Chapter 1 Macro and Micro Structure of the Lung*
Lung Development
The emergence of a normal, functioning respiratory system requires simultaneous development of the conducting airway system and the vascular system. Of interest, the mechanisms that drive this process also hold true for other branched-structure organ systems, such as the kidney and breast. Lung development, beginning with organogenesis, is divided into several stages, as indicated in Table 1-1. However, considerable overlap of the signaling cascades between the various stages is recognized.
Normal Lung Anatomy
As shown in Table 1-2 and Figure 1-1, A to D, the lungs are asymmetrically paired. The right lung is divided by major and minor fissures into three lobes: the upper, lower, and middle lobes. By contrast, the left lung has a single fissure dividing it into upper (superior) and lower (inferior) lobes. In the left lung, the homologue of the right lung’s middle lobe is the lingula, made up of the anterior and inferior portions of the upper lobe. In some persons there may be an incomplete fissure separating the lingula from the upper lobe. Bronchopulmonary segments are subunits of the lobes that derive from the first generation of bronchi below the lobar bronchi. These also are asymmetric between lungs; Table 1-1 shows the nomenclature.
Right Lung | Left Lung |
---|---|
Upper Lobe | Upper Lobe |
1. Apical | 1, 2. Apical-posterior |
2. Posterior | |
3. Anterior | 3. Anterior |
Middle Lobe | Lingula |
4. Lateral | 4. Superior |
5. Medial | 5. Inferior |
Lower Lobe | Lower Lobe |
6. Superior | 6. Superior |
7. Medial basal | |
8. Anterior basal | 8. Anterior basal |
9. Lateral basal | 9. Lateral basal |
10. Posterior basal | 10. Posterior basal |
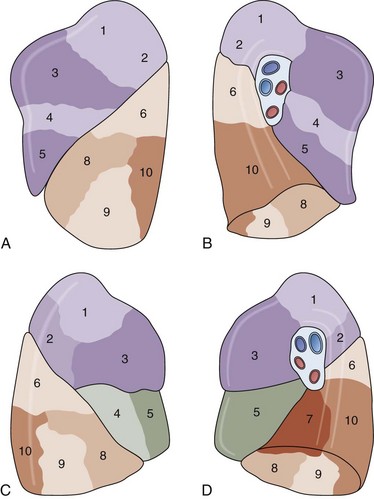
Figure 1-1 Lobes for left (A and B) and right (C and D) lungs. The numbered segments are identified in Table 1-2.
The next-smallest unit of the lung below the gross level of definition is the pulmonary lobule, also known as the secondary lobule of Miller. On the pleural surface, the secondary lobule is outlined by connective septa and has a roughly polygonal shape, measuring between 1 and 2 cm in diameter. Examination of the cut surface of lung again shows the interlobular septa demarcating the edges of the lobule (Figures 1-2 and 1-e1); these are best seen in the periphery, because interlobular septa are less well developed in the center of the lungs. Lobules also can be identified on microscopic examination (Figures 1-e2 and 1-e3). Each lobule contains three to five acini, which are the basic units of gas exchange. An acinus is defined as the lung parenchyma that derives from a single terminal membranous bronchiole and includes three successive generations of respiratory bronchioles and their subtending alveolar ducts, alveolar saccules, and alveoli. The terminal bronchioles branch near the center of the lobules, and their acini abut the interlobular septa. This relationship allows assessment of alterations in the lung parenchyma in terms of its location within the lung lobule (centrilobular, panlobular, paraseptal).
Airways
The bronchial system generally is divided into conducting and respiratory airways. The conducting airways extend from the trachea to the membranous bronchioles, and the architecture changes as the airways decrease in size. The trachea has a membranous posterior aspect composed of transverse smooth muscle bundles that together make up the trachealis muscle, with protective cartilaginous rings on the anterior aspect (Figure 1-e4). In the cartilaginous bronchi, the cartilage plates enclose but do not completely surround the airway, and the muscle fibers wind around the airway in a spiral. These airways are surrounded by loose connective tissue, which contains the bronchial arteries, venous trunks, lymphatics, and nerves (Figures 1-3 and 1-e5). In the upper airways, the epithelium is predominantly ciliated, with a modest number of goblet cells. The cells are supported by a thin basement membrane. The bronchial mucous glands lie deep to the epithelium, with the mucous gland pits emptying onto the surface of the airway (Figure 1-e6). The glands contain both serous and mucous cells, with myoepithelial cells, all enclosed within the basal lamina of the glands (Figure 1-e7).
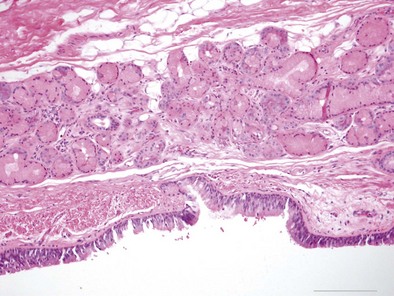
Figure 1-e6 Higher-power view of trachea from the same specimen as in Figure 1-e4 demonstrating the pseudostratified epithelium with goblet cells and the mixed seromucinous nature of the bronchial gland.
The noncartilaginous conducting airways extend from approximately generation 5 to generation 14 and are termed membranous bronchioles (Figure 1-4). The more proximal airways have a ciliated cell predominance (Figure 1-5), whereas the more distal airways acquire increasing numbers of nonciliated bronchiolar (Clara) cells until they account for approximately 11% of the total epithelial cell numbers in the terminal membranous bronchioles. Each bronchiole has a complete fibromuscular wall, with the muscle arranged in a tight spiral. An inconspicuous compartment located between the basement membrane and the muscle layer contains a few collagen fibers and longitudinally oriented elastic fibers. The bronchioles have an adventitial sheath into which adjacent alveoli insert.
The respiratory bronchioles have an incomplete fibromuscular wall, because they are partially alveolarized (Figure 1-6); the degree of alveolarization increases in each generation. The muscle bundles continue down the walls of the alveolar ducts, which are airways with completely alveolate walls, and end at the alveolar saccule entrance rings (Figure 1-e8).
Alveolus
The alveolus is the main gas exchange area of the lung and is composed of a thin epithelial layer supported by its basement membrane, a capillary endothelium supported by its own basement membrane, and the interstitium between the two basement membranes (Figures 1-7 and 1-e9). Where the two basement membranes are fused, the alveolus is optimized for gas exchange; this region is known as the “thin” portion of the blood-air barrier. In the “thick” portion, by contrast, the basement membranes are separate, and there are fibroblasts, collagen and elastin fibers, and contractile interstitial cells (myofibroblasts, pericytes, and smooth muscle cells).
The alveolus is penetrated by openings known as the pores of Kohn (Figure 1-8). These appear early in postnatal life and increase in number with age. A majority of the pores are filled by alveolar lining fluid, but their geometry is affected by lung volumes. They may be important in collateral ventilation or may represent an acquired degenerative lesion.
Alveolar Epithelial Cells
Most of the alveolar surface is covered by simple squamous cells known as type I pneumocytes (Figure 1-9). These cells have a small nucleus with highly branched cytoplasmic processes covering 4000 to 5000 µm2. The cytoplasm contains sparse organelles. Type I cells form by mitotic division and transformation of type II cells. The normal ratio of type I to type II cells is 1:2.
Type II epithelial cells have a granular appearance and, in humans, are noted to protrude into the alveolar space (Figure 1-10). They have abundant mitochondria, endoplasmic reticulum, and a large Golgi apparatus, in addition to conspicuous secretory granules, called lamellar bodies, which are composed of surfactant. Surfactant is approximately 90% lipid in nature, the major portion of which is phosphatidylcholine; surfactant apoproteins A, B, C, and D make up the remainder.
Vascular System
The main pulmonary artery splits into its two main branches within the mediastinum and beneath the aortic arch. The right pulmonary artery passes beneath the aortic arch and enters the lung anterior to the main bronchus. The left pulmonary artery travels above the main bronchus, passes over the superior lobar bronchus, and can then be identified posterior to the bronchus. The pulmonary arteries branch in company with the bronchi (see Figures 1-3 and 1-4) and can be identified down to the level of the tertiary respiratory bronchioles–alveolar ducts where they are small and poorly muscularized (see Figure 1-6). These precapillary vessels feed into the alveolar-capillary network, which consists of a gridlike mesh (Figure 1-11). The capillaries empty into the pulmonary veins, which travel a path independent of the bronchi, at the periphery of the acinus. When the interlobular septa are well formed, the veins lie within the septal fibrous tissue. At the hilus of each lung, the two pulmonary veins independently enter into the left atrium.
Burri PH. Structural aspects of postnatal lung development—alveolar formation and growth. Biol Neonate. 2006;89:313–322.
Cardoso WV, Kotton DN Specification and patterning of the respiratory system (July 16, 2008), StemBook. Cambridge, Mass, Harvard Stem Cell Institute (serial online) http://www.stembook.org accessed March 5, 2011
Lauweryns JM. The blood and lymphatic microcirculation of the lung. Pathol Annu. 1971;6:365–415.
Morrisey EE, Hogan BL. Preparing for the first breath: genetic and cellular mechanisms in lung development. Dev Cell. 2010;18:8–23.
Ochs M, Weibel ER. Functional design of the human lung for gas exchange. In: Fishman AP, Elias J, Fishman Jet al. Fishman’s pulmonary diseases and disorders. ed 4. New York: McGraw-Hill; 2008:23–70.
Parent RA. Comparative biology of the normal lung. Ann Arbor, Mich: CRC Press; 1992.
Pinkerton KE, Green FH. Normal aging of the lung. In: Harding R, Pinkerton KE, Plopper CG. The lung: development, aging, and the environment. New York: Academic Press; 2004:213–233.
Plopper CG, Hill LH, Mariassy AT. Ultrastructure of the nonciliated bronchiolar epithelial (Clara) cell of mammalian lung. III. A study of man with comparison of 15 mammalian species. Exp Lung Res. 1980;1:171–180.
Plopper CG, George J, Mariassy A, et al. Species differences in airway cell distribution and morphology. In: Crapo JD, Miller FJ. Extrapolation of dosimetric relationships for inhaled particles and gases. New York: Academic Press; 1989:19–34.