40 Malignant Hyperthermia
MH was first described by Denborough and Lovell in 1960, who reported a 21-year-old man with compound fractures of his right tibia, who, with great trepidation, underwent general anesthesia with halothane.1 After 10 minutes of anesthesia, he became hemodynamically unstable with hypotension, tachycardia, and mottled skin that was hot to the touch. The soda lime canister was found to be hot and was changed because it appeared to be exhausted. The anesthetic was discontinued, the patient was packed in ice, and he recovered without sequelae. Postprocedural examination did not reveal any known medical abnormalities. A careful family history disclosed that 10 blood relatives had previously died after ether anesthesia, suggesting an autosomal dominant pattern of inheritance for the disorder. This patient required a subsequent operation and was administered a spinal anesthetic without incident, demonstrating the effectiveness of nontriggering anesthetics.2 Subsequent reports from around the world established this disorder as a familial entity that was potentially fatal.3,4 The term malignant hyperpyrexia (later changed to malignant hyperthermia) was coined in 1967 at the first international meeting on this disorder.
The incidence of MH based on occurrences of MH reactions has been reported to be 1 case per 50,000 to 100,000 adults and 1 case per 3000 to 15,000 children.5,6 Subsequent surveys of suspected MH reactions revealed even greater incidences; the incidence of suspected MH was 1 case per 16,000 anesthetics in adults in Denmark, an incidence that increased to 1 case per 4200 adults when an inhaled anesthetic and succinylcholine were combined.7 However, a survey of anesthesia in a pediatric hospital in the United States during the halothane era revealed an MH incidence of 1 case per 20,000 to 40,000 children, almost one half of that reported previously.8 The incidence of fulminant MH (i.e., rapid increase in temperature accompanied by life-threatening metabolic changes, arrhythmias, and increased serum creatine kinase level) was 1 case per 250,000 general anesthetics in Denmark.7 A similar incidence of 1 case of fulminant MH per 200,000 general anesthetics was reported from the United Kingdom.9 The Danish survey found an incidence of 1 case of masseter muscle spasm per 12,000 anesthetics among children who received succinylcholine, whether in combination with inhalational or intravenous anesthetics.7 Clinically, the demographic data suggested that the incidence or suspicion of MH was greater among children than adults and that the incidence was even greater among children in whom succinylcholine was used.
The frequency of MH reactions is greatest in childhood, with a peak age of 3 years, although recent data suggest that only about 18% of MH reactions occur in childhood.10,11 Reactions have also been reported in neonates and infants, although they have been infrequent.12 Many have perceived a decrease in the incidence of MH reactions in the past 2 decades, although some have challenged this perception.11 Two reasons for the perception that the incidence of MH reactions have decreased are that many families with a genetic predisposition to MH have been identified and bring it to the attention of their surgical and anesthetic care providers preoperatively, and that the routine use of succinylcholine has decreased dramatically as a result of concerns regarding rare complications, such as hyperkalemic cardiac arrest. The latter has resulted in a black box warning admonishing against the routine use of succinylcholine in children, particularly male children younger than 8 years of age who may have unrecognized muscular dystrophy or other myopathy.13,14
It remains certain that all inhalational anesthetics and succinylcholine are potent triggers of MH reactions in susceptible patients,15–19 with the possible exception of xenon based on evidence from MH-sensitive pigs20 and caffeine-halothane contracture tests (CHCTs) from MH-susceptible and normal humans.21 No other drugs used for intravenous or regional anesthesia trigger MH reactions. A comprehensive list of triggering and nontriggering drugs is available from the Malignant Hyperthermia Association of the United States (MHAUS) (http://www.mhaus.org/ [accessed July 2012]).
The mortality rate for MH has decreased dramatically from more than 80% in the 1960s to 1.5% to 5% in recent years (Fig. 40-1).11,22–24 For children, the MH mortality rate (0.7%) is 15-fold less than the rate (14%) for adults.11 The overall decrease in mortality may be attributable to several factors: better identification of MH-susceptible individuals; the routine use of capnography and pulse oximetry, facilitating early identification of the signs and symptoms of an acute MH reaction25,26; a better understanding of the pathogenesis of MH; and the widespread availability of dantrolene.10
Clinical Presentation
The most common presentation of MH is a hypermetabolic response to the inhalational anesthetics, with or without succinylcholine (Table 40-1). Among the earliest clinical signs is a marked increase in the end-tidal CO2 that resists control by either mechanical ventilation or an increase in minute ventilation when the patient is breathing spontaneously.23,27 Other nonspecific early signs include tachycardia and hemodynamic instability with a trend toward hypertension. Severe masseter muscle spasm (i.e., masseter tetany) refers to the inability to insert a laryngoscope blade into the mouth, the so-called “jaws of steel,” even with no twitches evident on a blockade monitor after administration of succinylcholine, and it strongly indicates MH susceptibility (Fig. 40-2). In vitro live muscle biopsy testing revealed a 28% to 50% incidence of MH susceptibility among children with jaws of steel (Table 40-2).28–30 Generalized muscle rigidity develops as a result of the excessive accumulation of myoplasmic Ca2+ concentrations in MH-susceptible skeletal muscle, causing sustained muscle contractures.27 This occurs even in the presence of neuromuscular blockade with nondepolarizing neuromuscular blocking drugs (NMBDs).
TABLE 40-1 Clinical and Laboratory Findings Associated with Malignant Hyperthermia
Clinical Findings | Laboratory Findings |
---|---|
Tachycardia, tachypnea and hypertension | Increased Paco2 |
Hypercarbia (etco2) | Acidosis (mixed respiratory and metabolic) |
Greatly increased minute ventilation | Relative hypoxia, increased alveolar to arterial partial pressure gradient for oxygen |
Generalized muscle rigidity (unresponsive to nondepolarizing muscle relaxants) | Hyperkalemia |
Skin mottling | Elevated plasma lactate concentration |
Hyperthermia (late sign) | Abnormal coagulation studies (late sign) |
Cardiac arrhythmias (hyperkalemia-induced: PVC, VT, VF) | Myoglobinuria, myoglobinemia |
Cola-colored urine (late sign) | Increased CPK level (usually a late sign) |
Disseminated intravascular coagulation (late) |
CPK, Creatinine phosphokinase; etco2, end-tidal carbon dioxide; Paco2, arterial partial pressure of carbon dioxide; PVC, premature ventricular contraction; VF, ventricular fibrillation; VT, ventricular tachycardia.
Data from Malignant Hyperthermia Association of the United States. Available at http://www.mhaus.org (accessed July 2012).
TABLE 40-2 Limited Excursion of the Mandible: Differential Diagnosis
Temporomandibular joint dysfunction: congenital, inflammatory/infectious, trauma, neoplasm, collagen vascular disease (rheumatoid arthritis)
Muscle disease: malignant hyperthermia, Duchenne or Becker muscular dystrophy, myotonia congenita
Integumentary disease: inflammatory or infectious disease, neoplasm, radiation effects, collagen vascular disease (scleroderma)
Ultra-rapid metabolizer of succinylcholine (Nietlich or Cynthiana [C5 enzyme] variant of pseudocholinesterase) (see Chapter 6)
Hyperthermia, often a late sign, results from the greatly increased aerobic and anaerobic metabolic activity of triggered skeletal muscle; the overlying skin soon becomes hot to the touch. In many instances, the large muscle groups such as calf or thigh muscles feel tight or knotted. This is accompanied by exaggerated carbon dioxide (CO2) production, which usually is the first sign of an evolving MH reaction, and the accumulation of lactate fueled by markedly increased glycogenolysis and glycolysis (i.e., mixed respiratory and metabolic acidosis).31,32 If an acute MH reaction is diagnosed and treated early in its evolution, before the body becomes unable to maintain this exaggerated aerobic metabolism, arterial blood gases may show an almost pure respiratory acidosis, which can make the diagnosis of MH difficult (Table 40-3). A mixed venous or peripheral venous blood gas analysis can be helpful in establishing the presence of hypermetabolism because it is more likely to demonstrate an increased CO2 level, significant oxygen desaturation consistent with increased oxygen consumption ( less than 40 mm Hg, despite administering supplemental oxygen for which the expected
is greater than 60 mm Hg), and a possible increased lactate concentration.
In fulminant cases, untreated MH may increase the temperature as rapidly as 1° C every 10 minutes.27 In one case, the temperature reached 43.8° C (110.8° F) within 18 minutes.33 In addition to the profound hypercarbia and tachycardia, severe hypoxia, skin mottling, exuberant metabolic acidosis, rhabdomyolysis, coagulopathy, and hyperkalemia may follow. Unstable hemodynamics and ventricular arrhythmias inevitably follow. Intractable ventricular arrhythmias, pulmonary edema, disseminated intravascular coagulation, cerebral hypoxia or edema, and renal failure due to myoglobin deposition in the renal tubules are often associated with fatal outcomes.
The presenting signs of MH vary (see Table 40-1). The syndrome may be fulminant or indolent, not all features of a classic MH reaction may be immediately evident, and it may occur intraoperatively or postoperatively, although the frequency of MH reactions occurring postoperatively is only 2%.27,34 The latest that an MH reaction has reportedly occurred postoperatively is 11 hours, although a recent review of reports of postoperative MH in the North American Malignant Hyperthermia Registry has not found any case occurring beyond 40 minutes after the end of the anesthetic.34,35 The likelihood that an MH-susceptible patient will develop MH in the presence of inhalational anesthetics is exasperatingly unpredictable. In one study, 50% of susceptible individuals reported two or more uneventful general anesthesias before an MH reaction was triggered.23,36 Only 6.5% of probands in a retrospective review reported a family or personal history of MH.36 A negative personal or family history is insufficient to conclude that a child is not susceptible to MH. Other disease states may be confused with MH (Table 40-4) and must be distinguished from it to provide correct therapy.
TABLE 40-4 Differential Diagnosis of Malignant Hyperthermia
Diagnosis | Distinguishing Traits |
---|---|
Hyperthyroidism | Patients often present with similar symptoms and physical findings; blood gas abnormalities gradually evolve; creatine phosphokinase value does not increase substantively. |
Sepsis | Usually, blood gases are normal early, and metabolic acidosis occurs late; creatine phosphokinase remains normal. |
Pheochromocytoma | Similar to MH, except for marked blood pressure swings |
Metastatic carcinoid | Flushing, diarrhea, hypotension |
Cocaine intoxication | Fever, rigidity, rhabdomyolysis similar to NMS |
Heat stroke | Similar to MH, except that the patient is outside the operating room |
Masseter muscle rigidity (MMR) | May progress to MH; total body spasm more likely than isolated MMR |
Neuroleptic malignant syndrome (NMS) | Similar to MH but evolves over weeks; usually associated with the use of antipsychotics |
Serotonergic toxicity | Similar to MH and NMS; associated with the administration of mood-elevating drugs (e.g., selective serotonin reuptake inhibitors) |
Nonmalignant hyperthermia syndrome | Reported only once; severe hyperthermia seemingly associated with fentanyl |
Given the variability of the clinical presentation of MH and the dearth of pathognomonic signs for this syndrome, establishing the diagnosis can be difficult. In response to the need for an objective measure to verify a clinical episode of MH, a retrospective, multivariable clinical grading scale was developed.37 This grading scale was devised to clarify the cutoff value for a positive muscle CHCT result. It was not intended to be a clinical guide in the operating room. Despite recommendations not to use this scale to guide treatment and to be more conservative in its application, Tables 40-5 and 40-6 are provided to help clinicians identify true MH reactions. Although this clinical grading scale is somewhat cumbersome and has not been prospectively validated in clinical settings for use by nonexperts, it is a useful guide for the clinician.
TABLE 40-5 Clinical Indicators for Determining the Malignant Hyperthermia Raw Score
Process | Indicator | Points |
---|---|---|
Rigidity | Generalized muscular rigidity | 15 |
Masseter spasm | 15 | |
Muscle breakdown | Creatine kinase >20,000 IU after succinylcholine | 15 |
Creatine kinase >10,000 IU with no succinylcholine | 15 | |
Cola-colored urine in perioperative period | 10 | |
Myoglobin in urine >60 µg/L | 5 | |
Myoglobin in serum >170 µg/L | 5 | |
Blood, plasma, or serum K+ >6 mEq/L, no renal illness | 3 | |
Respiratory acidosis | Petco2 >55 mm Hg with controlled ventilation | 15 |
Arterial Paco2 >60 mm Hg with controlled ventilation | 15 | |
Petco2 >60 mm Hg with spontaneous ventilation | 15 | |
Arterial Paco2 >65 mm Hg with spontaneous ventilation | 15 | |
Inappropriate hypercarbia, anesthesiologist’s call | 15 | |
Inappropriate tachypnea | 10 | |
Temperature increase | Inappropriately rapid increase | 15 |
Inappropriately increased temperature >38.8° C (101.8° F) | 10 | |
Cardiac involvement | Inappropriate sinus tachycardia | 3 |
Ventricular tachycardia or fibrillation | 3 | |
Family history | Positive family history for first-degree relative | 15 |
Positive family history for more distant relative | 5 | |
Others | Arterial base excess more negative than −8 mEq/L | 10 |
Arterial pH <7.25 | 10 | |
Rapid reversal of maligant hyperthermia (MH) signs after intravenous administration of dantrolene | 5 | |
Positive MH family history with another indicator from the patient’s anesthesia experience other than increased creatine kinase level | 10 | |
Elevated creatine kinase level and a family history of MH | 10 |
From Larach MG, Localio AR, Allen GC, et al. A clinical grading scale to predict malignant hyperthermia susceptibility. Anesthesiology 1994;80:771-9.
TABLE 40-6 Malignant Hyperthermia Clinical Grading Scale
Raw Score Range | Rank | Likelihood |
---|---|---|
0 | 1 | Almost never |
3-9 | 2 | Unlikely |
10-19 | 3 | Somewhat less than likely |
20-34 | 4 | Somewhat greater than likely |
35-49 | 5 | Very likely |
≥50 | 6 | Almost certain |
Modified from Larach MG, Localio AR, Allen GC, et al. A clinical grading scale to predict malignant hyperthermia susceptibility. Anesthesiology 1994;80:771-9.
Patient Evaluation and Preparation
Ambulatory surgery has rapidly expanded to include most pediatric surgery. Consequently, the safety of discharging children with a personal or family history of MH after an uneventful, trigger-free anesthesia on the day of surgery has raised concerns. Two retrospective studies concluded that the risk of a child developing an MH reaction after an uneventful, trigger-free anesthesia was exceedingly small.38 Postoperative monitoring for an MH reaction while in the hospital was gradually reduced from 6 hours to 2 hours before discharge.39,40 For MH-susceptible children, parents should be provided with a written description of the signs and symptoms of an MH reaction and a phone number for the on-call anesthesiologist for further advice. Families should contact an anesthesiologist rather than return to the emergency department, because the emergency physician may be unfamiliar with MH, particularly in children. Additional advice for the parents should include the use of an oral antipyretic drug (e.g., acetaminophen) to treat a mild fever. If the fever abates after a dose or two of acetaminophen, the fever was not caused by MH. If the fever persists despite acetaminophen and sponge baths, and is accompanied by tachycardia and tachypnea, the parents should notify the on-call anesthesiologist and return the child to the hospital.
When a child with a known susceptibility to MH is scheduled for general anesthesia, the anesthesia machine (i.e., anesthetic workstation [AWS]) must be prepared to preclude the delivery of triggering agents. First, succinylcholine should be removed from the local vicinity to avoid inadvertent administration. Second, all vaporizers should be physically disengaged from the AWS, which is preferable because they can leak trace concentrations of inhalational anesthetics even in the off state, or if they cannot be removed from the AWS, tape should be placed across them in the off position to avoid accidentally turning them on.41 Third, to accelerate the washout of anesthetics, the CO2 absorbent should be replaced, a new anesthetic breathing circuit installed, and the ventilator bellows flushed and left operating.41–43 Fourth, to eliminate inhalational anesthetics from the AWS, many clinicians follow a standardized protocol of flushing the workstation with 10 L/min of oxygen for 10 to 20 minutes, depending on the manufacturer and age of the machine.44 However, the assumption that one protocol fits all to reduce the anesthetic concentration to less than 10 ppm, which is assumed to be the threshold below which an MH reaction cannot be triggered,45 may not hold true for every AWS, particularly the newer ones, which are more complex in construction and more likely to contain internal working parts made of plastic, which act as sumps for inhalational anesthetics. To address the various types of AWS, the duration of flushing with large fresh gas flows (Table 40-7) and the need to exchange contaminated internal components with clean versions must be determined for each AWS. For some types of AWS, more than 60 minutes may be required to reach anesthetic concentrations less than 10 ppm.44,46,47 To achieve an anesthetic concentration of 10 ppm or less in the Drager Primus, Fabius, and Zeus machines in a timely manner, the ventilator diaphragm and integrated breathing system should be replaced with autoclaved components and then flushed for 20 minutes at a fresh gas flow of 10 L/min.46,47 Table 40-7 lists the published times required to reach an anesthetic concentration of less than 10 ppm without replacing any AWS components.44,47,48 These data support the notion that the previously held protocols to wash out inhalational anesthetics from older AWSs do not hold true for the newer AWSs. The need for a single protocol or intervention that consistently achieves an anesthetic concentration of less than 10 ppm in all AWSs is of even greater importance because none of the available anesthetic agent analyzers is capable of measuring anesthetic concentrations in the 10 ppm range to confirm adequate removal of inhalational anesthetics.
TABLE 40-7 Time to Wash Out Inhalational Anesthetics to Less than 10 ppm from Anesthetic Workstations
After the AWS has been flushed with 10 L/min of an air and oxygen mixture, most anesthesiologists reduce the fresh gas flow during anesthesia. However, evidence has shown that the concentration of inhalational anesthetic surges (≥50 ppm) when the fresh gas flow is reduced, and the magnitude of the rebound directly depends on the fresh gas flow rate.48,49 Those who reduce the fresh gas flow after purging the AWS may be exposing their patients to concentrations of inhalational anesthetics that may trigger an MH reaction, although no MH reactions have been reported in patients in whom a reduced fresh gas flow was used in an AWS that had been purged using a large (>10 lpm) fresh gas flow. To avoid confusion, a single, consistent, effective, and reliable intervention is required to prevent MH reactions in patients exposed to trace gases from a previously contaminated AWS.
A commercially available charcoal filter (Vapor-Clean, Dynasthetics, LLC, Salt Lake City) fitted to the expiratory and inspiratory limbs of the AWS reduces the concentration of inhalational anesthetics to less than 5 ppm within several minutes.50 These filters are sold in pairs. The manufacturer recommends that a filter should be inserted into both limbs of the anesthesia breathing circuit just distal to the valves, which is reasonable during an acute MH reaction. However, when only the machine is contaminated with inhalational anesthetic (e.g., after flushing the AWS for elective MH cases), we apply a single filter in the inspiratory limb and keep the second of the pair to replace the first after 60 to 90 minutes, since it may become expended by that time.50
Monitoring
Capnography and pulse oximetry, key monitors for early signs of an MH reaction, are required for all children who receive general anesthesia, irrespective of the duration of the procedure. Measurement of the body temperature is recommended for all children who undergo general anesthesia when fluctuations may be anticipated, according to the American Society of Anesthesiologists 2011 standards for basic anesthesia monitoring (www.asahq.org/For-Members/Standards-Guidelines-and-Statements.aspx). Monitoring the axillary temperature site (opposite the extremity with the intravenous line) is recommended rather than a core site for early detection of an MH reaction because the axillary region is surrounded by large muscle bulk in the pectoral shoulder girdle. Although most consider an increasing temperature a late sign of an MH reaction, a retrospective review suggested that an increasing body temperature may occur early in an evolving MH reaction.36,51 Evidence also suggests that crystalline skin temperature tapes may not reliably track temperature changes during MH reactions.36
Diagnosis
Because the underlying disorder in MH is a hypermetabolic reaction (i.e., increased CO2 production and oxygen consumption), massive volumes of CO2 are released into the circulation, which rapidly increase the partial pressure of CO2 (Pco2) and respiratory rate in the unparalyzed child. The cardiovascular response is an increased cardiac output, heart rate, and in some cases, blood pressure (E-Fig. 40-1). The first clinical signs and symptoms of this hypermetabolic reaction in a spontaneously breathing patient are hypercapnia, tachypnea, and tachycardia (see Table 40-1).36 A steady and relentless increase in the end-tidal CO2 pressure (Petco2) is the earliest sign of a reaction and is usually evident whether respirations are spontaneous or controlled. In some instances, the increase in Petco2 and heart rate occur contemporaneously, alerting the clinician to consider an evolving MH reaction (Fig. 40-3
). Sudden unexpected cardiac arrest is a very rare presentation of MH and suggests a disease process other than MH, such as acute rhabdomyolysis and hyperkalemia after succinylcholine in a (male) child with an undiagnosed myopathy.
During airway obstruction and hypermetabolic states such as thyrotoxicosis and sepsis, an increased Petco2 can be readily corrected with mild to moderate hyperventilation. During MH reactions, however, it is very difficult to restore the Petco2 to the normal range, even with vigorous mechanical hyperventilation.26 The CO2 production is sometimes so great that the in-circuit CO2 absorbent rapidly becomes exhausted in an exothermic reaction, and the absorbent container becomes hot to touch.
A child’s response to surgery during light anesthesia often includes tachycardia and may sometimes include bronchoconstriction. However, a dramatic and unexpected increase in heart rate from 120 to 180 beats/min in a healthy, 7-year-old child (or an increase from 70 to 120 beats/min in an adult) strongly suggests a pathologic process, and a differential diagnosis beyond light anesthesia should be seriously considered. Before intervening, it is important to quickly scan all of the monitors to determine whether the aggregate indices point to a specific diagnosis. If tachycardia is associated with an increase in body temperature, a differential diagnosis of fever and tachycardia under anesthesia should be considered. Fever related to sepsis or viral infection usually has a slow onset, whereas fever from an MH reaction typically has a rapid onset. The differential diagnoses include iatrogenic external overheating and an MH reaction (see Table 40-4). A rapid increase in the inspired concentration of desflurane and isoflurane, but not sevoflurane, may cause a sympathetic-based tachycardia that in isolation should not suggest a diagnosis of MH because the Petco2 remains unchanged.52,53 Of the inhalational anesthetics, halothane appears to be the most likely to trigger an MH reaction and the best discriminator for the CHCT. Enflurane provides the smallest trigger, sevoflurane and isoflurane are intermediate, and preliminary data suggest that xenon does not trigger MH reactions.21,45,54–56 If none of these factors appears to be causative and a deeper level of anesthesia (achieved with propofol with or without an opioid) fails to abate the signs, simultaneous venous and arterial blood gases should be analyzed to determine whether the patient has or is developing a hypermetabolic state.
A moderate but gradual increase in body temperature may occur in children excessively draped, those with forced-air warming devices, those with bilateral limb tourniquets, and those covered with plastic occlusive wrap. However, the sudden onset of a high fever must be more thoroughly investigated because it may result from several potentially fatal causes (see Table 40-4).57–60
Management, Susceptibility Screening, and Counseling
Treatment
If the anesthesiologist suspects that a child is experiencing an MH episode, the inhalational anesthetic should be immediately discontinued, 100% oxygen administered at a large fresh gas flow rate (≥10 L/min), and the surgeon informed; if surgery cannot be aborted, it must be completed expeditiously. Charcoal filters should be inserted into both limbs of the breathing circuit until a clean breathing circuit is available (E-Fig. 40-2). They prevent the child from being contaminated by residual anesthetic in the AWS and to prevent the AWS from being contaminated by anesthetic in the patient.50 The MH cart and additional personnel to assist in dissolving the dantrolene should be brought to the operating room immediately (Table 40-8). Minute ventilation should be increased to control the Paco2 and Petco2.
Because native dantrolene is quite insoluble in water, several strategies have been developed to speed its solubility. The current formulation is packaged as a lyophilized yellow powder in 20-mg vials that contain 3 g of mannitol and enough base to maintain a pH of about 9.5 (E-Fig. 40-3). The lyophilized formulation, mannitol, and the alkaline pH all speed the dissolution of dantrolene in water. Sixty milliliters of sterile water should be added to each vial to dissolve the dantrolene, with a resultant dantrolene concentration of 0.33 mg/mL. Warming the sterile water speeds dissolution.61 Most of the dantrolene in a vial dissolves within 60 seconds of adding the water. When the vial is vigorously shaken, any residual crystals dissolve, and the solution turns clear orange. The solution should be withdrawn immediately and administered intravenously as rapidly as possible. Occasionally, 1 or 2 additional minutes may be required to dissolve the last few crystals of dantrolene. Given the extreme alkaline pH of the dantrolene solution, it should be rapidly infused into a large vein to reduce the risk of phlebitis. Extravasation of dantrolene or prolonged continuous infusions of dantrolene may cause thrombophlebitis or thrombosis of large and small veins.62–64 Some have recommended continuous infusions of dantrolene in adults after the initial bolus, although the risk/benefit ratio of this practice is unproved in adults and untested in children.65 A new formulation of nanocrystalline dantrolene that rapidly dissolves and is available in a much greater concentration than the current formulation is undergoing federal review.66
The pharmacokinetics of intravenous dantrolene have been studied in MH-susceptible children 2 to 7 years of age.67 A loading dose of 2.5 mg/kg produced predictable blood concentrations (≥3 µg/mL) for about 6 hours after the loading dose (Fig. 40-4).67 Based on these pharmacokinetic data, if half of the loading dose of dantrolene were repeated at 6 hours after the loading dose, therapeutic blood concentrations of dantrolene would be maintained for a total of 15 hours and possibly prevent a recrudescence.67
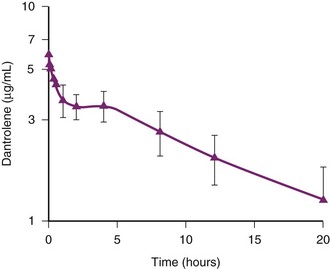
FIGURE 40-4 Pharmacokinetics of dantrolene in children.
(From Lerman J, McLeod ME, Strong HA. Pharmacokinetics of intravenous dantrolene in children. Anesthesiology 1989;70:625-9.)
An initial bolus dose of 2.5 mg/kg can control most MH reactions if the dantrolene is administered as soon after the onset of the reaction as possible (see Fig. 40-3).68 Delay in instituting dantrolene therapy increases the probability of failed therapy and death.36 For a 70-kg patient, this corresponds to 8 vials of dantrolene. The response to dantrolene should be evident within minutes, with a marked reduction in Petco2, heart rate, and respiratory rate (see Fig. 40-3). If there is no response within 3 to 5 minutes, the initial dose should be repeated until signs that the physiologic variables are abating. The clinical end points include resolution of the hypercapnia, tachypnea, and tachycardia; resolution of muscle rigidity; restoration of clear urine output; return of normal consciousness when sedation is discontinued; self-correction of blood gas abnormalities; and resolution of electrolyte disturbances. If these do not occur or are incomplete, additional doses of dantrolene should be administered until all signs and symptoms of the MH reaction have abated or the diagnosis reevaluated.68 There is no upper limit to the amount of dantrolene that can or should be given acutely to stop an MH reaction. In rare instances of persistent MH or recrudescence, a cumulative dose of up to 40 mg/kg has been required.69 Dantrolene is a fairly potent muscle relaxant, and a child with an unintubated airway (particularly one with respiratory disorders) may become weak and require controlled respirations.
Although dantrolene is effective in terminating acute MH reactions, this effect may wane as the blood concentration of dantrolene decreases. In this case, the MH reaction may recrudesce. The prevalence of recrudescence has been reported to be as great as 20% and associated with several predictive factors, including muscular body type and a greater time interval between induction of anesthesia and the development of the initial reaction.70 However, the first episode of recrudescence may occur any time after the initial reaction was successfully treated, up to and as late as 36 hours.71 The possibility of recrudescence must always be considered during the first 2 to 3 days after an MH reaction has occurred. Because recrudescence cannot be predicted, all children who experience an MH reaction must be admitted to the pediatric intensive care unit or a monitored bed until the reaction resolves and the child’s metabolic indices return to and remain normal for 2 to 3 days. MHAUS recommends that 1 mg/kg of dantrolene be administered intravenously every 6 hours for 24 to 48 hours after an MH reaction to prevent recrudescence. This recommendation remains empirical because there are no data to support the effectiveness of dantrolene in preventing recrudescence, although these dosages are consistent with pharmacokinetic data in children.67 We recommend continued vigilance, frequent physical examinations for muscle tightness, and repeated laboratory tests (e.g., blood gas analyses), and monitoring of vital signs, particularly heart rate and expired CO2 tension for evidence of a recrudescence during the first 48 hours after an MH episode. If recrudescence does occur, additional intravenous dantrolene should be administered until the reaction again abates.
Acute administration of dantrolene causes skeletal muscle weakness that can lead to respiratory embarrassment. Not surprisingly, neostigmine is ineffective in reversing the effects of dantrolene because the latter acts intracellularly, not at the neuromuscular junction (see the section on molecular mechanisms of dantrolene). It may be advisable to maintain control of the airway (combined with intravenous sedation) until there is no further need for dantrolene. Preoperative, orally administered dantrolene, initially recommended in MH-susceptible children 2 decades ago, caused skeletal muscle weakness, dysarthria, sialorrhea, and diplopia and was ineffective in preventing MH.72 As a result, this practice has been discontinued.72 There have been no reports of acute dantrolene toxicity, although reported adverse effects after intravenous administration include muscle weakness (about 22%), phlebitis (9%), gastrointestinal upset (about 4%), and respiratory failure (3.8%).63 Long-term use in the treatment of chronic skeletal muscle spasticity has been reported to cause liver dysfunction and fatal hepatitis in about 1% and 0.1% of patients, respectively.73 Given the risks of an MH reaction, there seems little downside to treating a child with dantrolene before the diagnosis is certain, provided all the necessary blood work and laboratory tests have been collected, because early treatment reduces mortality and morbidity (see Fig. 40-1).36 The probability of developing a complication from an MH reaction increases almost threefold for every 2° C increase in body temperature and 1.6-fold for every 30-minute delay in administering intravenous dantrolene during a reaction.36 Cardiac arrest and death during an MH reaction correlate with a muscular physique and greater time intervals between induction of anesthesia and the maximal value of Petco2.24 Other possible diagnoses should continue to be considered while the treatment for the suspected MH reaction is organized (see Table 40-4). A positive response to dantrolene is not pathognomonic of an MH reaction, because other conditions may respond with resolution of their signs.