37 Long-Term Complications and Management
Most patients who have undergone cardiac surgical procedures have brief stays in the intensive care unit (ICU; < 24 hours), and these stays typically follow a predictable pattern. During this time, most instability and morbidity are attributable to the cardiopulmonary organ systems, bleeding, hypothermia, and the emergence from anesthesia.1 A small minority of patients, however, have prolonged ICU stays, characterized by multisystem complications involving both the cardiac and noncardiac systems. This group of patients consumes a disproportionate number of ICU resources; generates enormous hospital costs; and, ultimately, has a much worse prognosis (both in-hospital and long term).1–3 For example, Bashour et al1 described a series of 2618 patients who had undergone cardiac operations, 5.4% of whom had an ICU length of stay (LOS) of longer than 10 days. In the prolonged LOS group, the in-hospital mortality rate was 33.1% (vs. 1.5% in a group with an LOS < 10 days). Of those patients with a prolonged ICU LOS who survived to discharge, 46.8% died within 30 months. Welsby et al2 analyzed types of complications in a cohort of patients who had undergone cardiac surgical procedures and who had a prolonged LOS in the ICU; they demonstrated that noncardiac complications are more deleterious than are isolated cardiac complications. In another series of 1280 patients, only 3.8% of patients had an ICU LOS longer than 14 days. The hospital mortality rate in the prolonged LOS group was 25.8% (vs. 5.3% in a short LOS group), and only 62% of patients discharged after an extended stay in the ICU were alive at 2 years.3
Sedation in the intensive care unit
Most patients who have an extended LOS in the ICU will require a different approach to sedation and pain control than do patients who do not have an extended LOS. The major goals of sedation in the ICU are to provide anxiolysis and to improve the patient’s perceptual experience during this physiologically and emotionally stressful period (Box 37-1).4 Secondarily, sedation reduces the physiologic stress response and attendant cardiovascular work, may facilitate the maintenance of circadian rhythms, and lessens delirium and agitation.5 These goals are distinct from those associated with analgesia, which are the alleviation of pain through nonpharmacologic and pharmacologic means and to facilitate diagnostic and therapeutic procedures.4 Although sedation and analgesia are separate therapeutic goals usually provided by individual drugs, combining anxiolytic and analgesic drugs often results in a synergistic effect, and some newer agents provide elements of both analgesia and anxiolysis, thus blurring the distinction in clinical practice (see Chapter 38).
BOX 37-1 Sedation Goals
In 1995, the Society of Critical Care Medicine (SCCM) published guidelines for sedation in the ICU,6 revised the guidelines in 2002,7 and is in the process of again updating the guidelines (Michael J. Murray, MD, PhD, Personal Communication, December 2010). The guidelines emphasize the need for the goal-directed delivery of psychoactive medications. Using goal-directed sedation is supported by an increasing body of literature that shows that daily interruption of sedation, intermittent sedation, and sedation protocols all reduce the duration of mechanical ventilation and, in some instances, decrease ICU LOS.8

Sedation Scoring Systems
Several scoring systems are available to assess a patient’s degree of sedation in the ICU and to facilitate goal-directed therapy. When using the seven-item Riker Sedation-Agitation Scale,9 which was the first scale proved to be reliable and valid for use in critically ill adults, the clinician assigns a score based on the patient’s behavior. The Motor Activity Assessment Scale10 includes seven categories to describe patients’ behavior in response to stimulation. Like the Sedation-Agitation Scale, it has been validated in critically ill adults.
Most comparative clinical studies of sedation in critically ill patients have used the Ramsay Scale.11 Scoring with this six-point scale of motor activity ranges from 1 (patient anxious, agitated or restless, or both) to 6 (no response to light glabellar tap or loud auditory stimulus; Table 37-1).5 Originally designed as a research tool, the Ramsay Scale has been used for decades in clinical practice.
The patient |
Other sedation scales that have been validated in critically ill adults include the Vancouver Interaction and Calmness Scale,12 the COMFORT scale,13 the Richmond Agitation-Sedation Scale,14,15 and the Minnesota Sedation Assessment Tool.16 Increasing recognition of the long-term sequelae of delirium has led to the development and use of other scoring systems. The Confusion Assessment Method for the ICU (CAM-ICU) recently was validated in one ICU study and it is increasingly being used.17
More objective parameters to assess sedation are being pursued. The bispectral index is a monitor that measures a simple, three-lead, frontal-montage that, through proprietary software, converts the electroencephalographic activity to a digital scale from 1 to 100. When specific sedative agents are used, the bispectral index reading typically correlates well with the level of sedation in patients in the ICU18 (see Chapter 16).

Sedative Agents
Benzodiazepines
Midazolam, a short-acting, water-soluble benzodiazepine, can only be given parenterally. Its intravenous administration causes no pain or venous irritation (and, therefore, thrombosis), and its potency is two to four times that of diazepam. Midazolam is readily redistributed in tissues and is rapidly cleared by the liver and kidneys. It is enzymatically degraded in the liver to α-hydroxy-midazolam, which has minimal, if any, clinical sedative or hypnotic effects. The clinical effects of midazolam are short-lived because of an elimination half-life of 1.5 to 3.5 hours. These properties make midazolam ideal as an anxiolytic benzodiazepine for short-term use in the ICU.19 Depending on the situation, intermittent boluses of midazolam can be given, or a continuous infusion of 0.5 to 5.0 mg/hr can be used. Greater doses may be required, and infusions of up to 20 mg/hr have been used safely in mechanically ventilated patients.20
Lorazepam is a long-acting agent with sedative effects that last 6 to 8 hours after a single dose. Lorazepam has minimal effect on cardiovascular and respiratory function and can be given orally or parenterally.21 Because its potency is two to four times that of midazolam, the dose must be adjusted accordingly. Because of the long half-life of lorazepam and despite the fact that its time of onset of action is considerably longer than that of midazolam, the use of lorazepam is preferred to midazolam for patients requiring long-term sedation.6 Because of different volumes of distribution, elimination half-lives, and so on, especially in patients with multiple organ dysfunction syndrome and in critically ill patients who require benzodiazepines for longer than 24 hours, lorazepam has a clinical half-life similar to that of midazolam.22
If a benzodiazepine is used to manage the sedation of patients in the ICU, the benzodiazepine antagonist, flumazenil, should be readily available.23 Flumazenil is a highly specific benzodiazepine antagonist that reverses all known central nervous system effects of the benzodiazepines. It reaches maximum concentration in the brain within 5 to 10 minutes after intravenous administration. The mean terminal half-life of flumazenil is approximately 1 hour. It is completely metabolized to free carboxylic acid and glucuronide, both of which are inactive metabolites and renally excreted.
Propofol
Propofol, an intravenously administered alkyl-phenol anesthetic agent that is chemically unrelated to other anesthetic agents, has been used to provide sedation for patients in ICUs and is the preferred drug for “fast-tracking” patients. Because it is so hydrophobic, it is formulated in a lipid emulsion (10% Intralipid), which must be taken into account when it is administered to patients. It is short-acting and rapidly redistributed and metabolized, making it suitable for use as a continuous infusion. Because it allows for a rapid recovery and has a favorable side-effect profile, propofol often is used not only to sedate patients but also for use in certain procedures such as cardioversions, chest tube insertions or withdrawal, and pleurodesis (see Chapter 9).
Compared with midazolam, propofol allows for more rapid weaning of patients from mechanical ventilation; because of this property, propofol is used more commonly for fast-tracking patients after cardiac operations.24 When used for sedation, propofol should be administered as an initial dose of 0.5 to 1.0 mg/kg followed by an infusion of approximately 25 to 100 μg/kg/min. Because case reports have recounted mortality in patients who received excessively high doses of propofol, the maximum dose should probably be less than 100 μg/kg/min.23,25 Propofol infusion syndrome has been reported to occur in patients who have received propofol for a very short period, indicating that this may be an idiosyncratic reaction.26
When given by bolus administration, propofol may cause a decrease in mean arterial pressure because of peripheral vasodilation, not direct myocardial depression. Reports have been published of death with the use of propofol in both adults and children.27 The U.S. Food and Drug Administration requires the following boxed warning on product labeling for propofol, “While causality has not been established, Diprivan injectable emulsion is not indicated for sedation in pediatric patients until further studies have been performed to document its safety in that population.”
Myoclonic activity versus frank seizures has been reported to occur in patients receiving propofol,28 and individuals have become chemically dependent on propofol. These side effects, however, are rare (with the exception of the hypotension after bolus infusion) and should not limit the use of propofol in patients after cardiac surgical procedures, particularly given the effectiveness of propofol in fast-tracked patients. Studies have specifically addressed the issue of sedation in patients after cardiac operations; most of the studies compared midazolam with propofol and, in general, showed no specific advantage of one agent over another.29,30
Dexmedetomidine
Significant interest has been shown in using the α2-receptor agonist dexmedetomidine for postoperative cardiac sedation. α2-Receptor agonists bind to noradrenergic receptors in the brain, spinal cord, and elsewhere in the body. The benefit with the use of these agents is that sedation is provided with supplemental analgesic effects without respiratory depression. Dexmedetomidine was compared with benzodiazepines for the sedation of all groups of critically ill patients in four separate randomized, controlled trials.9,31–33 The findings of the Safety and Efficacy of Dexmedetomidine Compared with Midazolam (SEDCOM) study group9 revealed that dexmedetomidine-treated patients experienced less time on mechanical ventilation, less tachycardia, and less hypertension, with the most common adverse effect being bradycardia. The Maximizing Efficacy of Targeted Sedation and Reducing Neurological Dysfunction (MENDS) group showed similar findings, with a longer duration of delirium for those treated with lorazepam, as compared with patients treated with dexmedetomidine.33
The results of trials specific to patients after cardiac surgery have not been as strong. Dexmedetomidine was used in a fast-track setting without a shorter duration on the ventilator, compared with opioids, at a $50-per-patient greater cost.31 The dexmedetomidine-treated group did have a lower incidence of postoperative delirium and reduction in opioid requirements.31 Herr et al32 conducted a multicenter trial comparing dexmedetomidine and propofol for sedation after coronary artery bypass grafting. They found no significant difference between groups in time to extubation, but the dexmedetomidine-treated patients required significantly fewer supplemental analgesics, antiemetics, epinephrine, and diuretics. In a more recent multicenter study of 356 patients in the ICU, the overall costs associated with the use of dexmedetomidine, compared with midazolam, were lower because the dexmedetomidine-treated patients had a shorter LOS in the ICU.34 After examining the literature applying to all critical care patients, not only those after cardiac operations, the committee that developed the SCCM sedation guidelines made multiple recommendations (Table 37-2; these recommendations predate the introduction of dexmedetomidine).7
TABLE 37-2 Society for Critical Care Medicine Sedation Guidelines

Neuromuscular Blocking Agents
Nondepolarizing NMBAs are divided into two classes: benzylisoquinolinium compounds and the aminosteroid compounds. Both classes of drugs can be used to chemically paralyze a patient in the ICU, which occasionally is required to intubate the trachea of a patient with acute respiratory failure.35 Patients in the ICU who are receiving NMBAs must be mechanically ventilated; they typically have acute lung injury or acute respiratory distress syndrome that requires mechanical ventilation but that cannot be appropriately managed without the use of an NMBA.36
If NMBAs are used, it cannot be overemphasized that the patient must be adequately sedated before the initiation of the NMBA—it has been several decades since reports first appeared of chemically paralyzed awake patients,37 but it is a situation that all individuals working in an ICU must strive to avoid. Once an adequate degree of sedation (usually to include an analgesic medication such as an opioid) is achieved, the patient is administered a bolus and then a continuous infusion of an NMBA. Although several NMBAs are available, the drugs most commonly used in the ICU are the aminosteroid compounds (e.g., rocuronium) and the benzylisoquinolinium compounds (e.g., cisatracurium). Because these drugs are infused continuously, the duration of action is not as important as if they were given as a single bolus, but duration of action does become of consequence when the medication is discontinued and the physician is assessing the return of the patient’s neuromuscular function. When infusing these medications, a twitch monitor should be used, and the physician should strive to achieve a train-of-4 of one or two twitches.38 If no twitches are observed, the patient may have received an overdose of medication and may be at risk for development of acute quadriplegic myopathy syndrome (AQMS), a situation that develops in patients receiving NMBAs in which, when the medication is discontinued, the patient remains flaccid for much longer than would be predicted simply based on the pharmacokinetics of the medications that were infused.39 The cause of this syndrome is unknown but is most likely secondary to the destruction of myosin by the NMBA or one of its metabolites. Differentiating between AQMS and critical illness polyneuropathy often is difficult, but in the latter, profound muscle necrosis—as is seen with AQMS—would not be expected to occur. Bolus administration of NMBAs, when tolerated, is advantageous for monitoring the effects of sedation and analgesia, as well as decreasing the incidence of tachyphylaxis.38
Another way to minimize the incidence of AQMS is to institute a daily drug holiday. Not only is this beneficial in decreasing the incidence of AQMS, but in patients receiving opioids and benzodiazepines, the incidence of drug withdrawal also decreases. When using NMBAs in the ICU, following the algorithm listed in Figure 37-1 is recommended.38
Infections in patients in the intensive care unit
Microbiologic infections are some of the most common and frequently serious secondary complications that plague patients in the ICU.40 The following is a brief review of the most frequent infectious complications occurring in critically ill patients after cardiothoracic operations (Box 37-2). Pneumonia and acute respiratory distress syndrome are reviewed in Chapter 35.

Intravascular Device–Related Infections
Virtually all adult patients having cardiac operations are monitored with invasive intravascular devices (IVDs), such as arterial, central venous, and pulmonary artery catheters (see Chapter 14). Unfortunately, patients who have IVDs in place frequently acquire bloodstream infections (BSIs). IVD-related BSIs are associated with an attributable mortality rate of 12% to 15%, prolonged hospitalization (mean of 7 days), and an increased hospital cost of approximately $35,000.41,42
Approximately 90% of all IVD-related BSIs occur with the short-term use of IVDs.43 IVDs that are present for a short period are most commonly colonized from the skin surrounding the insertion site.44,45 Organisms migrate along the external surface of the catheter and then the intercutaneous and subcutaneous segments, leading to colonization of the intravascular segment of the catheter.46,47 Once the catheter is colonized, it is difficult to eradicate organisms from the intravascular segment without catheter removal because the microbes adhere to and are covered by either a biofilm layer that they produce or the thrombin layer that the host forms on the device.48 Because the skin is the most common site of colonization, coagulase-negative staphylococci and Staphylococcus aureus from the host’s skin and the hands of hospital personnel caring for the patient are the most common infecting pathogens.49 However, with the long-term use of IVDs, contamination of the catheter hub also contributes to intraluminal colonization.46,47
Several factors have been associated with an increased risk for patients acquiring IVD-related bacteremia. These factors include site of insertion (femoral > internal jugular > subclavian), number of lumens (multiple > single), duration of catheter in situ, established infection elsewhere in the body, the presence of bacteremia, and the experience of the personnel placing the catheter. Although some practitioners support the use of peripherally inserted IVDs, the risk for patients acquiring BSIs approaches that of patients with short centrally placed catheters.50 In an effort to reduce the incidence of IVD-related BSIs, a Centers for Disease Control and Prevention advisory committee formulated pertinent evidence-based guidelines51:
These guidelines are summarized in Box 37-3.
BOX 37-3 Central Venous Catheter Management
The diagnosis of IVD-related BSI can be challenging. The diagnosis should be suspected in patients with evidence of infection (e.g., fever, leukocytosis, positive blood cultures) when another source is not evident. Careful inspection of the catheter site is warranted; the presence of exit-site erythema or purulence strongly supports the diagnosis. If the patient has no visible signs of infection, clinical suspicion and supporting data must be used to guide therapy. The most frequently used technique to culture IVDs is the semiquantitative roll-plate technique. With this technique, the most common threshold to define colonization is growth of at least 15 colony-forming units (cfu).48,52 Siegman-Igra and colleagues49 and others53,54 have shown that quantitative culture of sonicated catheters is superior to the roll-plate technique. However, because these techniques require catheter removal, paired concomitant qualitative blood cultures are drawn through the device and percutaneously. IVD-related BSI is diagnosed if both cultures are positive for the presence of microorganisms and the concentration of microorganisms from the device is twofold to fivefold greater than the concentration from the peripherally drawn culture.55
The first clinical decision to make when managing a suspected IVD-related BSI is to remove the catheter or leave it in place. This decision is influenced by whether the risk for IVD-related BSI is low, intermediate, or high. Risk, in turn, is determined by the infecting organism and whether the IVD-related BSI is complicated or uncomplicated. Complicated infections are those associated with shock; the persistence of positive blood cultures for longer than 48 hours after appropriate antibiotics are administered; and IVD-related BSIs associated with septic thrombosis, septic emboli, or deep-seated infections (e.g., endocarditis or a tunnel or port-pocket infection46,47; Figure 37-2). A low-risk IVD-related BSI is caused by organisms of low virulence (e.g., coagulase-negative staphylococci) in a patient without complications of infection. A moderate-risk IVD-related BSI is characterized by uncomplicated infections with moderate to highly virulent organisms (S. aureus, Candida species). A high-risk IVD-related BSI is a complicated IVD-related BSI.
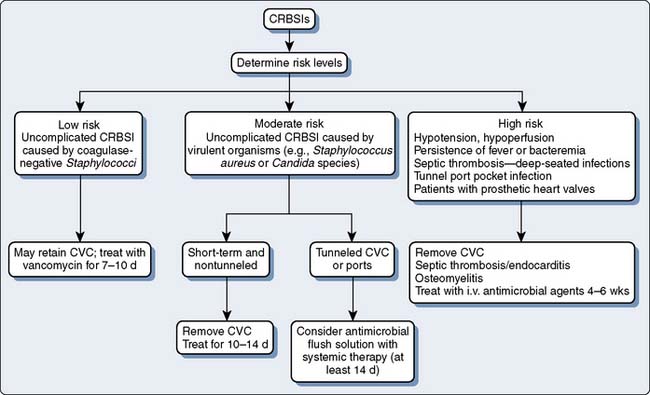
Figure 37-2 Algorithm for the management of intravascular catheter-related bloodstream infections (CRBSIs).
(Redrawn from Raad II, Hanna HA: Intravascular catheter-related infections. New horizons and recent advances. Arch Intern Med 162:871, 2002, by permission of the American Medical Association.)
Low-risk IVD-related BSIs can be treated without catheter removal.46,47 However, catheters should be removed in low-risk patients with prosthetic heart valves. In intermediate-risk patients, the catheters should be removed and the patients treated with a 10- to 14-day course of antibiotics. In high-risk patients, catheters should be removed and the duration of antibiotic use should be based on the nature of the complication. In deep-seated infections, such as septic thrombosis or endocarditis, antimicrobials should be administered for 4 to 6 weeks.56

Sternal Wound Infections
Deep and superficial surgical site infections occur infrequently but are morbid complications after cardiac operations, with an incidence rate of approximately 1% to 4%.57 Deep sternal infections are defined as those infections involving muscle and fascial layers, any other organ spaces manipulated during the operation, or organ involvement.58 They are associated with a 250% greater mortality rate, as compared with matched individuals without infection, and postoperative wound infections double the length of hospitalization.59 A host of preoperative, intraoperative, and postoperative risk factors have been identified for chest wall infections (Table 37-3).57–63
Host Factors | Surgical Factors | Postoperative Factors |
---|---|---|
Obesity, diabetes mellitus, hyperglycemia, use of internal mammary artery grafts (especially bilateral), advanced age, male sex, COPD, smoking, prolonged mechanical ventilation, steroids, preoperative hospital stay longer than 5 days | Duration of surgery and bypass, use of IABP balloon pump, postoperative bleeding, reoperation, sternal rewiring, extensive electrocautery, shaving with razors, and use of bone wax | Postoperative bleeding, prolonged ventilation, chest re-exploration, blood transfusion, and use of an IABP |
COPD, chronic obstructive pulmonary disease; IABP, intra-aortic balloon pump.
The diagnosis of sternal infections is based on the presence of wound tenderness, drainage, cellulitis, fever, leukocytosis, and sternal instability.60 S. aureus and coagulase-negative staphylococci account for approximately 50% of the organisms causing sternal wound infections after coronary artery bypass graft procedures.58 Several preventive strategies have been proposed to reduce the rates of surgical-site infection after cardiac operations. Martorell et al64 reported a reduction in the incidence rate of chest wall infections from greater than 8% to less than 2% after an intensive surveillance and intervention program that included the nasal application of mupirocin and having the patient shower before surgery with chlorhexidine. Other variables that are being investigated to reduce infection rates include perioperative antibiotic timing and redosing, adequacy of glycemic control, perioperative temperature control, and conservative transfusion protocols.65 The treatment of mediastinitis involves the prompt institution of antibiotics (empirically covering staphylococci species before obtaining culture results), debridement, open packing, and frequent dressing changes. On resolution, the chest is closed by primary closure or flap transposition in patients with large chest wall defects.60

Prosthetic Valve Endocarditis
Prosthetic valve endocarditis (PVE)—the infection of a prosthetic heart valve, the surrounding cardiac tissues, or both the valve and surrounding tissue—is a rare but serious source of infection in patients after cardiac surgery.65 The incidence rate of PVE is between 0.3% and 0.8% after valve-replacement operations.66–70 S. aureus is the most common organism related to PVE; timing of infection can be helpful to illustrate the pathophysiology and causative pathogen. PVE cases can be clustered into two groups according to the time of infection. In early PVE (within 2 months of valve implantation), the valve and sewing ring have not yet endothelialized; hence microorganisms frequently invade the surrounding tissue planes, causing perivalvular abscess and perivalvular leak. In early PVE, the responsible microorganisms are nosocomial pathogens, such as staphylococci, gram-negative bacilli, and Candida species, which are introduced at the time of the operation or are hematogenously seeded in the immediate postoperative period. The pathophysiology of late PVE probably resembles that of native-valve endocarditis; that is, platelet-fibrin thrombi form on the valve leaflet and are then hematogenously seeded during episodes of transient bacteremia. In late PVE, the infecting organisms are usually streptococci, S. aureus, enterococci, and fastidious gram-negative organisms (the HACEK group).71–75 Infection appears to occur with equal frequency in both the mitral and aortic valves and is exceedingly rare in tricuspid prostheses (excluding intravenous drug abusers).67
In the ICU, cases of early PVE present more dramatically than do either native-valve endocarditis or late PVE. The clinical signs that suggest PVE include new or changing murmurs, congestive heart failure, new electrocardiographic conduction disturbances, and the presence of systemic emboli. In fact, 40% of patients have clinically apparent central nervous system emboli.76–79 The diagnosis is confirmed by positive results of blood cultures and findings on transesophageal echocardiography (TEE). If blood cultures are obtained before institution of antibiotic therapy, more than 90% of culture results will be positive. Transesophageal echocardiography is the diagnostic imaging modality of choice because it has a sensitivity of 82% to 96%, as compared with 17% to 36% with transthoracic echocardiography.80,81 Transesophageal echocardiography also allows the detection of abscesses, fistulas, and perivalvular leaks.82 The treatment of early PVE involves the administration of antibiotics directed at the cultured organism and prompt surgical intervention in cases of complicated PVE (Table 37-4).83 Mortality is related to older age, S. aureus infection, and patients with persistent bacteremia with the occurrence of septic shock.84,85 In complicated PVE, survival is improved with the institution of both medical and surgical therapy versus medical therapy alone.77,86,87
TABLE 37-4 Indications for Surgical Intervention in Patients with Prosthetic Valve Endocarditis
Valvular regurgitation with or without heart failure |
Uncontrollable infection* caused by: |
Periannular extension |
Difficult-to-treat microorganisms |
Abscess |
Large vegetation with high risk for embolization or recurrent emboli |
* Uncontrollable infection includes persistent fever and positive results of blood culture.

Systemic Inflammatory Response Syndrome and Sepsis
Systemic inflammatory response syndrome (SIRS) and sepsis are part of a spectrum of disorders that produce dysfunction in a variety of organ systems and arise from a combination of tissue injury and the host’s response to that injury. In 1992,88 1997,89 and 2001,90 the American College of Chest Physicians, the SCCM, the European Society of Intensive Care Medicine, the American Thoracic Society, and the Surgical Infection Society published consensus statements to clarify the definitions used to describe this spectrum. In 1991, the consensus statement introduced the now widely used term SIRS to describe a syndrome with multiple, clinically evident, organ-system effects (e.g., fever, oliguria, mental-status changes) that occurred as the result of the body’s inflammatory response activated by a variety of causes.88 Historically, it had been thought that infection, particularly gram-negative infection, was the sole source of this clinical phenomenon.91,92 It is now accepted that any major tissue injury (burns, sterile pancreatitis, major trauma) can precipitate such a dramatic inflammatory response with or without the presence of infection.91,92 However, in the most recent consensus statement, the authors acknowledge that, although SIRS exists, it is too broad and lacks the specificity to make it a useful, working “diagnosis” at this time.90
Sepsis is defined as the clinical syndrome that occurs as the result of an infection (or suspected infection) and an inflammatory response (Table 37-5).90,92 Severe sepsis is associated with organ dysfunction, hypoperfusion, or hypotension. Septic shock includes sepsis-induced hypotension and organ-perfusion abnormalities that persist despite fluid resuscitation.88 A detailed description of the current understanding of the pathophysiology of SIRS and sepsis is beyond the scope of this chapter; see Chapter 8 and reviews of this topic93–97 for more detailed discussions.
Documented or suspected infection plus some of the following:a |
a Defined as a pathologic process induced by a microorganism.
b Defined as core temperature > 38.3° C.
c Defined as core temperature < 36° C.
d Defined as > 90 beats/min or > 2 standard deviations (SDs) above the normal value for age.
e Defined as > 20 mL/kg over 24 hours.
f Defined as a plasma glucose concentration > 120 mg/dL or 7.7 mmol/L.
g Defined as a white blood cell (WBC) count > 12,000/μL.
h Defined as a WBC count < 4000/μL.