CHAPTER 101 Maldigestion and Malabsorption
Malabsorption can be caused by many diseases of the small intestine and also by diseases of the pancreas, liver, biliary tract, and stomach (Table 101-1). Whereas in some of these diseases, malabsorption may be the presenting feature, in others malabsorption may be only a minor clinical problem or may be detected only as a laboratory abnormality.
Table 101-1 Diseases That Cause Nutrient Malabsorption
Gastric Diseases |
Pancreatic Diseases |
Liver Diseases |
Obstructive Biliary Diseases |
Intestinal Diseases |
Lymphatic Diseases |
Neuroendocrine Tumors |
Cardiac and Vascular Diseases |
Endocrine Causes |
Systemic Diseases |
AIDS, acquired immunodeficiency syndrome; HIV, human immunodeficiency virus.
ETIOLOGY AND PATHOPHYSIOLOGY
Normal uptake of nutrients, vitamins, and minerals by the gastrointestinal tract requires several steps, each of which can be compromised in disease. (Normal digestion and absorption are discussed in Chapter 100.)
Digestion of macromolecular compounds, such as polysaccharides, triglycerides, and proteins, to their molecular components—monosaccharides, fatty acids, and amino acids, respectively—is achieved by soluble or membrane-bound digestive enzymes. Absorption of undigested or partially digested macromolecular compounds occurs to a very minor degree in health and may be increased slightly in various intestinal diseases. Although such absorption does not play a nutritive role, it may be important for the normal function of the immune system and for the pathogenesis of diseases such as food allergy (see Chapter 9).
Chemical changes to nutrients may be required for absorption, such as changing the charge of iron.
Intestinal sensory and motor function permits detection of the presence of nutrients, facilitates adequate mixing of nutrients with intestinal secretions and delivery to absorptive sites, and provides adequate time for nutrient absorption (see Chapter 97).
An overview of pathophysiologic mechanisms of maldigestion and malabsorption is provided in Table 101-2. This table also shows the ingested substrates primarily affected by the individual pathophysiologic mechanisms and lists examples of etiologic disorders for these mechanisms.
Table 101-2 Mechanisms of Malabsorption, Malabsorbed Substrates, and Representative Causes
MECHANISM | MALABSORBED SUBSTRATE(S) | REPRESENTATIVE CAUSES |
---|---|---|
Maldigestion | ||
Conjugated bile acid deficiency |
CCK, cholecystokinin.
FATS
DEFECTIVE MIXING
For sufficient digestion and absorption of lipids, dietary fat must adequately mix with digestive secretions. Gastric resections or gastrointestinal motility disorders that result in rapid gastric emptying or rapid intestinal transit, such as autonomic neuropathy resulting from diabetes mellitus or amyloidosis, can cause fat malabsorption consequent to impaired gastrointestinal mixing of dietary fat.1
REDUCED SOLUBILIZATION OF FAT
Fat malabsorption due to decreased formation of micelles occurs if the luminal concentrations of conjugated bile acids are lower than the critical concentration required for forming micelles.2,3 Table 101-31,4 details the pathophysiologic mechanisms and representative diseases that cause luminal bile acid deficiency.
Table 101-3 Pathophysiologic Mechanisms That Result in Deficiency of Luminal Conjugated Bile Acids
PATHOPHYSIOLOGIC MECHANISM | CAUSES |
---|---|
Decreased synthesis and/or secretion of conjugated bile acids |
CCK, cholecystokinin.
DECREASED LIPOLYSIS
If exocrine pancreatic function is severely reduced, impairment of pancreatic lipase and colipase secretion results in decreased luminal hydrolysis of dietary fat.5 Chronic pancreatitis, cystic fibrosis, pancreatic duct obstruction by pancreatic and ampullary tumors, and pancreatic resection are the most common causes of pancreatic insufficiency.1 Even when pancreatic enzyme concentrations are normal, reduced pancreatic lipase activity due to a low luminal pH,6 excessive calcium ingestion,7 or ingestion of the specific lipase inhibitor orlistat8 can cause pancreatic steatorrhea. Selective congenital lipase or colipase deficiency is a rare cause of pancreatic fat malabsorption.9
DECREASED MUCOSAL ABSORPTION AND CHYLOMICRON FORMATION
Generalized mucosal diseases, such as celiac disease or tropical sprue, often are associated with fat malabsorption. Defective uptake of free fatty acids and monoglycerides results from reduced mucosal surface area because of villus shortening, reduced enterocyte function, and mucosal inflammation.1 Intestinal fat absorption also is impaired in diseases that result in disturbance of intracellular formation of chylomicrons and accumulation of lipids within the enterocytes, including abetalipoproteinemia, hypobetalipoproteinemia, and chylomicron retention disease.10
DEFECTIVE LYMPHATIC TRANSPORT OF CHYLOMICRONS
Impairment of lymphatic transport of chylomicrons is a cause for postmucosal malabsorption of dietary fat. Decreased lymphatic transport can result from congenital diseases such as primary intestinal lymphangiectasia or from obstruction of lymphatic vessels due to metastatic solid tumors, lymphoma, Whipple’s disease, retroperitoneal fibrosis, or trauma6 (see Chapter 28). Usually, lymphatic vessels in the mucosa become dilated (lymphangiectasia), and chylomicrons are lost into the intestinal lumen postprandially and also in the fasting state11; steatorrhea in these situations usually is only mild to moderate.10
PROTEINS AND AMINO ACIDS
Defective digestion or absorption of dietary proteins has to be differentiated from excessive loss of serum proteins into the gastrointestinal tract, which is termed protein-losing enteropathy (see Chapter 28).
DEFECTIVE INTRALUMINAL PROTEOLYSIS
Protein digestion may be impaired in patients who have undergone partial or total gastric resection, presumably as a result of poor mixing with digestive secretions, although gastric pepsin deficiency could be contributory. Defective proteolysis also occurs with exocrine pancreatic insufficiency.1,12,13 In congenital diseases, pancreatic proteolysis can be impaired by inborn errors in the synthesis of proteolytic enzymes (trypsinogen deficiency)13 or by defective activation of pancreatic proenzymes resulting from congenital deficiency of intestinal enterokinase (see later).14
DEFECTIVE MUCOSAL HYDROLYSIS OF PEPTIDES AND DECREASED ABSORPTION OF OLIGOPEPTIDES AND AMINO ACIDS
Generalized mucosal diseases, such as celiac disease and tropical sprue, result in global malabsorption, which includes malabsorption of oligopeptides and amino acids due to lack of mucosal hydrolysis of oligopeptides and defective mucosal absorption.13 Reduction of intestinal absorptive surface, as in short bowel syndrome or jejunoileal bypass, also results in protein and amino acid malabsorption.13,15 Congenital defects of amino acid transporters on the enterocytes, such as Hartnup’s disease and lysinuric protein intolerance, can lead to selective malabsorption of a subgroup of amino acids (see later on).
CARBOHYDRATES
DEFECTIVE INTRALUMINAL HYDROLYSIS OF CARBOHYDRATES
Pancreatic α-amylase normally is secreted in excess into the intestinal lumen. In mild forms of pancreatic insufficiency, carbohydrate digestion usually is at least partially preserved,16 but severe pancreatic insufficiency results in clinically apparent carbohydrate malabsorption and diarrhea due to decreased luminal hydrolysis of ingested starch.17
MUCOSAL DEFECTS OF CARBOHYDRATE DIGESTION AND ABSORPTION
The most common cause of carbohydrate malabsorption is late-onset lactose malabsorption due to decreased levels of the intestinal brush border enzyme lactase (adult-type hypolactasia, acquired primary lactase deficiency). Depending on ethnic background, lactase is present in less than 5% to more than 90% of the adult population; its deficiency results in a selective malabsorption of lactose. Acquired malabsorption of carbohydrates occurs commonly after extensive intestinal resections, in diffuse mucosal diseases such as celiac disease or Crohn’s disease, or temporarily after self-limited gastrointestinal infections (postinfection carbohydrate malabsorption).16,17 The pathophysiologic mechanisms of carbohydrate malabsorption are reduction of the intestinal mucosal surface area and a reduced activity or expression of intestinal oligo- and disaccharidases or transport proteins for monosaccharides.16 Congenital disaccharidase deficiencies (lactase, sucrase-isomaltase, and trehalase)18 and congenital deficiency or malfunction of transport molecules as in congenital glucose-galactose malabsorption19 can cause early onset of malabsorption of mono- or disaccharides (see later on). Intolerance of fructose is discussed in a subsequent section.
VITAMINS
FAT-SOLUBLE VITAMINS
Diseases causing malabsorption of dietary fat commonly cause malabsorption of fat-soluble vitamins, because they require similar absorptive mechanisms. This is especially important in diseases that result in impaired micelle formation from bile salt deficiency.20 Fat-soluble vitamins also are malabsorbed in diffuse diseases of the mucosal surface area, in diseases affecting chylomicron formation and transport,21 and in exocrine pancreatic insufficiency.22 Some authors have suggested that absorption of fat-soluble vitamins is less affected by exocrine pancreatic insufficiency than by small intestinal diseases resulting in steatorrhea.23
WATER-SOLUBLE VITAMINS
Vitamin B12 (Cobalamin)
Decreased release of dietary vitamin B12 from food sources because of impaired pepsin and acid secretion, as in atrophic gastritis24 or use of acid inhibitory drugs such as proton pump inhibitors,25 usually results in only mild cobalamin malabsorption without clinical consequences. By contrast, deficiency of gastric intrinsic factor secretion, as occurs in pernicious anemia or after gastric resections, or secretion of an abnormal intrinsic factor, as in some congenital diseases, results in severe vitamin B12 malabsorption with clinical consequences.24
Autoimmune gastritis of pernicious anemia is the most common cause of vitamin B12 malabsorption.26 Cobalamin malabsorption in pernicious anemia is caused by decreased intrinsic factor secretion resulting from parietal cell destruction and by blocking autoantibodies that inhibit intrinsic factor binding to vitamin B12.26 Mild cobalamin malabsorption may be found in patients with pancreatic insufficiency and in patients with Zollinger-Ellison syndrome, owing to decreased proteolytic release of vitamin B12 from its complex with R-binding protein24,27 (see Chapters 32, 59 and 100).
In bacterial overgrowth syndrome (see Chapter 102) or helminthic infection with Diphyllobothrium latum (see Chapter 110), dietary cobalamin is made unavailable to the host or is consumed by the microorganisms or parasites in the intestinal lumen and, therefore, is not available for intestinal absorption.26
Diseases and conditions affecting the ileal mucosa, such as Crohn’s disease or ileal resection, lead to a reduction of specific absorptive sites for the intrinsic factor-vitamin B12 complex.24 Ileal resections of more than 60 cm usually result in clinically significant vitamin B12 malabsorption.28 Imerslund-Gräsbeck syndrome, a disease of autosomal recessive inheritance due to malfunction of the cubilin-amnionless (AMN) complex, is characterized by selective ileal malabsorption of the intrinsic factor–vitamin B12 complex despite normal ileal morphology.24,29 Congenital diseases affecting transcobalamin II also result in malabsorption of cobalamin.24,30
Folate
Folate malabsorption occurs with mucosal diseases affecting the proximal small intestine, such as celiac disease, Whipple’s disease, and tropical sprue.31 Folate deficiency is common in chronic alcoholism, in which it is postulated to be caused by decreased dietary intake as well as decreased intestinal absorption of folate.32 As discussed later, several drugs result in impaired intestinal uptake of folate, and an inherited form of selective folate malabsorption has been described. In contrast with cobalamin, body stores of folate are small relative to the daily requirements; therefore, folate deficiency develops faster than cobalamin deficiency in the setting of malabsorption. Increased serum folate levels resulting from bacterial formation of tetrahydrofolate have been reported in small intestinal bacterial overgrowth states.33
Other Water-Soluble Vitamins
Other water-soluble vitamins, such as ascorbic acid and the B-complex vitamins, are absorbed in the small intestine either by carrier-mediated transport or by passive diffusion. Generalized malabsorption syndromes from intestinal causes impair the absorption of these vitamins, thereby leading to deficiency states.34,35 Deficiency of these water-soluble vitamins also occurs in chronic alcoholism, probably owing to decreased oral intake and reduced intestinal absorption.32
MINERALS
CALCIUM
Severe calcium malabsorption can occur in diseases that affect the small intestinal mucosa, such as celiac disease. In these disease states, calcium absorption is impaired directly because of the reduction of the intestinal surface area and indirectly because of formation of insoluble calcium soaps with malabsorbed long-chain fatty acids. Therefore, diseases causing malabsorption of long-chain fatty acids by other mechanisms, such as bile acid deficiency, also can result in calcium malabsorption.21 In many of these diseases, malabsorption and deficiency of vitamin D further contribute to intestinal calcium malabsorption.21 Selective intestinal malabsorption of calcium—that is, without fat malabsorption—can occur in renal disease, hypoparathyroidism, and inborn defects in formation of 1α,25-dihydroxyvitamin D or in the intestinal vitamin D receptor.21 Calcium malabsorption also occurs commonly after gastric resection (see subsequent section, “Malabsorption after Gastric Resection”).
MAGNESIUM
In many generalized malabsorptive disorders, magnesium malabsorption can result in magnesium deficiency.36 Malabsorption is due to the reduction in mucosal absorptive surface area and to luminal binding of magnesium by malabsorbed fatty acids; a congenital form of selective intestinal magnesium malabsorption also has been reported.37
IRON
Iron deficiency is common in patients with gastric resection or with celiac disease. Reduction in the mucosal surface area of the small intestine as a result of diffuse mucosal disease, intestinal resection, or intestinal bypass also can result in impaired iron absorption, potentially leading to iron deficiency38; a congenital form of iron malabsorption also has been described (see Table 101-14).39 Intestinal loss of iron from chronic gastrointestinal bleeding is, however, the most common gastrointestinal cause of iron deficiency.40 Worldwide, hookworm infection is a common cause of iron deficiency.
ZINC
Zinc, like other minerals, is malabsorbed in generalized mucosal diseases of the small intestine.41 A congenital selective defect of zinc absorption, acrodermatitis enteropathica, is caused by a defect in the zinc transport protein hZIP4.42
OTHERS
Generalized malabsorption can cause deficiency of copper and selenium.43,44 In Menkes disease (kinky hair disease), an inherited disorder of cellular copper transport, selective intestinal copper malabsorption results (see later on). It is uncertain whether malabsorptive diseases result in deficiencies of chromium and manganese.41
MECHANISMS THAT COMPENSATE FOR MALABSORPTION
ROLE OF THE COLON
The colon has the capacity to absorb a limited number but a wide variety of substances and nutrients including sodium, chloride, water, oxalate, short chain fatty acids, calcium, and vitamin K. Although colonic nutrient absorption does not play a major role in health, the nutritive role of the colon in patients with severe malabsorption is clinically relevant.45 Colonic preservation of malabsorbed nutrients also can result in symptoms and complications of malabsorption,46 such as colonic hyperabsorption of oxalate, which contributes to formation of renal stones (see later on).
Colonic Salvage of Incompletely Absorbed Carbohydrates
In healthy people, between 2% and 20% of ingested starch escapes absorption in the small intestine47; pancreatic insufficiency or severe intestinal disorders further increase this amount.17 Carbohydrates that reach the colon cannot be absorbed by the colonic mucosa, but they can be metabolized by the colonic bacterial flora. Metabolism by anaerobic bacteria results in the breakdown of oligosaccharides and polysaccharides to mono- and disaccharides, which are metabolized further to lactic acid; short-chain (C2 to C4) fatty acids (SCFAs) such as acetate, propionate, and butyrate; and to odorless gases, including hydrogen, methane, and carbon dioxide.48
Studies in normal subjects have suggested that the bacterial metabolism of starch to small carbohydrate moieties is a rapid process in the normal colon. The rate-limiting step in the overall conversion of polysaccharides to SCFAs appears to be the conversion of monosaccharides to SCFAs.17 Colonic absorption of SCFAs results in a reduction of the osmotic load and, as a result, in mitigation of osmotic diarrhea.49 In normal subjects, more than 45 g of carbohydrates must reach the colon to cause diarrhea, and up to 80 g of carbohydrates per day can be metabolized by bacteria to SCFAs; approximately 90% of these SCFAs are absorbed by colonic mucosa50 (Fig. 101-1). Chronic carbohydrate malabsorption causes adaptive changes in bacterial metabolic activity that result in an even higher efficiency of the bacterial flora to digest carbohydrates,51 although at the expense of increased flatus production (see later).
Because SCFAs have caloric values between 3.4 and 5.95 kcal/g,52 their colonic absorption can contribute positively to overall calorie balance. In patients with short bowel syndrome, colonic salvage of malabsorbed carbohydrates can save up to 700 to 950 kcal/day, provided that a substantial part of the colon remains in continuity with the small intestine.53 Not all SCFAs are absorbed by the colon, and those that are not absorbed contribute to osmotic diarrhea.
The beneficial effects of colonic bacterial carbohydrate metabolism may be accompanied by side effects due to gas production (see Chapter 16). Up to 10-fold differences in the volume of gas produced in the colon have been observed in normal persons.54 The colon also can absorb gas. If intracolonic gas volumes are low, up to 90% of the volume of intracolonic gas can be absorbed; if gas volumes are high, however, this proportion can decrease to 20%54 (Fig. 101-2). Therefore, persons who have the disadvantage of producing more gas in their colons have an additional disadvantage of absorbing a smaller fraction of the gas. Gas produced from bacterial carbohydrate metabolism is odorless. The odor of flatus is due to volatile sulfur-containing substrates that result from bacterial metabolism of protein.55
Impaired colonic salvage of carbohydrates has been suggested to contribute to the diarrhea in Crohn’s disease56 and ulcerative colitis.57 Bacterial carbohydrate metabolism may be lessened by antibiotic treatment.58 In some patients, antibiotic-associated diarrhea may be the result of impaired colonic salvage of carbohydrates that normally are not absorbed or the result of dietary fiber that can accumulate in stool because of decreased bacterial fermentation.59
Role of the Colon in Fat Malabsorption
Long-chain triglycerides or fatty acids, which constitute most dietary fat, cannot be absorbed by the human colon. Long-chain fatty acids bind calcium in the colon, thereby increasing the amount of sodium oxalate that is absorbed.60 Fatty acids with chain lengths longer than 12 carbons can cause diarrhea, because they increase mucosal permeability and inhibit colonic absorption of fluid and electrolytes.61 An increase in colonic permeability due to long-chain fatty acids also may be a contributing factor for the increased colonic oxalate absorption seen in patients with steatorrhea and hyperoxaluria.62
Patients with short bowel syndrome can gain caloric energy from colonic absorption of medium-chain fatty acids, coming from medium-chain triglyceride supplementation, if they have at least part of the colon in continuity with the remaining small intestine.63 In the rat colon, absorption of octanoate is not affected by the simultaneous presence of other luminal substrates.64
Colonic Salvage of Calcium
Although most unabsorbed calcium is insoluble when it reaches the terminal ileum,65 preservation of at least half of the colon in patients with extensive small bowel resection improves calcium absorption by about 40%, compared with calcium absorption in patients who have an ileostomy.66 Absorption of calcium requires solubilization of calcium salts. Bacterial metabolism of dietary fiber or incompletely absorbed carbohydrates can help solubilize calcium by causing a decrease in the pH of luminal contents in the colon. Once calcium is solubilized, it can contact the cecal mucosa, which in the rat has been demonstrated to be the site with the highest calcium absorption rate per surface area in the entire intestine.65 Calcium solubilization in the colon from bacterial fermentation of malabsorbed lactose also can occur in patients with lactose malabsorption, because in this condition, the bioavailability of calcium from milk is greater than that from mineral water.67 In addition to their effect on luminal pH, the SCFAs acetate and propionate, which are products of bacterial metabolism of lactose, have been shown to enhance calcium absorption directly in the human colon.68
ROLE OF INTESTINAL TRANSIT IN THE SALVAGE OF MALABSORBED NUTRIENTS
The lower parts of the gastrointestinal tract do not normally contact nutrients, and when they do, intestinal transit time is prolonged.69,70 This delay in transit could contribute to the compensation mechanisms in malabsorptive diseases; however, nutritional salvage by this mechanism has not been quantitated.
CLINICAL FEATURES AND EVALUATION
SUSPECTING AND CONFIRMING THE PRESENCE OF MALABSORPTION
History and Physical Examination
Table 101-4 lists symptoms and signs suggestive of malabsorption, although virtually all can have causes other than malabsorption. For example, greasy stools might indicate malabsorption, but a greasy appearance also can be due to mucus in stool. Floating of stool in the toilet water can be due to a high stool fat content, but it also can be caused by high gas content. Nevertheless, such symptoms and signs are helpful in raising the clinician’s index of suspicion and in guiding the physician as to which specific laboratory tests, structural evaluations, or function tests should be ordered.
Table 101-4 Symptoms and Signs of Malabsorption and Relevant Pathophysiology
SYMPTOM OR SIGN | PATHOPHYSIOLOGIC EXPLANATION |
---|---|
Gastrointestinal | |
Diarrhea |
The current obesity epidemic has led to a changing picture of malabsorption; for example, few patients today with celiac disease are underweight at diagnosis and some are even overweight. These patients have been reported to be less likely to present with classic features, such as diarrhea or anemia. In these patients, a further increase in weight after dietary gluten exclusion may be a cause of morbidity.71
Laboratory Findings
Certain blood tests might yield abnormal results in malabsorption, but with rare exceptions they are not specific for malabsorptive diseases. Blood tests also can be used as a screening tool to help the physician decide how vigorously to evaluate malabsorption. Table 101-5 lists blood tests in which abnormal results should raise the suspicion of malabsorption and stool tests that should be used to confirm the suspicion of malabsorption.
Table 101-5 Laboratory Tests That Are Useful in Patients with Suspected Malabsorption and for Establishing Possible Nutrient Deficiencies
TEST | COMMENT(S) |
---|---|
Blood Cell Count | |
Hematocrit, hemoglobin | Decreased in iron, vitamin B12, and folate malabsorption or with blood loss |
Mean corpuscular hemoglobin or mean corpuscular volume | Decreased in iron malabsorption; increased in folate and vitamin B12 malabsorption |
White blood cells, differential | Decreased in vitamin B12 and folate malabsorption; low lymphocyte count in lymphangiectasia |
Biochemical Tests (Serum) | |
Triglycerides | Decreased in severe fat malabsorption |
Cholesterol | Decreased in bile acid malabsorption or severe fat malabsorption |
Albumin | Decreased in severe malnutrition, lymphangiectasia, protein-losing enteropathy |
Alkaline phosphatase | Increased in calcium and vitamin D malabsorption (severe steatorrhea); decreased in zinc deficiency |
Calcium, phosphorus, magnesium | Decreased in extensive small intestinal mucosal disease, after extensive intestinal resection, or in vitamin D deficiency |
Zinc | Decreased in extensive small intestinal mucosal disease or intestinal resection |
Iron, ferritin | Decreased in celiac disease, in other extensive small intestinal mucosal diseases, and with chronic blood loss |
Other Serum Tests | |
Prothrombin time | Prolonged in vitamin K malabsorption |
β-Carotene | Decreased in fat malabsorption from hepatobiliary or intestinal diseases |
Immunoglobulins | Decreased in lymphangiectasia, diffuse lymphoma |
Folic acid | Decreased in extensive small intestinal mucosal diseases, with anticonvulsant use, in pregnancy; may be increased in small intestinal bacterial overgrowth |
Vitamin B12 | Decreased after gastrectomy, in pernicious anemia, terminal ileal disease, and small intestinal bacterial overgrowth |
Methylmalonic acid | Markedly elevated in vitamin B12 deficiency |
Homocysteine | Markedly elevated in vitamin B12 or folate deficiency |
Citrulline | May be decreased in destructive small intestinal mucosal disease or intestinal resection |
Stool Tests | |
Fat | Qualitative or quantitative increase in fat malabsorption |
Elastase, chymotrypsin | Decreased concentration and output in exocrine pancreatic insufficiency |
pH | Less than 5.5 in carbohydrate malabsorption |
Quantitative fecal fat measurement followed by measurement of fecal chymotrypsin or elastase concentration may be helpful, both in establishing malabsorption and in differentiating between pancreatic and intestinal causes of malabsorption. Low levels of serum β-carotene, cholesterol, triglycerides, and calcium and a prolonged prothrombin time suggest malabsorption of fat and fat-soluble vitamins. Low levels of vitamin B12, folate, iron, and albumin suggest malabsorption of water-soluble substances and, therefore, indicate intestinal disease rather than pancreatic or biliary disease. Severe deficiency of fat-soluble vitamins might indicate intestinal or biliary disorders. Low levels of plasma citrulline are associated with destructive small intestinal disease, such as celiac disease, or can follow intestinal resection,72 although fasting plasma citrulline tests are poor predictors of enterocyte dysfunction in clinical practice; an oral citrulline generation test has been proposed to improve its predictive value.73
DIAGNOSTIC APPROACH
Clinical Clues to the Presence of Specific Diseases
Clinical clues (Table 101-6) or results of laboratory tests (Table 101-7)74 can indicate the presence of a specific underlying disease or can help in the differential diagnosis. In addition, the following questions may be helpful and should be asked as part of the history before physical examination:
Table 101-6 Cardinal Clinical Features of Specific Malabsorptive Disorders
DISORDER | CARDINAL CLINICAL FEATURES |
---|---|
Adrenal insufficiency | Skin darkening, hyponatremia, hyperkalemia |
Amyloidosis | Renal disease, nephrotic syndrome, cardiomyopathy, neuropathy, carpal tunnel syndrome, macroglossia, hepatosplenomegaly |
Bile acid deficiency | Ileal resection or disease, liver disease |
Carcinoid syndrome | Flushing, cardiac murmur |
Celiac disease | Variable symptoms: dermatitis herpetiformis, alopecia, aphthous mouth ulcers, arthropathy, neurologic symptoms, and (life-threatening) malnutrition; abnormal liver biochemical test results, mild iron deficiency |
Crohn’s disease | Arthritis, aphthous mouth ulcers, episcleritis, uveitis, pyoderma gangrenosum, erythema nodosum, abdominal mass, fistulas, primary sclerosing cholangitis (PSC), laboratory signs of inflammation |
Cystic fibrosis | Chronic sinopulmonary disease, meconium ileus, distal intestinal obstruction syndrome (DIOS), elevated sweat chloride |
Cystinuria, Hartnup’s disease | Kidney stones, dermatosis |
Diabetes mellitus | Long history of diabetes and diabetic complications |
Disaccharidase deficiency | Bloating and cramping, intermittent diarrhea |
Gastrointestinal fistulas | Previous intestinal surgery or trauma, Crohn’s disease |
Glucagonoma | Migratory necrolytic erythema |
Hyperthyroidism, hypothyroidism | Symptoms and signs of thyroid disease |
Hypogammaglobulinemia | Recurrent infections |
Intestinal ischemia | Other ischemic organ manifestations; abdominal pain with eating (chronic mesenteric ischemia) |
Lymphoma | Enlarged mesenteric or retroperitoneal lymph nodes, abdominal mass, abdominal pain, fever |
Mastocytosis | Urticaria pigmentosum, peptic ulcer |
Mycobacterium-avium complex infection | Acquired immunodeficiency syndrome |
Pancreatic insufficiency | History of pancreatitis, abdominal pain; or alcoholism; large-volume fatty, oily stools |
Parasitic infection | History of travel to endemic areas |
Primary biliary cirrhosis | Jaundice, itching |
Scleroderma | Dysphagia, Raynaud’s phenomenon, skin tightening |
Small intestinal bacterial overgrowth | Previous intestinal surgery, motility disorder (scleroderma, pseudo-obstruction), small intestinal diverticula, strictures |
Tropical sprue | History of travel to endemic area |
Tuberculosis | Specific history of exposure, living in or travel to endemic area, immunosuppression, abdominal mass or intestinal obstruction, ascites |
Whipple’s disease | Lymphadenopathy, fever, arthritis, cerebral symptoms, heart murmur |
Zollinger-Ellison syndrome | Peptic ulcers, diarrhea |
Table 101-7 Laboratory Tests That Are Useful in the Differential Diagnosis of Malabsorption
TEST | COMMENT |
---|---|
Blood Cell Count | |
Acanthocytes | Abetalipoproteinemia |
Nuclear remnants in erythrocytes (Howell-Jolly bodies) | Splenic atrophy in celiac disease, inflammatory bowel disease, radiation enteritis, amyloidosis |
White blood cells, differential |
ACTH, adrenocorticotropic hormone; AIDS, acquired immunodeficiency syndrome; ANA, antinuclear antibodies; EMA, endomysial antibodies; ESR, erythrocyte sedimentation rate; HIV, human immunodeficiency virus; HLA, human leukocyte antigen; Ig, immunoglobulin; TSH, thyroid-stimulating hormone.
* Perform this test if there is a strong suspicion of an underlying neuroendocrine tumor.77
The sequence of tests thus depends on the affected person’s symptoms and history, as well as results of previous testing (Table 101-8). Tests that can detect the most common causes of malabsorption or are noninvasive or inexpensive usually should be performed initially (first-line tests). In some patients, testing for rarer causes of malabsorption and use of more invasive or more expensive tests may be necessary to establish the diagnosis (second-line tests). For unusually difficult cases, additional tests may be required that may be available only in specialized centers (third-line tests).
Table 101-8 Tests to Establish the Cause of Malabsorption Based on Main Symptoms
Weight Loss, Osteomalacia or Osteopenia, Diarrhea, Suspected Steatorrhea, or Deficiency of Fat-Soluble Vitamins |
First-line Tests |
ALT, alanine aminotransferase; AP, alkaline phosphatase; AST, aspartate aminotransferase; CT, computed tomography; EGD, esophagogastroduodenoscopy; ERCP, endoscopic retrograde cholangiopancreatography; 5-HIAA, 5-hydroxyindoleacetic acid; IgE, immunoglobulin E; MCH, mean corpuscular hemoglobin; MCV, mean corpuscular volume; MRCP, magnetic resonance cholangiopancreatography; MRI, magnetic resonance imaging; PAS, periodic acid–Schiff; PCR, polymerase chain reaction; TSH, thyroid-stimulating hormone.
ENDOSCOPY, BIOPSY, AND DUODENAL ASPIRATION
Endoscopy
Endoscopic inspection of the duodenal mucosa can provide clues to some causes of malabsorption. Aphthae suggest Crohn’s disease, and small, diffuse, white, punctate lesions can be seen in primary or secondary lymphangiectasia. Mosaic-like scalloping of duodenal folds (Fig. 101-3) and reduction in the number of duodenal folds are highly suggestive of villus atrophy in celiac disease, although these abnormalities may be seen in other diseases (see Chapter 104).75 Villus atrophy may be seen endoscopically using magnification endoscopy76 and chromoendoscopy with indigocarmine staining; however, a normal duodenal fold pattern should not deter the endoscopist from taking mucosal biopsy specimens. Endocrine tumors causing malabsorption, such as duodenal gastrinomas or somatostatinomas or ampullary tumors obstructing the pancreatic duct, also can be detected during endoscopy. If ileal disease is the suspected cause of malabsorption, visual examination and biopsy of the ileal mucosa may be required to establish a diagnosis; this can be accomplished by retrograde intubation of the ileum at colonoscopy or by double-balloon endoscopy.
Biopsy
Examination of endoscopic biopsy specimens from the duodenum may be diagnostic or highly suggestive of a variety of small bowel disorders resulting in malabsorption (Table 101-9); follow-up small intestinal biopsy can be used to assess treatment effects. Duodenal biopsy specimens should be obtained from patients with atypical or nonspecific gastrointestinal symptoms, including abdominal pain, bloating, and weight loss, and should not be limited only to patients with diarrhea.77,78 Endoscopic biopsy is an adequate substitute for jejunal suction biopsy,79 and its advantages over capsule biopsy are that multiple specimens are more easily obtained and focal or patchy lesions can be identified and targeted for sampling.80 Compared with duodenal biopsies, endoscopically obtained biopsies from the jejunum to find changes of celiac disease are helpful in only very few patients.81 The adequacy of mucosal biopsy specimens is a function of their size and the number obtained.82 If large specimens can be obtained using jumbo biopsy forceps, they can be oriented on a piece of filter paper before they are put into a fixing solution83; two or three jumbo biopsy specimens usually are sufficient to allow histologic sectioning parallel to the villi and crypts. Specimens also may be obtained with smaller forceps, although the number of specimens obtained must then be increased to four to six. Specimens can be inspected with a low-power dissecting microscope or by magnification endoscopy to obtain an initial impression of the villus architecture and to ensure proper orientation before they are placed in formalin.
Table 101-9 Causes of Malabsorption That Can Be Diagnosed by Small Bowel Biopsy
CAUSE OF MALABSORPTION | MAIN HISTOLOGIC FEATURES |
---|---|
Generalized Histologic Abnormalities | |
Abetalipoproteinemia, hypobetalipoproteinemia | Lipid accumulation and vacuolization of enterocytes |
Collagenous sprue (Chapter 104) | Collagenous band below atrophic epithelium |
Mycobacterium-avium complex infection (Chapter 33) | Acid-fast bacilli, foam cells |
Whipple’s disease (Chapter 106) | Foamy macrophages with PAS-positive inclusion bodies |
Patchy Histologic Abnormalities | |
Amyloidosis (Chapter 35) | Congo red–stained deposits with apple-green birefringence in polarized light |
Crohn’s disease (Chapter 111) | Epithelioid granulomas and characteristic focal inflammation |
Eosinophilic gastroenteritis (Chapter 27) | Eosinophilic infiltration |
Lymphangiectasia (Chapter 28) | Ectatic lymph vessels |
Lymphoma (Chapter 29) | Clonal expansion of lymphocytes |
Mastocytosis (Chapter 35) | Diffuse infiltration with mast cells |
Parasites (Giardia lamblia, Strongyloides stercoralis, coccidia) (Chapters 109, 110) | Some parasites may be seen on histologic examination |
PAS, periodic acid–Schiff.
Modified from Riddell RH. Small intestinal biopsy: Who? how? what are the findings? In Barkin JS, Rogers AI, editors. Difficult Decisions in Digestive Diseases. Chicago: Year Book; 1989. p 326.
The diagnostic yield of biopsy is influenced by the distribution of histologic abnormalities, which in some diseases is diffuse but in other diseases is patchy. Tropical diarrhea malabsorption syndrome (tropical sprue; see Chapter 105), abetalipoproteinemia, and immunodeficiency usually result in a diffuse alteration of small intestinal mucosa. Thus, a completely normal appearance of a duodenal biopsy specimen rules out these disorders. Primary lymphangiectasia has a patchy distribution, so that a single mucosal biopsy might not rule out the disorder (see Chapter 28). Patchy distribution also has been described for the histologic changes in some patients with celiac disease, especially when symptoms are subtle, although this disorder usually affects the small intestine diffusely.84 Other possible sources of error and misdiagnosis include poorly oriented specimens and those obtained proximally, where peptic injury can be the cause of mucosal alterations. Additional biopsy specimens from the stomach and duodenal bulb can help the pathologist to establish the extent of peptic injuries in the upper gastrointestinal tract and to interpret inflammatory changes in the duodenum in relation to these lesions. Distortion of villus architecture over Brunner’s glands or lymphoid aggregates, common in the duodenum, should be interpreted with caution.
Specific histologic features may be diagnostic for some rare causes of malabsorption (see Table 101-9)85 such as Whipple’s disease (Fig. 101-4), abetalipoproteinemia or hypobetalipoproteinemia, intestinal lymphangiectasia, giardiasis (Fig. 101-5), lymphoma, or collagenous sprue. In most patients with small intestinal disorders, however, histologic examination is not diagnostic85 (Table 101-10) and reveals a spectrum of mucosal responses ranging from infiltration by lymphocytic cells to a flat mucosa with villus atrophy and crypt hyperplasia (Fig. 101-6). In many parts of the world, celiac disease is by far the most common cause of this type of histologic alteration, but a definite diagnosis of celiac disease cannot be established by mucosal biopsy alone (see Chapter 104).
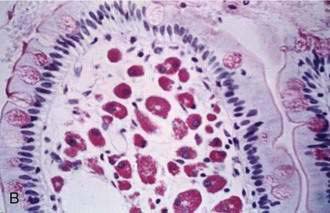
B, High-power view demonstrates purple-red macrophages. (Periodic acid–Schiff stain.)
(Courtesy of Günter J. Krejs, MD, Graz, Austria.)
Table 101-10 Malabsorptive Diseases with Abnormal but Not Diagnostic Small Intestinal Histologic Findings
Increased Lymphocyte Infiltration with or without Crypt Hyperplasia |
Flat Lesion with or without Mucosal Inflammation |
Atrophic Lesion |
AIDS, acquired immunodeficiency syndrome; NSAIDs, nonsteroidal anti-inflammatory drugs.
Modified from Riddell RH. Small intestinal biopsy: Who? how? what are the findings? In Barkin JS, Rogers AI, editors. Difficult Decisions in Digestive Diseases. Chicago: Year Book; 1989. p 326.
Some disease states can be identified only with use of special histologic stains, such as Congo red (intestinal amyloidosis), periodic acid–Schiff (PAS) (Whipple’s disease), or immunohistochemical techniques for detecting refractory celiac disease, small intestinal lymphoma, or enteroendocrine insufficiency (see later on). Polymerase chain reaction analysis of intestinal biopsy specimens for Tropheryma whipplei may be helpful in evaluating patients in whom Whipple’s disease is suspected (see Chapter 106).86 In cases in which these diseases are a possibility, the clinician has to request these specific tests. Measurement of mucosal enzyme activities in a jejunal biopsy can be used to confirm disaccharidase deficiency, although this is not recommended for routine clinical use.
Aspiration
Fluid aspirated from the descending part of the duodenum may be examined microscopically for Giardia lamblia (see Chapter 109) or cultured to detect bacterial overgrowth in patients with diffuse small intestinal motility disorders (see Chapters 97 and 102).
Video Capsule Endoscopy
Video capsule endoscopy (VCE) is an increasingly popular technique for diagnosing diseases of the small intestine. VCE was initially introduced for evaluating suspected bleeding in the small intestine, but subsequently it has been used to diagnose a wider range of diseases such as Crohn’s disease, celiac disease, and other malabsorptive disorders. In several studies, lesions suggesting Crohn’s disease were detected by VCE when they had been missed by conventional diagnostic procedures.87 These reports need to be interpreted carefully, because no biopsy specimens were obtained, and long-term evaluations to confirm the diagnosis are lacking.
VCE appears to be superior to conventional radiologic imaging of the small intestine and to computed tomography (CT) with small bowel enteroclysis to detect subtle mucosal changes, such as aphthous or erosive lesions of the small intestine.87 In celiac disease, the detection of villus atrophy by VCE has a good correlation to villus atrophy seen in duodenal biopsy specimens,88,89 but it is questionable if this procedure can detect subtle changes, such as Marsh 1 and 2 lesions. Changes on VCE that suggest villus atrophy are scalloping, mosaic pattern, and fissuring. In a recent study of VCE in patients with celiac sprue, villus atrophy was seen in the duodenum and jejunum in 59% of cases, in the duodenum only in 32%, and in the jejunum only in 3%.89
In refractory celiac disease, VCE can detect changes such as ulcerations and strictures that suggest T-cell lymphoma but that are missed by conventional techniques.90 This test may be used in patients with established malabsorption in whom no diagnosis has been established despite extensive diagnostic workup.
ABDOMINAL IMAGING
Small Bowel Follow-through and Small Bowel Enteroclysis
The principal role of small bowel radiologic series in evaluating malabsorption is to identify focal or diffuse abnormalities and alterations that predispose to bacterial overgrowth, including diverticula, stagnant loops of intestine, generalized intestinal hypomotility or dilatation, intestinal fistulas, and tumors.91
Small bowel enteroclysis is preferred to small bowel follow-through examinations, because distention of the lumen results in better demonstration of the small bowel contour.92 Double-contrast enteroclysis, in which intubation of the upper jejunum is used to instill contrast material directly into the upper jejunum, has a higher sensitivity than small bowel series for detecting mucosal changes, although it is less acceptable to the patient and can miss focal changes in the duodenum, such as diverticula. Use of an intravenous agent such as glucagon to reduce motility enables overlapping loops of small intestine to be separated and imaged more distinctly.
Ulcerations and strictures may be seen in various malabsorptive disorders, including Crohn’s disease, radiation enteritis, celiac disease, intestinal lymphoma, and tuberculosis. Aphthous ulcers and cobblestoning of the mucosa, either alone or with thickened and distorted folds, are features of Crohn’s disease but also can be present in other conditions. Reduced numbers of jejunal folds and an increased number of and thickening of ileal folds can suggest celiac disease.91 Mass lesions can be found with intestinal lymphoma or, rarely, with hormone-producing tumors.
The disadvantage of conventional enteroclysis is that direct imaging of the bowel wall and surrounding structures is not possible, and overlapping bowel loops potentially impair complete visualization of the whole small bowel—hence the rationale for combining enteroclysis with CT or MRI scanning.93
Abdominal Computed Tomography
Abdominal CT for small bowel investigation is performed after administration of oral or intravenous contrast agents.94 Small intestinal CT scanning is useful to detect focal intestinal lesions, such as thickening of the small bowel wall in Crohn’s disease or small intestinal lymphoma, intestinal fistula, and dilated bowel loops; however, mild mucosal changes such as aphthae in Crohn’s disease or villus atrophy of various causes are missed by this technique. Diffuse thickening of the small bowel may be seen in Whipple’s disease and in graft-versus-host disease.94 In some cases of celiac disease, reversal of the jejunoileal fold pattern is observed.95 CT is a sensitive test for detecting enlarged abdominal lymph nodes, which can be present in disorders such as Whipple’s disease, small bowel lymphoma, or small intestinal inflammatory diseases such as Crohn’s disease. Evidence for pancreatic disease that may be detected on CT includes calcifications of the pancreas, dilatation of the pancreatic duct, and pancreatic atrophy. Tumors obstructing the pancreatic duct or hormone-secreting neuroendocrine tumors also can be located by CT.
Magnetic Resonance Imaging of the Small Intestine
MRI may be used to image the small intestine either with administration of oral contrast solutions or by enteroclysis. Segmental bowel wall thickening with inflammatory involvement of the mesentery, cobblestoning, and ulcerations may be seen in Crohn’s disease; this method is very sensitive for demonstrating complications of Crohn’s disease, such as intestinal fistula formation. In celiac disease, small bowel MRI with oral contrast can demonstrate small intestinal dilatation, mucosal thickening, and an increased number of folds in the ileum (ileal jejunization) with flattening of the jejunal folds.96 Most of these signs also are found in other inflammatory diseases of the intestine, but the fold pattern abnormalities are most specific for celiac disease.96 This method also is very useful to detect changes suggesting complications, such as lymphoma or carcinoma. With MRI enteroclysis, subtle mucosal changes might be missed and be more evident on conventional small bowel enteroclysis97 or capsule endoscopy.87 Because MRI or CT imaging of the small intestine requires no tube placement, these techniques have largely replaced classic small bowel enteroclysis.
Other Radiologic Studies
Endoscopic retrograde cholangiopancreatography (ERCP) can help establish the cause of pancreatic insufficiency (see Chapter 59). It can help distinguish chronic pancreatitis from pancreatic tumor and can document pancreatic duct stones. ERCP and endoscopic ultrasound (EUS) are the methods of choice for documenting various causes of biliary obstruction. Magnetic resonance pancreatography (MRCP) is increasingly being used to replace diagnostic ERCP. If a neuroendocrine tumor (e.g., gastrinoma, somatostatinoma) is the suspected cause of malabsorption, an indium-111 octreotide scintigraphic scan, positron emission tomography (PET), or an EUS examination of the pancreas may be helpful in establishing the diagnosis or demonstrating the extent of disease (see Chapters 31 and 32).
NONINVASIVE EVALUATION OF GASTROINTESTINAL DIGESTIVE AND ABSORPTIVE FUNCTION
Some conditions causing malabsorption can be diagnosed with noninvasive tests, although, as pointed out in Table 101-11, diagnostic accuracy may be limited, and further tests may be necessary to identify underlying diseases or to differentiate primary and secondary causes. Apart from providing a diagnosis, tests evaluating gastrointestinal absorptive and digestive function may be helpful in evaluating complex disease presentations. For most or all of the following tests, the potential benefits with regard to the costs of workup or to patient acceptability have not been established. Because test procedures and analytical methods can vary among laboratories,98 each laboratory should establish its own reference values for these tests.
Table 101-11 Malabsorptive Diseases or Conditions in Which Noninvasive Tests Can Establish Malabsorption or Provide a Diagnosis
DISEASE OR CONDITION | DIAGNOSTIC TEST(S)* | COMMENT(S) |
---|---|---|
Lactose malabsorption |
SeHCAT, selenium-75-homotaurocholic acid test; TCA, taurocholic acid.
* See text for diagnostic accuracy of the different tests listed.
Fat Malabsorption
Quantitative Fecal Fat Analysis
The van de Kamer method is the quantitative titration of fatty acid equivalents in which the results are expressed as fecal output of fat in grams per 24 hours. This method is considered the gold standard for fecal fat analysis.99 Modifications in which the extracted fats are weighed rather than titrated100 have an excellent correlation with the results of the van de Kamer method. Near-infrared reflectance analysis may be a less-cumbersome method to quantify fecal fat output in stool collections101 because it requires less handling of stool by laboratory personnel, but it still requires a 48- to 72-hour collection to exclude the influence of day-to-day variability; the stool must be mixed before a sample is obtained for analysis.
Fecal fat excretion of less than 7 g per day with a fat intake of 100 g per day usually is considered normal. However, the volume effect of diarrhea by itself increases fecal fat output to levels of up to 14 g per day (secondary fat malabsorption)102 (Fig 101-7); this latter value could be used as the upper limit of normal in patients with diarrhea. Diet is important in considering causes of steatorrhea; for example, elevated fecal fat values can be observed in patients consuming a diet rich in the fat substitute olestra.100
Despite the limitations of quantitative fecal fat analysis, it nevertheless is still useful in several clinical circumstances: to establish malabsorption and avoid nutritional deterioration103 when overt features of intestinal or pancreatic disorders are lacking, as in some cases of osteoporosis, osteomalacia, anemia, or weight loss; to monitor treatment in patients with established malabsorptive disorders, such as exocrine pancreatic insufficiency or short bowel syndrome; to estimate fecal calorie loss in patients with severe malabsorption syndromes; and to quantitate fecal fat excretion in patients with diarrhea who have undergone ileal resection, thereby distinguishing steatorrhea due to bile acid deficiency from secretory diarrhea caused by bile acid loss.104
Semiquantitative Fat Analysis
For the acid steatocrit test,105 a sample of stool is diluted 1 : 3 with distilled water in a test tube. The diluted stool is homogenized, and a 500-µL aliquot is pipetted into a tube. Then 100 mL of 5M HClO4 is added to allow better fat extraction and separation of the lipid layer. An aliquot of the diluted stool-HClO4 mixture is put into a nonheparinized microcapillary tube and sealed on one end. After centrifugation of this aliquot at 13,000 rpm for 15 minutes, the fatty layer (FL) and the solid layer (SL) are measured, and the acid steatocrit (AS) is determined according to the following equation:
An acid steatocrit of less than 31% is normal. In a small study, the acid steatocrit for random spot stool samples had a high sensitivity and specificity for detection of steatorrhea, compared with the van de Kamer method, which is performed on a 72-hour stool collection. A linear correlation also was found between results obtained with the acid steatocrit and those of the van de Kamer method, although results were quite divergent in some patients.105 Because quantitative fecal fat measurements are based on 48- to 72-hour stool collections (to minimize the effect of day-to-day variability in fecal fat excretion), however, the acid steatocrit cannot be expected to replace quantitative measurement of fat output in borderline cases or in cases in which exact measurement of fecal fat loss is required.
Qualitative Fecal Fat Analysis
Fat analysis by microscopic examination of random stool samples might provide a clue to the presence of steatorrhea, although it cannot be used to exclude steatorrhea; its sole advantage is its ease of performance. A sample of stool is placed on a glass slide to which several drops of glacial acetic acid and Sudan III stain are added. Acidification of stool samples improves fat extraction and separation of the lipid layer.105 The slide is held over a flame-burner and the acidified mixture is heated to boiling and then examined while still warm for presence of orange fat globules. A count of up to 100 globules with a diameter less than 4 mm per high-power field is normal.6
Results of qualitative fat analysis by this method and of quantitative fat analysis do not correlate very well.106 In a small study, Sudan staining of spot stool samples had a sensitivity of 78% and a specificity of 70% for the detection of steatorrhea.105 A quantitative microscopic method of counting and measuring fat globules using the Sudan stain has been shown to correlate well with chemically measured fecal fat output.107
Breath Tests for Fat Malabsorption
The principle of the 14C-triolein breath test is to measure 14CO2 in the breath after ingestion of a triglyceride that has been radiolabeled with 14C. Fat malabsorption results in decreased pulmonary excretion of 14CO2.108 Because of erroneous results in a variety of metabolic and pulmonary diseases, lack of sensitivity in mild malabsorption, radiation exposure to the patient, cost of the substrate, and the need for expensive equipment, this test has not found widespread acceptance for clinical use; the nonradioactive isotope 13C is used to label triglycerides instead (see later on).
Serum Tests for Fat Malabsorption
The photometric measurement of β-carotene at 456 nm109 has been suggested as a useful screening test for steatorrhea, although experience with this technique is limited; values less than 100 mg per 100 mL suggest the presence of steatorrhea, and values less than 47 mg per 100 mL strongly indicate steatorrhea. Concentrations in excess of 100 mg per 100 mL do not exclude mild steatorrhea, although they make steatorrhea with fat losses in excess of 16 g per day very unlikely. Normal values also have been established in the pediatric population.110 β-Carotene can be falsely low in patients with liver disease or in alcoholics who consume a diet deficient in β-carotene. Disorders in lipoproteins or intake of carotene-containing food additives also can influence the results.
Carbohydrate Malabsorption
The hydrogen breath test is a noninvasive test that takes advantage of the fact that in most people, bacterial metabolism of carbohydrate results in accumulation of hydrogen, which then is absorbed by the colonic mucosa and excreted in the breath. Using different carbohydrates, such as lactose or fructose, the hydrogen breath test can be used to detect malabsorption of these carbohydrates. Measurement of breath hydrogen excretion after ingestion of lactulose has been used to assess orocecal transit time, and glucose has been used as a substrate to detect small bowel bacterial overgrowth, although sensitivity and specificity are poor.111 Unfortunately, up to 18% of people are hydrogen nonexcretors,112 and in these persons, hydrogen breath test results may be falsely negative because hydrogen is metabolized by bacteria to methane. Such limitations and pitfalls of breath hydrogen testing have to be taken into account when test results are interpreted.113
The diagnosis of lactose malabsorption is established if an increase in breath hydrogen concentration of greater than 20 parts per million over baseline occurs after ingestion of 20 to 50 grams of lactose. An increase within the first 30 minutes after ingestion of lactose has to be disregarded, because it may be due to bacterial degradation of lactose in the oral cavity. Up to four hours may be required for the increase in breath hydrogen concentration to occur. Breath hydrogen measurements obtained before and at 30, 60, 90, 180, and 240 minutes after ingestion of 50 grams of lactose provide the best diagnostic yield with the fewest possible measurements.112
The lactose hydrogen breath test still is performed by most clinicians for evaluating lactose malabsorption, but this test can miss the disorder in hydrogen nonexcretors. In these patients, a lactose tolerance test—measurement of blood glucose levels before and 30 minutes after ingestion of 50 grams of lactose—can be used. An increase in glucose concentration of less than 20 mg/dL over baseline within 30 minutes of ingestion of 50 grams of lactose indicates lactose malabsorption. The lactose tolerance test has a lower sensitivity than the lactose hydrogen breath test for diagnosing lactose malabsorption.112
Lactase deficiency in acquired primary lactase deficiency (adult-type hypolactasia) is not caused by mutations in the gene coding for intestinal lactase (LPH gene). It has been shown, however, that a single-nucleotide polymorphism (SNP), either the C or T nucleotide −13910 upstream of the LPH gene, is involved in the regulation of intestinal lactase expression.114 A CC genotype at −13910 C/T is associated with acquired primary lactase deficiency (adult-type hypolactasia), whereas TC and TT genotypes are linked with lactase persistence.115,116 This polymorphism can be used as a diagnostic test for adult-type hypolactasia.116 This SNP is only associated with adult-type hypolactasia in whites; other SNPs are linked to adult-type hypolactasia or lactase persistence in Africans.117 In patients with diarrhea, a stool test to detect a fecal pH lower than 5.5 can serve as a qualitative indicator of carbohydrate malabsorption.118 In the research setting, fecal carbohydrates can be determined by the anthrone method, which measures carbohydrates on a weight basis.119 By contrast, the reducing sugar method gives results on a molar basis and, therefore, provides information about the osmotic activity of malabsorbed carbohydrates.17 Total SCFAs and lactic acid, which are the products of bacterial carbohydrate metabolism, can be measured in stool by titration.120 Individual SCFAs can be determined by gas chromatography.121
Protein Malabsorption
The classic test to quantify protein malabsorption, measurement of fecal nitrogen content in a quantitatively collected stool specimen,12 rarely is used today. For research purposes, a combined 14C–octanoic acid–13C–egg white breath test, accompanied by measurement of the urinary output of phenol and p-cresol, has been used to assess the effect of gastric acid on protein digestion.122 In this method, labeling of the 13C–egg protein test meal with 14C–octanoic acid allows the simultaneous measurement of protein assimilation and gastric emptying rate. Phenol and p-cresol are the quantitatively most important phenolic compounds in the feces and urine and are specific metabolites of tyrosine, produced by bacterial fermentation in the colon. They result from protein that has escaped digestion and absorption in the small intestine and are rapidly absorbed in the colon, detoxified, and excreted in urine. Recovery of higher amounts of urinary phenols observed after omeprazole treatment in the study of this test indicated an increased availability of protein in the colon.
Vitamin B12 (Cobalamin) Malabsorption
The Schilling Test
The Schilling test can be used clinically to distinguish between gastric and ileal causes of vitamin B12 deficiency. Because both intrinsic factor and hydrochloric acid are produced by parietal cells in humans, alternative approaches to diagnosing pernicious anemia are to document atrophic gastritis by endoscopy and biopsy, to confirm achlorhydria by acid secretion analysis and increased serum gastrin levels, and to look for antibodies in the serum directed against parietal cells or intrinsic factor.26,123 Because the intrinsic factor used in the Schilling test is of bovine origin, the test is not commercially available in some countries.
The Schilling test is performed by administering a small oral dose of radiolabeled vitamin B12 and, simultaneously or within one or two hours, a large intramuscular flushing dose of nonradiolabeled vitamin B12. The unlabeled B12 saturates vitamin B12 carriers; thus, any radioactive vitamin B12 absorbed by the intestine is excreted in the urine. If less than 7% to 10% of the administered dose is recovered in urine within 24 hours, vitamin B12 malabsorption is confirmed. To specify the site of vitamin B12 malabsorption, a second phase of the Schilling test is performed subsequently with oral administration of intrinsic factor. In patients with pernicious anemia, the results of the Schilling test normalize after oral administration of intrinsic factor.24,123
Patients with pancreatic exocrine insufficiency might have an abnormal result on the Schilling test, with or without added intrinsic factor, but results normalize with addition of pancreatic enzymes (Chapter 59). In small bowel bacterial overgrowth the results of the Schilling test can improve after antibiotic therapy (Chapter 102). In ileal disease or following ileal resection, abnormal results of the Schilling test persist despite intrinsic factor. Schilling test results are normal in patients with dietary vitamin B12 deficiency, in protein-bound (food-bound) vitamin B12 malabsorption,24 and sometimes in congenital transcobalamin II deficiency.124 False-positive results on the Schilling test can result from renal dysfunction or inadequate urine collection.123 The value of this test is diminished by the need for accurately timed urine collections. Results in the 5% to 10% excretion range often are difficult to interpret. A variation of the standard Schilling test is the dual-isotope or single-stage Schilling test, in which two different cobalamin isotopes are given simultaneously, one of them bound to intrinsic factor. This makes it possible to perform the first two phases of the Schilling test in one day; however, the results of this test are not as accurate as those obtained with the standard protocol.24,123
Serum Test for Vitamin B12 and Folate Deficiency
Measurements of serum cobalamin and folate concentrations commonly are used to detect deficiency states of these vitamins. The sensitivity and specificity of these tests are unknown because no gold standard test has been established and because serum levels do not always correlate with body stores.24,125 Furthermore, results of vitamin B12 levels vary with different commercial tests.126
Several causes of misleading serum cobalamin levels have been established. Serum vitamin B12 levels can be normal despite depleted body stores in small intestinal bacterial overgrowth (as a result of production of inactive cobalamin analogs by the bacteria), liver disease, myeloproliferative disorders, congenital transcobalamin II deficiency, and with high levels of intrinsic factor antibodies. In contrast, oral contraceptives, pregnancy, and folate deficiency can cause low serum cobalamin levels despite normal body stores.123 Therefore, if there is a high suspicion, especially for cobalamin deficiency, parenteral replacement with monitoring of the clinical response is recommended.126 Measurement of methylmalonic acid, homocysteine, and holotranscobalamin are of limited clinical use in establishing vitamin B12 deficiency.126
Serum folate concentrations decrease within a few days of dietary folate restriction, even if tissue stores are normal. Feeding also influences serum folate levels; therefore, determination of folate in the fasting state is recommended. Measurement of red blood cell folate concentration has been considered a better estimate of folate tissue stores than serum folate levels by some authors.123
Small Intestinal Bacterial Overgrowth
Tests for the diagnosis of bacterial overgrowth are covered in more detail in Chapter 102. Briefly, tests used to diagnose bacterial overgrowth are the quantitative culture of a small intestinal aspirate (which is considered to be the gold standard diagnostic test), measurement of deconjugated bile acids or vitamin B12 analogs in intestinal aspirates, measurement of serum folate, and several breath tests, including the 14C-glycocholate breath test, the 14C-d-xylose breath test, the lactulose hydrogen breath test, and the glucose hydrogen breath test. The rationale for the breath tests is the production by intraluminal bacteria of volatile metabolites (i.e., 14CO2 or H2), from the administered substances, which can be measured in the exhaled breath.
Exocrine Pancreatic Insufficiency
Pancreatic function tests are discussed in detail in Chapters 56 and 59. Invasive pancreatic function tests require duodenal intubation and measurement of pancreatic enzyme, volume, and bicarbonate output after pancreatic stimulation by a liquid test meal (the Lundh test) or by injection of cholecystokinin (CCK) or secretin. Noninvasive tests include measurement of fecal chymotrypsin or elastase concentration, the fluorescein dilaurate test, and the N-benzoyl-l-tyrosyl para-aminobenzoic acid (NBT-PABA) test. Elastase has a higher sensitivity for the detection of exocrine pancreatic insufficiency compared with chymotrypsin,127 but the specificity of elastase is low.128
Measurement of pancreatic enzymes and components of pancreatic fluid in duodenal aspirates obtained during endoscopy and after intravenous stimulation with secretin and CCK can have an excellent correlation with the more classic intubation tests for secretory function.129
Bile Salt Malabsorption
Measurement of Fecal Bile Acid Output
Elevated fecal bile acid concentrations or output can indicate intestinal bile acid malabsorption.130 Under steady-state conditions, the increased fecal bile acid output reflects increased hepatic synthesis of bile acids.131 In severe bile acid malabsorption, fecal bile acid output can be reduced if hepatic synthesis of bile acids is impaired. The measurement can be performed by enzymatic methods or by gas chromatography. This test requires a quantitative stool collection, and the analytic techniques are time consuming and require considerable expertise. Enzymatic methods may be unreliable in severe steatorrhea.132
Carbon-14–Taurocholate Bile Acid Absorption Test
The 14C-taurocholate bile acid absorption test requires a 72-hour stool collection after ingestion of radioactively labeled bile acid. The rate of intestinal bile acid absorption is calculated from the fecal recovery of 14C-labeled taurocholic acid (14C-TCA). Normal values for this test have been established in normal persons with laxative-induced diarrhea, because diarrhea by itself can increase fecal losses of bile acids,131 presumably because of accelerated intestinal transit.133 Clinical limitations of this test are that it requires substantial analytical work, access to a gamma camera, and a time-consuming stool collection.
Therapeutic Trial of Bile Acid–Binding Resins (Cholestyramine)
In patients with established bile acid malabsorption in whom no improvement is obtained with bile acid–binding resins, it is very unlikely that bile acid malabsorption is the cause of diarrhea. In these patients, bile acid malabsorption is considered a secondary phenomenon due to a washout effect.131 In patients with severe bile acid malabsorption resulting in steatorrhea, cholestyramine might even aggravate fat malabsorption and diarrhea.3 Therefore, without further testing for bile acid malabsorption, neither a positive nor a negative result of a therapeutic trial of cholestyramine constitutes proof of the presence or absence, respectively, of bile acid malabsorption.
Selenium-75–Labeled Homotaurocholic Acid Test
The radioactive taurocholic acid analog used for this test is resistant to bacterial deconjugation. After it has been administered orally, the patient undergoes serial gamma scintigraphy to measure whole-body bile acid retention or, as suggested by some authors, bile acid retention in the gallbladder.134 A limitation of this test is that normal values for bile acid retention, which are used to compare normal and abnormal bile acid absorption, were obtained only in healthy persons without diarrhea.135 However, secondary bile acid malabsorption can be induced by diarrhea itself and is proportional to the stool weight, as demonstrated with the 14C-TCA test.131,133 For this test to be clinically useful, normal values need to be established for patients with diarrhea. Finally, this test is very time consuming, because bile acid retention needs to be measured either four or seven days (depending on the protocol) after the bile acid administration.
D-Xylose Test
Absorption of the pentose d-xylose is facilitated by passive diffusion. Approximately 50% of the absorbed d-xylose is metabolized, and the remainder is excreted in urine. After an overnight fast, a 25-g dose of d-xylose is swallowed, and the patient is encouraged to drink sufficient volumes of fluid to maintain good urine output. Urine is collected for the next five hours. As an alternative, one hour after ingestion of d-xylose, a venous sample may be obtained.136 Less than 4 grams (16% excretion) of d-xylose in the urine collection or a serum xylose concentration below 20 mg/dL indicates abnormal intestinal absorption. The traditional urine test appears to be more reliable than the one-hour blood test.
False-positive results occur if the duration of urine collection is too short or if the patient is dehydrated or has renal dysfunction, significant ascites, delayed gastric emptying, or portal hypertension. d-Xylose absorption may be normal in patients with only mild impairment of mucosal function or with predominantly distal small intestinal disease. Because d-xylose is susceptible to bacterial metabolism, absorption is diminished in patients with bacterial overgrowth, although the test has a poor sensitivity for detecting this.137 The test is of limited clinical value today and mostly has been replaced by small intestinal biopsy.
Intestinal Permeability Tests
Intestinal permeability tests mostly are used in studies of the pathophysiology of intestinal disorders; they do not provide a specific diagnosis.138
Most current permeability tests are based on the differential absorption of mono- and disaccharides. Damage to the mucosa can result in an increased permeability for disaccharides and oligosaccharides consequent to epithelial injury, and it can result in a decreased permeability of monosaccharides due to reduction of mucosal surface area.139 Absorption is measured by urinary excretion. Expression of results as the absorption ratio of the mono- and disaccharide minimizes the influences of gastric emptying, intestinal transit, renal and hepatic function, and variations in time of urine collections.140
Increased intestinal permeability has been shown to predict the development of Crohn’s disease or relapse in patients with this disease.141 In celiac disease, the finding of considerably increased permeability is a sensitive marker for advanced disease; permeability tests also have been used to judge response to a gluten-free diet142 or to screen first-degree relatives for celiac disease. Elevated serum aminotransferase levels in patients with celiac disease correlate with increased intestinal permeability.143 Disturbances of intestinal permeability have been documented in users of nonsteroidal anti-inflammatory drugs,144 in inflammatory joint disease,145 and in diabetic diarrhea.146
Carbon-13 Breath Tests
The increasing availability of stable isotopes has raised interest in replacing radioactive 14C with nonradioactive 13C for breath tests.111,147 In malabsorptive diseases, 13C-labeled substrates have been evaluated in the diagnosis of steatorrhea,148 small bowel bacterial overgrowth, and exocrine pancreatic insufficiency149 and in the evaluation of the digestibility of egg protein. Because of concerns about diagnostic accuracy, cost, and limited availability, these tests have not gained widespread acceptance.