Chapter 4
Lung Volumes, Airway Resistance, and Gas Distribution Tests
1. Describe the measurement of lung volumes using gas dilution/washout methods.
2. Explain two advantages of measuring lung volumes using the body plethysmograph.
3. Calculate residual volume and total lung capacity from FRC and the subdivisions of VC.
4. Identify a restricted disease process from measured lung volumes.
5. Describe the measurement of airway resistance using the body plethysmograph.
1. Calculate FRC using helium dilution and nitrogen washout methods.
2. Describe the correct technique for measuring thoracic gas volume using body plethysmography.
3. Identify air trapping and hyperinflation using measured lung volumes.
4. Describe the differences between Raw, sGaw, and sRaw.
5. Identify uneven distribution of gas in the lungs by either single- or multiple-breath techniques.
closed-circuit, multiple-breath helium dilution (FRChe)
FRCpleth (FRC measured with the body plethysmograph)
functional residual capacity (FRC)
lung volume reduction surgery (LVRS)
open-circuit, multiple-breath nitrogen washout technique (FRCn2)
single-breath nitrogen washout test (SBN2)
specific airway conductance (sGaw)
Lung Volumes: Functional Residual Capacity, Residual Volume, Total Lung Capacity, and Residual Volume/Total Lung Capacity Ratio
Description
Functional residual capacity (FRC) is the volume of gas remaining in the lungs at the end of a quiet breath. On a simple spirogram, this point is termed the end-expiratory level (see Figure 2-1). Residual volume (RV) is the volume of gas remaining in the lungs at the end of a maximal expiration regardless of the lung volume at which exhalation was started (see Figure 2-1). Total lung capacity (TLC) is the volume of gas contained in the lungs after maximal inspiration. FRC, TLC, and RV are reported in liters (L) or milliliters (mL), corrected to BTPS. The RV/TLC ratio defines the fraction of TLC that cannot be exhaled (RV), expressed as a percentage.
Technique
There are a variety of methods for measuring absolute lung volumes (Table 4-1). FRC is measured directly with the open-circuit multiple-breath N2 washout, closed-circuit multiple-breath He dilution, and body plethysmographic techniques. Once FRC and VC have been measured, RV and TLC can be calculated. TLC can be estimated directly with the single-breath N2 washout, and single-breath He dilution as part of the diffusing capacity (Dlco) test, and by chest imaging techniques. RV can only be measured indirectly once FRC or TLC has been determined.
Table 4-1
Methods for Measurement of Lung Volumes
Method | Lung Volume | Advantages/Disadvantages |
Multiple-breath He dilution | FRC | Simple, relatively inexpensive; affected by distribution of ventilation in moderate or severe obstruction; multiple-breath; requires IC, ERV to calculate other lung volumes |
Multiple-breath N2 washout | FRC | Simple, relatively inexpensive; affected by distribution of ventilation in moderate or severe obstruction; multiple-breath; requires IC, ERV to calculate other lung volumes |
Single-breath N2 washout | TLC | Calculated from single-breath N2 distribution test; may underestimate lung volume in the presence of obstruction |
Single-breath He (or other inert gas; e.g., neon) dilution | TLC | Calculated as part of Dlco (VA); may underestimate lung volume in the presence of obstruction |
Plethysmography | VTG (FRCpleth) | Plethysmographic method more complex, but very fast; tends to be more accurate in the presence of airway obstruction than gas dilution techniques |
Chest radiograph | TLC | Requires posterior-anterior and lateral chest x-ray films; must breath-hold at TLC; not accurate in the presence of diffuse, space-occupying diseases |
Chest computerized tomography (CT) | TLC | Involves radiation exposure and increased cost; must breath-hold at TLC; underestimates lung volumes in the presence of airway obstruction |
Magnetic resonance imaging (MRI) | TLC | No radiation exposure; very costly; research tool only |
Dlco, Diffusing capacity; IC, inspiratory capacity; N2, nitrogen; VA, alveolar volume.
Open-Circuit, Multiple-Breath Nitrogen Washout

FEN2final = fraction of N2 in volume expired
FAN2alveolar1 = fraction of N2 in alveolar gas initially
FAN2alveolar2 = fraction of N2 in alveolar gas at end (from an alveolar sample)
N2tissue = volume of N2 washed out of blood/tissues
The original N2 washout technique lasted 7 minutes. However, not all of the N2 in the lungs may be washed out, even after 7 minutes of 100% O2 breathing. The FAn2alveolar2 is measured at the end of the test and subtracted from the initial N2 concentration. Correction for the “switch-in” error should also be made (see Correcting for the Switch-in Error). The final FRC is then corrected to BTPS, and volume of the equipment dead space (including filters) must be subtracted (see the Sample Calculations on Evolve at http://evolve.elsevier.com/Mottram/Ruppel/).

Some available commercial systems use a rapid N2 analyzer in combination with a spirometer to provide a “breath-by-breath” analysis of expired N2 (Figure 4-1, A). An alternative approach is to use fast-response O2 and carbon dioxide (CO2) analyzers to calculate the concentration of N2 in expired gas during the washout:
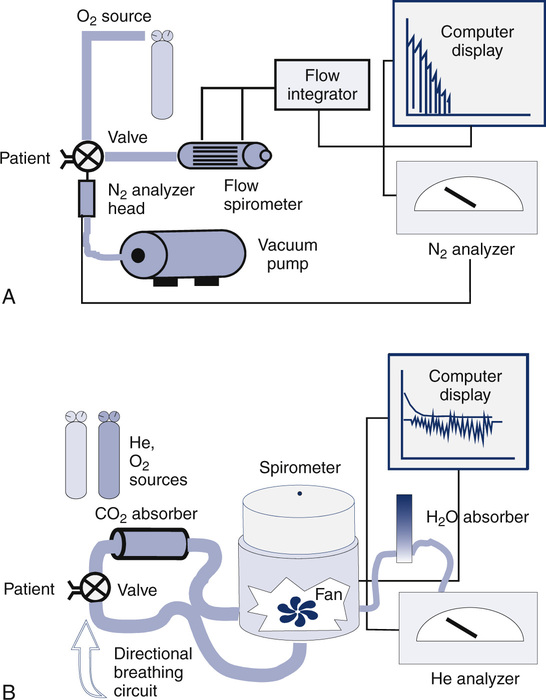
(A) Open-circuit equipment used for N2 washout determination of FRC. The patient inspires O2 from a regulated source and exhales past a rapidly responding N2 analyzer into a pneumotachometer. FRC is calculated from the total volume of N2 exhaled and the change in alveolar N2 from the beginning to the end of the test (Figure 4-2 and open-circuit method); (B) Closed-circuit equipment used for He dilution FRC determination includes a directional breathing circuit with a volume-based spirometer, He analyzer, CO2 absorber, O2 source, and water absorber. A breathing valve near the mouth allows the patient to be “switched in” to the system after He has been added and the system volume determined. Tidal breathing and the He dilution curve are displayed on the computer.

FEo2 = fraction of O2 in expired gas (dry)
FEco2 = fraction of CO2 in expired gas (dry)
In these fast methods, the patient, wearing a noseclip, breathes through a mouthpiece-valve system. Precisely at end-expiration, a valve is opened to allow 100% O2 breathing to begin. Each breath of 100% O2 washes out some of the residual N2 in the lungs. Analog signals proportional to N2 concentration and volume (or flow) are integrated to derive the volume of N2 exhaled for each breath. Values for each breath are summed to provide a total volume of N2 washed out (Figure 4-2). The test is continued until the N2 in alveolar gas has been reduced to approximately 1% (Criteria for Acceptability 4-1). Some older systems terminate the test at 7 minutes. However, the O2 breathing should be continued until alveolar N2 falls to less than 1.5% for at least three consecutive breaths. A change in inspired N2 concentration of greater than 1% or sudden large increases in expiratory N2 concentrations indicate a leak, in which case the test should be stopped and repeated.
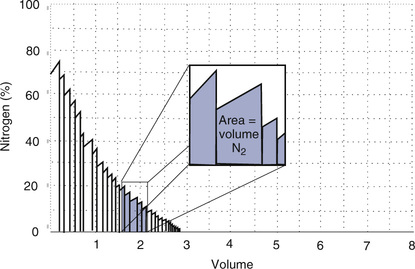
The concentration (or log concentration) of N2 is plotted against time or against the volume expired as the patient breathes through a circuit (Figure 4-1, A). The volume of N2 expired with each breath is measured by integrating flow and N2 concentration to determine the area under each curve (see inset). FRC is determined by dividing the volume of N2 expired by the change in alveolar N2 from the beginning to the end of the test, with corrections, as described in the text.
accomplished by software or electronic correction of the analyzer output.
Closed-Circuit, Multiple-Breath Helium Dilution
FRC can also be determined by equilibrating the gas in the lungs with a known volume of gas containing He (closed-circuit, multiple-breath helium dilution [FRChe]). A spirometer is filled with a known volume of air, and then a volume of He is added so that a concentration of approximately 10% is achieved (see Figure 4-1, B). The exact concentration of He and spirometer volume is measured and recorded before the test is begun. The patient breathes through a valve that allows a connection to a rebreathing system. The valve is opened at the end of a quiet breath (i.e., the end-expiratory level). Then the patient rebreathes the gas in the spirometer, with a CO2 absorber in place, until the concentration of He falls to a stable level (Figure 4-3). A fan or blower mixes the gas within the spirometer system. O2 is added to the spirometer system to maintain the Fio2 near or above 0.21 and to keep the system volume relatively constant.
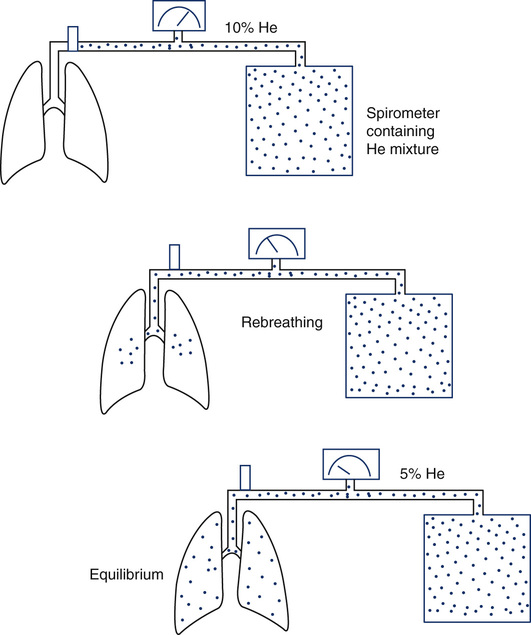
At the beginning of the test, the patient’s lungs contain no He. The spirometer contains a known concentration of He in a known volume (see text). The patient then rebreathes the He mixture from this system (Figure 4-1, B). He is diluted until equilibrium is reached. At the end of the test, the known volume of He has been diluted in the rebreathing system and the lungs. FRC is calculated from the change in He concentration and the known system volume. The patient must be switched from breathing air to the He mixture at the end-expiratory level for accurate measurement of FRC. RV is derived by subtracting the ERV. (Modified from Comroe JH Jr, Forster RE, Dubois AB, et al. The lung: Clinical physiology and pulmonary function tests. 2nd ed. St. Louis: Mosby; 1962.)
Equilibration between normal lungs and the rebreathing system takes place in approximately 3 minutes when a 10% He mixture in a system volume of 6–8 L is used (Figure 4-4). The final concentration of He is then recorded. The system volume is computed first. System volume is the volume of the spirometer, breathing circuitry, and valves before the patient is connected. It can be calculated as follows:
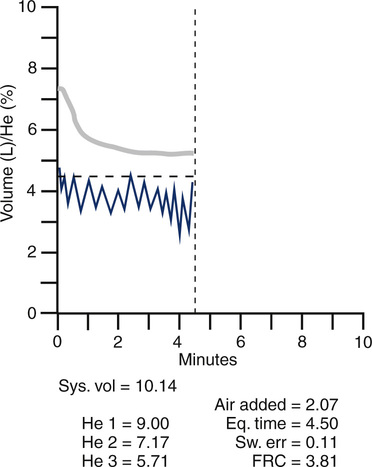
Graph shows He concentration from the beginning of rebreathing until equilibrium is achieved (upper line). System volume of the spirometer and the patient’s tidal breathing is also shown (lower line). A CO2 absorber removes carbon dioxide produced by the patient. A computerized valve system replaces O2 to keep the system volume constant. Measurements of He concentrations and system variables are also displayed.

Headded = volume of He placed in the spirometer in liters (L)
Fhe initial = % He converted to a fraction (%He/100)
When the system volume is known, FRC can be computed as follows:

Some automated systems use a similar method to calculate the system volume; a small amount of He is added to the closed system, followed by a known volume of air. The change in He concentration after the addition of the air is used to determine the system volume. Rebreathing is continued until the He concentration changes by no more than 0.02% in 30 seconds (Criteria for Acceptability 4-2).
Additional Comments on FRC by Gas Dilution Techniques
In either of the gas dilution techniques, a leak will cause erroneous estimates of FRC. Leaks may occur in breathing valves or circuitry, or at the patient connection. Some patients have difficulty maintaining an adequate seal at the mouthpiece throughout the test. Failure to properly apply noseclips can also result in a leak. Leaks usually result in an overestimate of lung volume. A leak in the open-circuit N2 washout system allows room air to enter, increasing the volume of N2 washed out. A leak in the closed-circuit He dilution system allows air to dilute the He concentration or He to escape. Each situation causes the test gas concentration to change more than it should. Leaks during the N2 washout can usually be identified by an inspection of the graphic display or recording (Figure 4-5). Inaccuracy or malfunction of the gas analyzers in either method often causes errors. Leaks or analyzer problems should be considered whenever FRC values are inconsistent with spirometry results (See Interpretive Strategies Box 4-1).
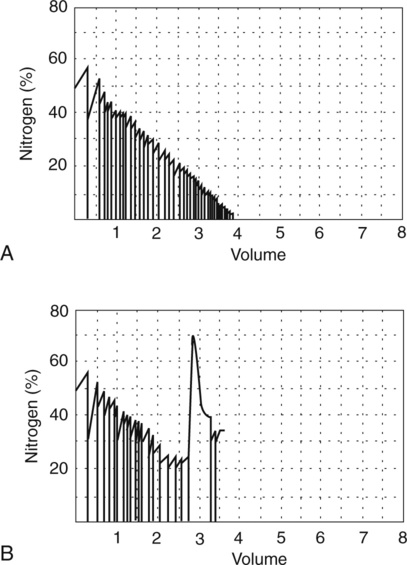
(A) A computer-generated recording of a nitrogen (N2) washout test in a healthy patient. The tracings show a continuous decrease in end-tidal N2 concentration with successive breaths. The test is continued until the N2 concentration falls to less than 1%. (B) A similar plot of N2 washout from a healthy patient, but, in this instance, a leak occurs during the test. Leaks may occur if the patient does not maintain a tight seal at the mouthpiece. Leaks are usually easy to detect because room air enters the system and causes an abrupt increase in N2 concentration.
Body Plethysmography
FRC measured with the body plethysmograph (FRCpleth) (Figure 4-6) refers to the volume of intrathorax gas measured when airflow occlusion occurs at end-expiration (FRC) during shutter closure. The technique is based on Boyle’s law relating pressure to volume. A volume of gas varies in inverse proportion to the pressure to which it is subjected if the temperature remains constant (isothermal). The patient has an unknown volume of gas in the thorax at the end of a normal expiration (i.e., the FRC). The airway is occluded momentarily at or near FRC; the patient is asked to gently pant at a frequency between 0.5 and 1.0 Hz (0.5–1.0 cycles per second), allowing the air in the chest to be compressed and decompressed. This causes a change in volume and pressure. The changes in pressure are easily measured at the mouth (Pmouth) with a pressure transducer. Mouth pressure theoretically equals alveolar pressure when there is no airflow. Changes in pulmonary gas volume are estimated by measuring pressure changes in the plethysmograph. The pressure in the plethysmograph is measured by a sensitive transducer. This transducer is calibrated by introducing a small, known volume of gas into the sealed box and relating the pressure change to the known volume (Pbox). The calibration factor is then applied to measurements made on human patients.
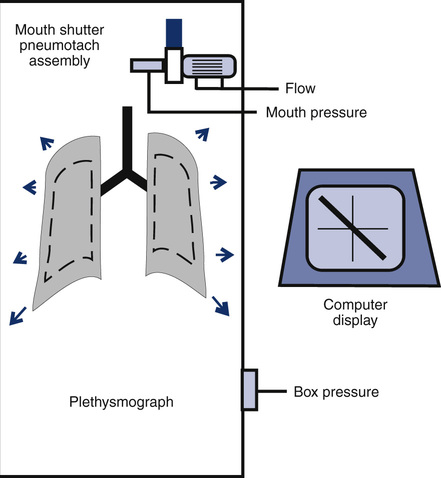
Boyle’s law states that volume varies inversely with pressure if temperature is held constant. A pressure-type (constant volume) plethysmograph, with pressure transducers for measurements of box pressure and mouth (alveolar) pressure, is shown. A pneumotachometer measures flow to track lung volumes. The mouth shutter occludes the airway momentarily so alveolar pressure can be estimated. The patient pants gently against the closed shutter. Gas in the lungs is alternately compressed and decompressed. Changes in lung volume are reflected by changes in box pressure. These changes are displayed as a sloping line on a computer display. When the original pressure (P), the new pressure (P +ΔP), and the new volume (V +ΔV) are known, the original volume (V or VTG) can be computed from Boyle’s law (see the section on the technique of thoracic gas volume and see Evolve at http://evolve.elsevier.com/Mottram/Ruppel/).
The display of the panting maneuver is a graph with Pmouth and Pbox. Pmouth is plotted on the vertical axis, and Pbox is plotted on the horizontal axis (Figure 4-7). The resulting figure appears as a sloping line equal to DP/DV, where DP equals change in alveolar pressure and DV equals change in alveolar volume. Change in alveolar volume is measured indirectly by noting the reciprocal change in plethysmograph volume.
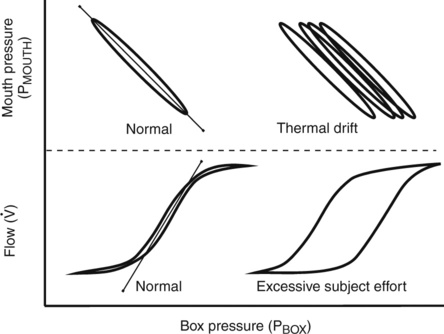
Top left, Normal closed-shutter maneuver in which mouth pressure is plotted against box pressure. The loop should be closed, or nearly so. If thermal equilibrium has not been achieved, the loop (shown on the top right) tends to be open and to drift across the screen. Bottom left, Normal open-shutter measurement in which flow is plotted against box pressure. If the patient pants near FRC, the loop takes on a nearly closed S-shaped appearance. If the patient pants too rapidly or too deeply, the tracing becomes open and flattened (shown on the bottom right). Thermal drift can also cause the open-shutter tracing to resemble excessive patient effort.
The VTG can then be obtained from the slope of the tracing by applying a derivation of Boyle’s law:

PB = barometric pressure minus water vapor pressure
λVTG = slope of the displayed line equal to DP/DV
Pboxcal = box pressure transducer calibration factor
Pmouthcal = mouth pressure transducer calibration factor
K = correction factor for volume displaced by the patient
For the complete derivation of the equation and sample calculations, see Evolve (http://evolve.elsevier.com/Mottram/Ruppel/).
lung volume can be monitored by continuously integrating the flow through the plethysmograph’s pneumotachometer. The end-expiratory level can be determined from tidal breathing. The patient then pants with the mouth shutter open. The computer records the change in lung volume above or below the resting level (FRC). When asked to pant, most patients do so slightly above FRC. The shutter then closes automatically, the patient continues panting, and VTG is measured as described. The computer then adds or subtracts the change in volume from the end-expiratory level (before panting began) to calculate the true FRC. This computerized technique allows the patient to pant at the correct frequency and depth before the shutter is closed. It also eliminates the necessity of closing the shutter precisely at end-expiration. Raw and sGaw can also be measured simultaneously during the open-shutter panting (see following text). It should be noted that the correct panting frequencies for measuring Raw and VTG are slightly different. Raw measurements should be made with the patient panting at about 1.5–2.5 Hz (1.5–2.5 cycles per second, or 90–150 breaths/min), whereas VTG should be measured with the patient panting at a slower rate of 0.5–1 Hz. Many computerized systems display the panting frequency so the technologist can coach the patient to achieve the correct rate.
Depending on the construction of the plethysmograph, venting to the atmosphere is usually required to establish thermal equilibrium. Equilibrium takes about 30-60 sec and can be presumed when the flow-volume recording stabilizes (i.e., does not drift). The patient is then instructed to pant. When the correct panting frequency and depth have been established, the shutter may be closed. Some plethysmograph systems require tidal breathing before shutter closure to establish the patient’s end-expiratory level. In either type of system, the patient should pant against the closed shutter until a stable tracing is produced. Two to four pants are usually sufficient. If the patient pants too hard, the tracing may drift or appear as an “open” loop (see Figure 4-7). The recorded pressure changes should be within the range over which the transducers were calibrated. The entire tracing should be visible on the display. If the tracing goes off-screen, the pressure changes probably exceed the calibration ranges. Three or more technically satisfactory maneuvers should be recorded, each followed by ERV and IVC maneuvers (Criteria for Acceptability 4-3). At least three FRCpleth values that agree within 5% should be obtained and the mean value reported. The technologist’s comments should note the acceptability of the maneuvers. Most computerized plethysmographs automatically measure the slope of the DP/DV tracings. This is done by using the least-squares method to calculate a “best-fit” line through the recorded data points. The technologist may need to correct computer-generated tangents, depending on data quality from the panting maneuvers.
Additional Comments on FRC by Plethysmography
The plethysmographic method is a quick and accurate means of measuring lung volumes. It can be used in combination with simple spirometry to derive all lung volume compartments. The plethysmograph’s primary advantage is that it measures all gas in the thorax, whether in ventilatory communication with the atmosphere or not. The plethysmographic measurement of FRC is often larger than that measured by He dilution or N2 washout. This is the case in emphysema and other diseases characterized by air trapping, as well as in the presence of an uneven distribution of ventilation. When gas dilution tests are continued for more than 7 minutes, the results for FRC determinations approach the VTG value. See Interpretive Strategies 4-2.