salvageable tissue will be spared from permanent damage.
In 1995, the National Institute of Neurological Disorders and Stroke (NINDS) rt-PA Stroke Study Group showed for the first time an improvement in ischemic stroke outcome with acute treatment.4 At present, intravenous (IV) recombinant tissue plasminogen activator (tPA) is the only treatment that has been approved by the U.S. Food and Drug Administration (FDA) for acute ischemic stroke patients presenting within 3 hours of symptom onset. Other treatments for acute ischemic stroke—intraarterial thrombolysis, devices for mechanical clot disruption, and neuroprotective agents—continue to be investigated.
Emergent Stroke Evaluation
A stroke team typically consists of individuals from multiple disciplines with specialized knowledge and interest in acute stroke care and often includes a vascular neurologist, nursing coordinator, and where available, a neurointerventionalist. A neurologist performs a National Institutes of Health Stroke Scale (NIHSS) (Table 34-1) assessment as an additional rapid neurologic assessment tool to better localize and ascertain the degree of clinical deficit. The score may impact which therapies may be available to patients. For patients presenting with stroke-like symptoms while already hospitalized in an intensive care unit (ICU) or other hospital floor, the algorithm should be identical.
1A. Level of Consciousness (LOC) | 1B. LOC Questions | 1C. LOC Commands |
---|---|---|
0 = Alert 1 = Not alert, but arousable 2 = Not alert, obtunded 3 = Coma |
Ask the month and his/her age. 0 = Answers both correctly 1 = Answers one correctly 2 = Answers neither correctly |
Open and close the eyes. Open and close the non-paretic hand. 0 = Performs both tasks correctly 1 = Performs one task correctly 2 = Performs neither task correctly |
2. Best Gaze (Horizontal) | 3. Visual Fields | 4. Facial Palsy |
0 = Normal 1 = Partial gaze palsy 2 = Forced deviation or total gaze paresis |
0 = No visual loss 1 = Partial hemianopia 2 = Complete hemianopia 3 = Bilateral hemianopia |
0 = Normal 1 = Minor paralysis 2 = Partial paralysis (total or near total paralysis of lower face) 3 = Complete paralysis of upper and lower face |
5. Motor Arm | 6. Motor Leg | 7. Limb Ataxia |
Right Arm extended with palms down 90 degrees (if sitting) or 45 degrees (if supine) for 10 seconds 0 = No drift 1 = Drift; limb drifts down from position and does not hit bed or support in 10 sec 2 = Some effort against gravity 3 = No effort against gravity 4 = No movement Left |
Right Leg extended at 30 degrees, always tested supine for 5 seconds 0 = No drift 1 = Drift; limb drifts down from position and does not hit bed or support in 5 sec 2 = Some effort against gravity 3 = No effort against gravity 4 = No movement Left |
The finger-nose-finger and heel-shin tests 0 = Absent 1 = Present in one limb 2 = Present in two limbs |
8. Sensory | 9. Best Language | 10. Dysarthria |
To pinprick or noxious stimuli 0 = Normal 1 = Mild to moderate sensory loss 2 = Severe to total sensory loss |
0 = No aphasia, normal 1 = Mild to moderate aphasia 2 = Severe aphasia 3 = Mute, global aphasia, coma |
0 = Normal 1 = Mild to moderate 2 = Severe (including mute/anarthric due to aphasia) Do not score if intubated. |
11. Extinction and Inattention | ||
0 = No abnormality 1 = Present 2 = Profound (2 modalities) |
TOTAL SCORE: |
Ischemic strokes generally are classified as large artery atherosclerosis, small vessel occlusion, cardioembolism, stroke of other determined etiology, or stroke of undetermined etiology.5 In the first few minutes to hours after ischemic stroke, identification of stroke mechanisms may be difficult or impossible. Emergent diagnosis is enhanced significantly by imaging modalities, including computed tomography (CT) and magnetic resonance imaging (MRI).
Imaging of Acute Stroke
It is necessary to differentiate ischemic from hemorrhagic stroke before deciding on thrombolytic administration, and imaging obviously plays a key role in this regard. However, imaging may provide much more information. At most stroke centers, time from symptom onset (i.e., time when patient was last confirmed to be seen at normal baseline) is a major determining factor in whether a patient may be a candidate for IV thrombolysis (i.e., up to 3 hours) or intraarterial therapy (i.e., 3 to 6 hours). One emerging concept gaining more acceptance is that physiology rather than time should be used to decide on eligibility for treatment.6 For example, some patients within the 3-hour time window may already have established infarction that would not reverse with thrombolysis and may result in hemorrhage due to reperfusion of infarcted brain. Conversely, some patients may have salvageable brain tissue despite presentation well after the 3-hour time window. A physiologic estimate of tissue viability would be preferable to a fixed time interval if a study were found that reliably predicted viability of brain after stroke. CT and MRI have the potential to provide this measurement.7
Computed Tomography
A noncontrast head CT is the initial imaging modality of choice for patients with suspected stroke for two main reasons. The foremost is the expediency with which one can obtain a CT scan because of its widespread availability, and the second is the ability of CT to exclude intracranial hemorrhage. However, in addition to differentiating ischemic stroke from hemorrhage, CT may demonstrate subtle parenchymal abnormalities indicative of early edema or infarction. It was previously believed that these changes did not occur on CT for at least 6 hours after ischemic stroke. More recent studies indicate, however, that early changes of ischemia frequently occur within a few hours of stroke onset and have been seen as soon as 1 hour after stroke.8 These changes include reduced attenuation in the basal ganglia,2 loss of gray-white differentiation particularly in the insular region,9 low density in the cortex and subcortical white matter, and loss of sulcal markings, suggesting early mass effect and edema (Figure 34-1, A and B).10
A hyperdense middle cerebral artery occurs in 20% to 37% of cases,11 indicating acute thrombus within the artery. It rarely occurs without at least one other early CT abnormality. Hyperdensity in the basilar artery associated with thrombosis also has been reported.12 In 100 patients studied within 14 hours (mean 6.4 hours) of stroke onset, multiple early CT abnormalities correlated with size of subsequent infarct and poor outcome.11 In the ECASS I trial of tPA for acute stroke, early CT changes correlated with larger subsequent infarct volume and a greater likelihood of hemorrhagic conversion after tPA.13 Quantitative assessment of CT changes using the Alberta Stroke Program Early CT Score (ASPECTS) scale in patients treated with IV tPA also showed a relationship between early CT hypodensity (ASPECTS < 8) and hemorrhage (Table 34-2).14,15 Based on these results, some experts recommend withholding thrombolytic therapy in patients with extensive early CT changes, particularly in patients later in the thrombolytic time window,16 although this practice is somewhat controversial. For example, subsequent analysis of the NINDS rt-PA trial data discovered that early ischemic changes did not predict symptomatic hemorrhage or response to treatment,17 and more recent evidence reports no association between early ischemic CT changes and outcome.18
TABLE 34-2 ASPECTS Measurement Tool for Early Changes on Computed Tomography
10 Regions of Interest*: | |
---|---|
At the Level of the Basal Ganglia and Thalamus | At the Level Just Rostral to Deep Nuclei |
Anterior middle cerebral artery (MCA) cortex | Superior to anterior MCA cortex |
MCA cortex lateral to insula | Superior to MCA cortex lateral to insula |
Posterior MCA cortex | Superior to posterior MCA cortex |
Caudate | |
Lentiform nucleus Internal capsule Insular ribbon |
**1 point is subtracted for each defined area of early ischemic change, such as focal swelling or parenchymal hypoattenuation. Score varies from 0 to 10. |
* One point is subtracted for each defined area of early ischemic change, such as focal swelling or parenchymal hypoattenuation. Score varies from 0-10.
Adapted from Pexman JH, Barber PA, Hill MD, et al. Use of the Alberta Stroke Program Early CT Score (ASPECTS) for assessing CT scans in patients with acute stroke. AJNR Am J Neuroradiol. 2001;22(8):1534-1542.
Computed Tomography Angiography
CT angiography (CTA) can be performed using spiral CT technology, allowing for imaging of the intracranial and extracranial circulation. Optimally, CTA of the neck should include visualization of the aortic arch as well. The typical single bolus of iodine contrast material is approximately 70 mL of iodine. Owing to this injection, CTA is of limited use in patients with renal failure or contrast hypersensitivity. In acute stroke, CTA of the head and neck has been shown to be highly reliable for diagnosis of intracranial occlusions and correlates with other imaging modalities.19,20 Three-dimensional reconstruction images can also be created using this technology and can provide additional views and information about the carotid bifurcation and carotid lesions, showing eccentric lesions or ulceration not visualized by conventional angiography (see Figure 34-1, C and D).
Computed Tomography Perfusion
In addition to imaging the brain parenchyma with a noncontrast head CT and the cerebral vasculature with CTA, CT perfusion (CTP) adds assessment of cerebral blood volume (CBV) and cerebral blood flow (CBF). Using a helical scanner during a bolus of IV contrast, the time-dependent concentration curve of contrast in each pixel can be acquired. Mean transit time (MTT) and subsequently CBF can be calculated (Figure 34-2). In patients with acute stroke, CTP has been correlated with final infarct size and outcome, particularly after recanalization.21 CTP maps combining CBV and CBF information identify brain tissue that progresses to infarction if not reperfused, consistent with ischemic penumbra.22 Recent evidence suggests that the inclusion of CTP in a stroke imaging protocol increases diagnostic performance.21,23,24
Whereas CTP serves as a qualitative measure of blood flow, there have been recent investigations into using xenon CT as a quantitative measure of blood flow.25 Stable xenon is an inert gas inhaled as a mixture of 27% xenon and 73% oxygen. During inhalation over a few minutes, rapid scanning is performed and pixel-by-pixel blood flow values are calculated at different brain levels (Figure 34-3). In a series of patients with middle cerebral artery (MCA) occlusion studied with xenon CT, areas of penumbra were present in all patients, and the percentage of MCA territory in the penumbral range (i.e., cerebral blood flow 8 to 20 mL/100 g/min) remained relatively constant across the group. In contrast, the percentage of MCA territory with CBF values representing infarcted tissue (i.e., cerebral blood flow <8 mL/100 g/min) varied greatly. Outcome was highly correlated with the area of infarcted MCA territory, not the amount of ischemic penumbra. These results suggest that after the first few hours, the size of the core infarcted tissue, not the amount of penumbral tissue, may be the most important imaging parameter to determine suitability for acute stroke therapy.26
Magnetic Resonance Imaging
Compared to CT modalities, MRI brain imaging is advantageous because it is more sensitive to cerebral infarction, especially in the brainstem and deep white matter. Typical sequences included in a MRI stroke protocol include diffusion-weighted imaging (DWI) and apparent diffusion coefficient (ADC) to evaluate for potential acute ischemia, multiplanar gradient-recalled (MPGR) or gradient recalled echo (GRE) to evaluate for hemorrhage, and fluid attenuated inversion recovery (FLAIR) to evaluate for important signs in both hyperacute and acute stages of stroke (i.e., assessment for absence of flow void in major cerebral arteries, which suggests occlusion or slow flow in that artery). Perfusion-weighted imaging (PWI) is also a sequence often used to determine abnormal tissue perfusion based on transit times for contrast material through brain parenchyma (Figure 34-4).
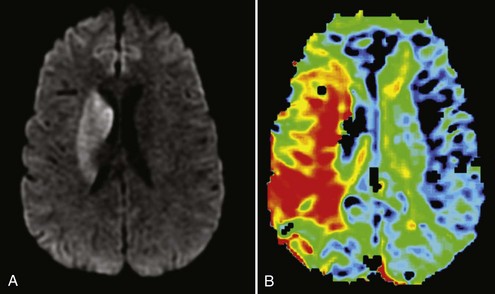
Figure 34-4 Magnetic resonance imaging of the same patient in Figure 34-2. A, Diffusion-weighted imaging (DWI) showing right basal ganglia stroke. B, Perfusion-weighted imaging (PWI) showing enhanced mean time to enhancement. These sequences together suggest a large ischemic penumbra in the right MCA territory.
DWI shows parenchymal abnormalities earlier than conventional T2-weighted images in patients with acute stroke.27 DWI detects the diffusion of water in the brain and shows hyperintensity in areas of reduced diffusion (see Figure 34-4). As water moves from the extracellular to the intracellular space, there is less movement of water and loss of signal, resulting in hyperintensity.28 DWI provides advantages in the evaluation of acute stroke. Early detection of lesions helps differentiate cerebral ischemia from other conditions that mimic stroke, such as seizures or toxic metabolic states. Additionally, combining DWI with PWI may identify reversibly ischemic tissue. If there is a large area of PWI abnormality indicating reduced blood flow but limited established infarction as evidenced by DWI abnormality, penumbral tissue is likely present, indicating areas of impaired flow at risk of undergoing infarction.
In stroke patients, the size of the DWI lesion and the growth of these abnormal DWI regions are strong predictors of outcome. In acute stroke, a marker of tissue viability is needed, and some investigators have suggested that the extent of mismatch between lesions on DWI and PWI could serve as this marker. The concept of DWI/PWI mismatch has been used as an inclusion criterion in several clinical trials (i.e., DIAS, DIAS-2, DEDAS, DEFUSE, EPITHET) assessing thrombolytic agents and is being employed more frequently to select patients that may ultimately benefit from reperfusion therapy.29–34 Patients with mismatch might be more likely to respond to reperfusion therapy.35 Patients with large areas of DWI abnormality or large severe PWI abnormalities may in fact be at greater risk for hemorrhage if reperfusion therapy is pursued.36
Magnetic Resonance Angiography
Magnetic resonance angiography (MRA) of the head and neck offers a noninvasive method of imaging the intracranial and extracranial vasculature. MRA typically uses gadolinium contrast in appropriate patients, but important information can be obtained based on time-of-flight techniques not utilizing contrast.37,38 Detection of dissection or occlusion in the circle of Willis and the extracranial vertebral and carotid arteries can be examined with MRA, but occlusions of small peripheral branch arteries may not be detected. Artifact may also wreak havoc in some cases by obscuring proper identification of arterial pathology. Signal dropout may occur at the site of arterial stenosis, owing to the effects of turbulent flow. If an artery is tortuous, it may extend out of the imaging section and appear occluded. MRI tends to overestimate the severity of stenosis, and evidence of severe stenosis should be confirmed with another modality. MRA is better for localizing the site of stenotic lesions than determining severity of stenosis. Similarly, differentiation between severe stenosis and occlusion is unreliable with MRI, and apparent occlusions by MRA should also be confirmed with angiography.
Treatment of Acute Stroke
Intravenous Thrombolysis
Acute stroke trials using IV thrombolytic agents date back to the early 1960s with use of streptokinase,39 fibrinolysin,40 and urokinase,41 showing either no benefit or a higher mortality in patients treated with thrombolysis. These studies preceded CT imaging, so patients with hemorrhage were not excluded. The discouraging results hindered the development of more acute stroke trials until the 1980s, when several case reports showed favorable outcomes with intraarterial thrombolytic therapy within a few hours of stroke onset.42,43 These reports resulted in small randomized trials and feasibility studies of IV thrombolytics44,45 which ultimately gave rise to the pivotal NINDS rt-PA trial that showed for the first time a beneficial effect of thrombolytic therapy for acute stroke treatment when administered within 3 hours of symptom onset.4
Tissue Plasminogen Activator Within 3 Hours
The NINDS trial included more than 600 patients with acute ischemic stroke. All patients were treated within 3 hours, and half of them were treated within 90 minutes. Patients were randomly assigned to receive either IV tPA at a dose of 0.9 mg/kg to a maximum of 90 mg, or IV placebo. Primary outcome measures were favorable outcomes at 90 days measured by the NIHSS, Barthel Index, Glasgow Outcome Scale, and modified Rankin Scale (mRS). By all four measures, significantly more patients had a favorable outcome at 90 days in the tPA group compared with placebo. Treatment with tPA resulted in an 11% to 13% absolute increase in good outcomes and a minor, non-significant decrease in mortality at 3 months. The benefit was sustained at 12 months.46 Intracerebral hemorrhage with clinical deterioration occurred in 6.4% of patients treated with tPA as compared to only 0.6% of placebo patients. Despite the increased hemorrhage rate, there was no significant increase in mortality or severe disability in the tPA group compared with placebo. When strokes were classified according to initial impression of stroke subtype, all types of strokes had more favorable outcomes with tPA. There were no clear factors that predicted response to tPA.47 Patients with large strokes as measured by NIHSS score higher than 20 and evidence of early low density or edema on CT had a higher rate of hemorrhage after tPA.48
On the strength of these results, in June 1996 the FDA approved IV tPA for treatment of stroke within 3 hours of onset. This recommendation was supported by the results of an analysis of patients treated within 3 hours of onset in the ATLANTIS trial.49 A subsequent pooled analysis of NINDS rt-PA, ECASS, and ATLANTIS data showed that clinical benefit with tPA is greatest when given early, especially if started within 90 minutes (Table 34-3).50 It was noted that not all patients recanalize with IV tPA. In a dose escalation trial of IV tPA, angiography was performed before thrombolysis in all patients, documenting the site of arterial occlusion and repeated 2 hours later. Proximal occlusions in the MCA opened less frequently than distal branch occlusions, and only 8% of carotid occlusions recanalized.51
Tissue Plasminogen Activator Beyond 3 Hours
Several subsequent tPA trials attempted to extend the window for treatment beyond 3 hours. The ECASS I and II trials and the ATLANTIS trial treated patients with IV tPA up to 6 hours after stroke onset but failed to show a significant benefit compared with placebo.52–54 Pooled analysis of NINDS rt-PA, ECASS, and ATLANTIS data suggested a potential benefit beyond 3 hours. The ECASS III trial recently revealed that IV alteplase administered between 3 and 4.5 hours after symptom onset significantly improved clinical outcomes in patients with acute ischemic stroke, thereby potentially extending the therapeutic window in which patients may receive IV tPA. In addition to standard IV tPA exclusion criteria (Table 34-4), ECASS III exclusion criteria includes combination of previous stroke and diabetes, NIHSS score greater than 25, oral anticoagulant treatment, or age older than 80 years.55 Whether patients in this time window with these exclusions also benefit from IV tPA is unknown.
TABLE 34-4 Inclusion and Exclusion Criteria for Intravenous tPA
Inclusion Criteria: |
CT, computed tomography; MCA, middle cerebral artery; NIH, National Institutes of Health; PT, prothrombin time; PTT, partial thromboplastin time; tPA, tissue plasminogen activator.
Other Thrombolytic Options
Desmoteplase (i.e., Desmodus rotundus salivary plasminogen activator) is a recombinant form of vampire bat saliva that is more potent than tPA. Desmoteplase possesses high fibrin selectivity, allowing it to dissolve a clot locally with less effect on the blood coagulation system. This property is thought to potentially reduce the risk of intracranial and systemic bleeding as compared to less fibrin-specific plasminogen activators like tPA. Desmoteplase was investigated in multiple trials to determine whether it could extend the treatment window for IV thrombolysis up to 9 hours.30,32 Unfortunately, no benefit of desmoteplase was realized between 3 and 9 hours after stroke symptom onset.31
Tenecteplase is a modified form of human tPA designed to achieve more effective thrombolysis. The half-life of tenecteplase is significantly longer, allowing administration as a single bolus. Similar to desmoteplase, tenecteplase has greater fibrin specificity and less fibrinogen depletion than tPA.56 A pilot safety study of tenecteplase for acute ischemic stroke was initiated but was recently discontinued due to slow enrollment; therefore no convincing conclusions at this time can be made about the promise of future study of tenecteplase in acute stroke.57,58
Reteplase is another recombinant form of human tPA that has been shown to be effective in the treatment of acute myocardial infarction.59 Reteplase also possesses a longer half-life compared to tPA, and a small case series found that in patients treated 9 hours after stroke onset with intraarterial reteplase, 88% completely recanalized and 44% achieved clinical improvement at 24 hours.60 Intraarterial reteplase has also been studied in conjunction with IV abciximab, a glycoprotein IIb/IIIa inhibitor, in a phase 1 study administering the combination therapy to stroke patients presenting between 3 and 6 hours.61 Abciximab may direct its effect through powerful antiplatelet effects or by direct thrombolysis. Abciximab monotherapy as emergent stroke treatment has also been evaluated in a phase 2 trial, with improved clinical outcome at 3 months in patients with mild to moderate strokes.62 Subsequently, a phase 3 trial was initiated but stopped prematurely due to an unfavorable benefit-risk profile.63
Sonothrombolysis is also currently being evaluated as an advantageous strategy for improving acute thrombolytic efficacy. The CLOTBUST trial indicated that continuous 2-MHz transcranial Doppler enhances tPA-induced arterial recanalization with a trend towards increased recovery from stroke.64 More recently, the TUCSON trial evaluated whether the addition of microspheres MRX-801 (ImaRx Therapeutics Inc., Round Rock, Texas) may further enhance the process of recanalization. Microspheres are a blend of phospholipids encapsulating a mixture of air and octafluoropropane gas (C3F8) that has the property of cavitation (i.e., rapid expansion and collapse) when exposed to ultrasound waves. The microspheres are administered IV, and when they reach intracranial occlusions, they transmit energy momentum from an ultrasound wave to residual flow and therefore promote recanalization. In TUCSON, it was concluded that microspheres could be safely combined with systemic tPA and ultrasound at a dose of 1.4 mL; however, there were safety concerns in the second dose tier of 2.8 mL that resulted in early termination of the trial. In both dose tiers, sonothrombolysis with microspheres and tPA showed a trend toward higher rates of early recanalization and clinical recovery compared to standard IV tPA therapy.65
Intraarterial Therapy
Intraarterial Thrombolysis
An alternative approach to IV thrombolysis is direct delivery of thrombolytic agents by a microcatheter embedded in the clot (Figure 34-5). The advantage of the intraarterial approach is direct visualization of the occluded artery and knowledge of the recanalization status as thrombolysis proceeds. Theoretically, delivery of the thrombolytic agent to the site of the clot should be more effective than IV infusion. The disadvantage is the additional time needed to bring the patient to the angiography suite, prepare the groin, catheterize the femoral artery, and guide the catheter from the femoral artery to the intracranial circulation before the thrombolytic agent can be administered.
Urokinase was used in early studies of intraarterial thrombolysis but is no longer available.66 Recombinant prourokinase was evaluated formally in clinical trials,67–69 and the PROACT II study was the first acute stroke trial to show a statistically significant improvement in clinical outcome when administered within 6 hours of stroke symptom onset. The median time to treatment was 5.5 hours, and most patients were treated after 5 hours.68