24 Local anesthetics
Conduction Block Anesthesia: Local anesthetic injected in the immediate vicinity of a major nerve plexus (brachial plexus, lumbar plexus, and neuraxial anesthesia).
Field Block Anesthesia: Local anesthetic injected, in a fanlike manner, into tissue surrounding an incision or puncture site.
Infiltration Anesthesia: Local anesthetic injected at an incision or puncture site.
Intravenous Anesthesia: Lidocaine is injected into the vein of an exsanguinated extremity with an inflated tourniquet.
Neuraxial Blockade: A generic term that encompasses both spinal and epidural anesthesia.
Peripheral Nerve Block: Local anesthetic deposited in the immediate vicinity of an individual nerve to produce anesthesia.
Topical Anesthesia: Local anesthetic applied to skin or mucous membranes (pharyngeal cavity or urethra). Systemic absorption from the mucous membranes is rapid. Excessive dosing can lead to toxicity.3–5
Local anesthetics are used to render a portion of the body insensate to painful stimuli through reversible nerve conduction blockade. Erythroxylon coca, the source of cocaine, was used in antiquity. In 1855, cocaine was isolated from the coca bean and rapidly found a place in modern medicine. In 1884, Carl Koller demonstrated its ability to anesthetize the eye, and William Halsted used cocaine for infiltration and nerve blocks. Cocaine had two major problems: toxicity and physical dependence. The first ester local anesthetic suitable for injection, procaine, was introduced in 1905. In 1948, Lofgren introduced the first clinically useful amide local anesthetic.1,2 The use of local anesthetics for anesthesia and/or postoperative analgesia continues evolve. Advances in pharmacology and technology have increased the use of local anesthetics. Perianesthesia nurses should understand the basic physiology of nerve conduction, pharmacology of local anesthetics, and identification and treatment of complications that may arise from their use.
Nerve physiology and conduction
Neurons convey information to and from the central nervous system (CNS). Sensory information is transmitted from the periphery by afferent neurons to the CNS. Motor impulses are transmitted by efferent neurons from the CNS to the periphery. Individual neurons contain a cell body, dendrites, and axons. Dendrites are multiple extensions of the cell body that transmit information toward the cell body. An axon is a single extension that transmits information away from the cell body. Axons are enveloped in a cell membrane known as the axolemma. Endoneurium is the connective tissue that envelops individual nerve fibers. Several nerve fibers form fascicles and are in turn covered with perineurium. Several fascicles covered with perineurium are bundled together and covered by connective tissue to form the epineurium that encompasses the entire nerve (Fig. 24-1).4–7
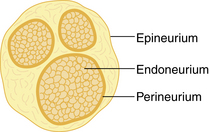
FIG. 24-1 Transverse section of a peripheral nerve.
(From Miller RD, et al: Miller’s anesthesia, ed 7, Philadelphia, 2009, Churchill Livingstone.)
Neurons contain positive and negative charges. At a resting state, the inside of the cell is more negative than the more positive extracellular charge. Within the neuron there are potassium (K+) ions, which have positive charges (cations), and proteins, which have negative charges (anions). The negatively charged proteins are impermeable to the walls of the cell, whereas the positively charged K+ ions are small enough to diffuse through small openings. Because negative ions attract positive ions, there is an accumulation of K+ within the neuron. The ratio of intracellular K+ to extracellular K+ is approximately 30:1. Extracellularly, sodium (Na+) is found in abundance. The ratio of extracellular Na+ to intracellular Na+ is approximately 10:1. Sodium ions are less permeable to the cell membrane and gain access through passive diffusion. Sodium specific channels are closed at this time, so the amount of Na+ that reaches the cell is low. In addition, the NA+/K+ pump actively maintains the gradient between extracellular Na+ and intracellular potassium. For every three Na+ ions that are removed from inside the cell, two K+ ions are replaced (Fig. 24-2). The net difference between the intracellular K+ (negative) and extracellular Na+ (positive) results in a resting membrane potential of –70 to –90 millivolts.4,7,8
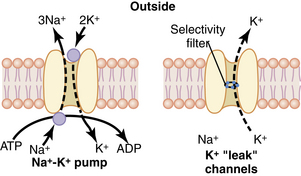
(From Hall JE: Guyton and Hall textbook of medical physiology, ed 12, Philadelphia, 2011, Saunders.)
Depolarization results in an intracellular change from negative to positive. This occurs because Na+ specific channels are opened (or activated), allowing Na+ to enter the cell. As Na+ enters the cell, additional Na+ channels are opened, resulting in a positive feedback loop. Potassium-specific channels are opened, allowing K+ to move out of the cell and into the extracellular space. When membrane potential becomes reversed, to approximately +35 millivolts, the movement of Na+ into the cell and K+ out of the cell stops (Na+ channels become inactivated) and the Na+/K+ pump restores the membrane potential back to a resting state (Na+ channels are at a resting state; Fig. 24-3).4,7,8
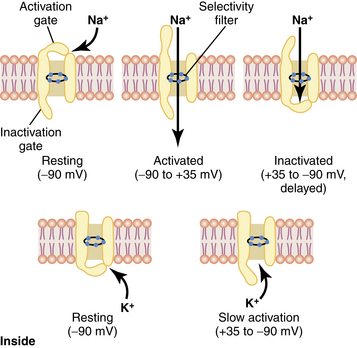
FIG. 24-3 Characteristics of the voltage-gated sodium (top) and potassium (bottom) channels.
(From Hall JE: Guyton and Hall textbook of medical physiology, ed 12, Philadelphia, 2011, Saunders.)
An action potential at a singular point will cause additional areas to also depolarize; this is called propagation. Action potentials must reach threshold to continue propagation (all or none response). In unmyelinated nerves, action potentials are propagated along Na+ and K+ gates located in close proximity, and the conduction rate is slow (0.1 to 2.0 m/sec; Fig. 24-4). Myelinated nerves are, in effect, insulated. Ions cannot move through the myelin. Nodes of Ranvier represent an interruption in the myelin. It is here that action potentials can stimulate depolarization and propagation of an impulse. Because the nodes of Ranvier are spaced farther apart, saltatory conduction occurs, resulting in a faster conduction rate (3-120 m/sec; Fig. 24-5).4,7,8
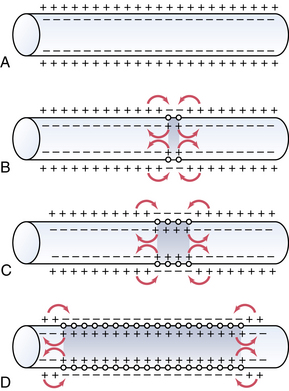
(From Hall JE: Guyton and Hall textbook of medical physiology, ed 12, Philadelphia, 2011, Saunders.)
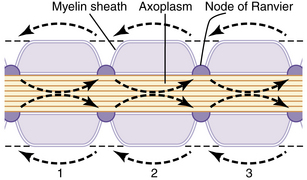
(From Hall JE: Guyton and Hall textbook of medical physiology, ed 12, Philadelphia, 2011, Saunders.)
Local anesthetics work at the level of the Na+ channel to prevent action potentials from being potentiated and not by altering resting or threshold potential. Local anesthetic molecules are stereospecific and bind within the Na+ channel or near its opening. Local anesthetic molecules have a greater affinity for receptors during their open or inactive state. When local anesthetic molecules bind with the Na+ channel, it prevents a conformational change and the influx of Na+, and depolarization slows. Eventually the minimum blocking concentration of the local anesthetic prevents an action potential from being achieved, and electrical conduction is blocked (Fig. 24-6).4,7,9
Nerve fiber type and local anesthetic effects
Peripheral nerves are divided into three classifications based on diameter, myelination, and conduction velocity. A and B peripheral nerves are myelinated, whereas C fibers are not. A fibers have the fastest conduction velocities, followed by B and C fibers. A fibers are further divided into alpha (α), beta (β), gamma (γ), and delta (δ) fibers. A-α fibers have the fastest conduction velocities and are motor neurons (efferent). A-β fibers are slower than A-α fibers but similar to A-γ fibers in conduction velocity, and are sensory (afferent) neurons that detect touch, pressure, and proprioception. A-γ fibers have a similar conduction speed to A-β fibers and are motor neurons (efferent) to muscle spindles and responsible for reflexes. A-δ fibers have the slowest conduction velocity of the A fibers, are sensory (efferent) for sharp pain (also known as fast pain such as an incision or pin prick) and temperature. B fibers have a slower conduction velocity than the A fibers and are preganglionic sympathetic neurons (efferent). C fibers are unmyelinated, have the slowest conduction velocity, and are postganglionic sympathetic neurons (efferent). C fibers transmit slow pain (generalized ache or burning sensation) and temperature (Table 24-1).3,4,7,9
FIBER TYPE | MYELIN | FUNCTION |
---|---|---|
A-α | +++ | Motor (efferent: to skeletal muscle) |
A-β | ++ | Touch, pressure, proprioception (afferent: from skin) |
A-γ | ++ | Motor (efferent: to muscle spindles) |
A-δ | ++ | Pain (sharp, fast) and temperature (efferent: from skin) |
B | + | Preganglionic sympathetic (efferent: to vascular smooth muscle) |
C | None | Pain (dull, slow) and temperature (afferent from skin); postganglionic sympathetic (efferent: to vascular smooth muscle) |
From Schick L, Windle PE, editors: Perianesthesia nursing core curriculum: preprocedure, phase I and phase II PACU nursing, ed 2, St. Louis, 2010, Saunders.
Classification of peripheral nerves is important in determining the sequence of local anesthetic blockade. B fibers are the most sensitive. Dilation of cutaneous blood vessels is often the first sign of local anesthetic onset. C fibers and A-δ are next in sensitivity, resulting in the inability to feel cool sensations such as an alcohol wipe. Next in sensitivity are the A-γ, A-β, and A-α fibers, which result in the loss of sensation, pressure, proprioception, and finally motor paralysis. Local anesthetic concentration must be adequate to block nerve fibers. It takes twice the concentration of local anesthetic to block motor fibers as it does to block sensory and sympathetic fibers require the least. The order of blockade onset is B fibers > C fibers = A-δ fibers > A-γ fibers > A-β fibers > A-α fibers. Recovery from local anesthetic is the opposite (Box 24-1).3,4,7,9
Local anesthetic pharmacology
Chemistry and stereoisomerism
A local anesthetic molecule contains a lipophilic group, an intermediate bond, and a hydrophilic group. The lipophilic (attracted to fat) portion of the molecule is aromatic, usually an unsaturated benzene ring. Classification is dependent on the intermediate bond, which is either an ester (–CO–) or an amide (–HNC–). The hydrophilic (attracted to water) group is generally a tertiary amine and proton acceptor (Fig. 24-7).4,10,11 The lipophilic and hydrophilic portions of the molecular structure are crucial to its ability to cross tissue barriers and reach their site of action. The intermediate bond determines the type of local anesthetic, affecting metabolism and potential for allergic reactions.4 Commonly administered amides and esters are noted in Box 24-2.
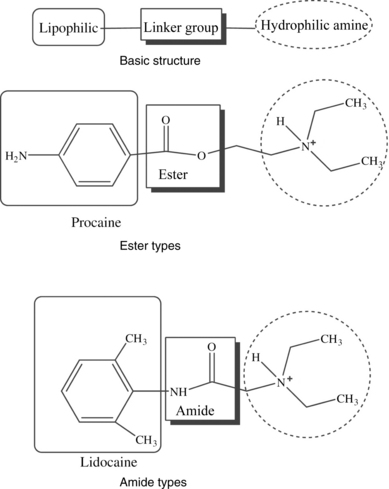
FIG. 24-7 Chemical structure of local anesthetics.
(From Friel CJ, et al: Local anesthetic use in perioperative areas, Perioperative Nurs Clin 5:203–214, 2010.)
BOX 24-2 Local Anesthetics
Amides | Esters |
---|---|
Bupivacaine | Benzocaine |
Etidocaine | Chloroprocaine |
Levobupivacaine | Cocaine |
Lidocaine | Procaine |
Mepivacaine | Tetracaine |
Prilocaine | |
Ropivacaine |
Most local anesthetics are stereoisomers. Optical isomers (enantiomers) are mirror images of each other with the same chemical formula, but structurally cannot be superimposed on one another. Most medications are racemic mixtures containing more than one isomer. Because drug receptors are stereospecific and stereoselective, administering a medication that contains stereoisomers means that the clinician is administering more than one medication. Each enantiomer may exhibit differences in absorption, distribution, potency, toxicity, and therapeutic action (Fig. 24-8). Advances in pharmacology have allowed the development of two pure S-enantiomers: ropivacaine and levobupivacaine. Both exhibit advances in safety because they are less toxic than racemic preperations,9,11,12 although they are still capable of causing toxicity.13
Pharmacodynamics
Pharmacodynamics is the study of a medication’s effect on the body.5 Local anesthetics are weak bases and exist as charged (ionized, water soluble) and uncharged (non-ionized, lipid soluble) particles. For local anesthetics to reach their site of action—the nerve fiber—they must transverse biologic barriers. Ionized local anesthetics do not pass through the cell membrane easily; however, non-ionized local anesthetics cross the barrier of the cell membrane, gaining access to the sodium channel. Non-ionized local anesthetics do not readily bind with Na+ channels; however, ionized local anesthetics will bind easily. The ratio of ionized to non-ionized local anesthetic is pH dependent. The pH at which a basic medication contains a 50% concentration of ionized and 50% non-ionized local anesthetic is known as pKa. Injectable local anesthetics vary in pKa from 7.6 to 9.0. The closer the local anesthetics pKa is to physiologic pH, the faster the onset (chloroprocaine is an exception). Confounding this is commercial preparation of local anesthetics. To stabilize local anesthetics, manufacturers prepare them as a hydrochloride salt with a pH of 6 to 7. If the local anesthetic contains epinephrine, it must placed in an even more acidic environment (pH of 4 to 5), resulting in less non-ionized local anesthetic to cross tissue and thus a slower onset. The same holds true when local anesthetic is injected into infected (acidotic tissue).4,7,9,11,13
Potency and duration of action of a local anesthetic is closely correlated to lipid solubility. In general, the more lipid soluble the local anesthetic, the more potent it is and the longer its duration of action. Potent local anesthetics bind with proteins and neural tissue readily, lending to their longer duration of action and toxicity potential. Bupivacaine, levobupivacaine, ropivacaine, etidocaine, and tetracaine are the most potent. Lidocaine, mepivacaine, and prilocaine are moderate, whereas procaine and chloroprocaine are the least potent.4,7,9,14
Pharmacokinetics
Pharmacokinetics is the study of a medication’s absorption, distribution, metabolism, and excretion.5 Absorption of local anesthetics is dependent on site of injection, dose, vasoconstrictor, pharmacologic profile, and individual physiologic factors.3,4,11 Vascularity of tissue has a direct effect on systemic absorption (increases uptake and may lead to toxicity). Absorption of local anesthetic from highest to lowest is as follows: intravenous, tracheal, intercostal, caudal, epidural, brachial plexus, sciatic, and subcutaneous.3,11,14 Total dose is closely related. The greater the dose, the greater the systemic absorption.7 Attention to the total dose is important to avoid toxicity. Epinephrine can be added to decrease systemic absorption and prolong duration of action through local vasoconstriction. Norepinephrine and phenylephrine can be added but are less efficacious. The ability of epinephrine to decrease systemic absorption varies by local anesthetic and injection site. The addition of epinephrine for peripheral nerve blocks and epidurals increases duration of action and decreases blood levels by 10% to 30% for lidocaine, mepivacaine, and bupivacaine, but does not alter duration or blood levels for ropivacaine and levobupivacaine. Attention should be placed on the total dose of epinephrine to avoid deleterious effects.4,7,9 All local anesthetics, with the exception of cocaine and ropivacaine, cause a degree of vasodilatation. Vasodilatation results in increased blood flow to the area of injection and absorption. Lidocaine results in greater local vasodilation than mepivacaine and prilocaine, whereas bupivacaine, levobupivacaine, and etidocaine produce the same degree of vasodilatation.4 Local anesthetics also vary in protein binding. Local anesthetics that are highly protein bound are absorbed less readily. Protein binding varies from 6% for procaine, which is the least, to greater than 97% for levobupivacaine. Least to greatest protein bound is as follows: procaine < prilocaine < lidocaine < tetracaine < mepivacaine < ropivacaine = etidocaine < bupivacaine < levobupivacaine.3,4,9 A final factor in determining the absorption rate of local anesthetics are individual physiological conditions. Extremes of age are a factor because newborns have immature hepatic function, and the elderly have decreased hepatic enzyme function and blood flow resulting in increased in blood concentrations. During pregnancy, doses of local anesthetic should be decreased by one third because of hyperdynamic circulation and hormonal changes that can result in toxicity. Renal, hepatic, and cardiovascular disease can affect local anesthetic pharmacokinetics.4,7,14,15
Uptake of local anesthetics results in their distribution to various tissues. The initial rapid disappearance phase (α) includes uptake into highly perfused tissue (brain, lung, liver, kidney, and heart). The lungs act as a large reservoir and decrease initial blood concentrations. The slow phase of disappearance (β) includes distribution to the gut and muscle. Muscle provides a large reservoir for local anesthetics.4,7,14 During pregnancy, amide local anesthetic molecules can be transferred across the placenta and accumulate in an acidotic environment; this is known as ion trapping.3,9
Metabolism depends on classification: amide or ester. Amides are metabolized primarily in the liver through cytochrome P-450 system. Extremes of age and disease processes that affect liver perfusion and enzyme activity alter hepatic elimination of amides. Individual amides exhibit different clearance rates (prilocaine > etidocaine > lidocaine > mepivacaine > bupivacaine> ropivacaine). Esters undergo rapid hydrolysis in the blood, which limits toxicity. Plasma cholinesterase is the primary enzyme responsible.4,9 Caution should be used in patients with a acquired or genetic pseudocholinesterase deficiency, which can decrease metabolism and lead to potentially toxic blood concentrations.4,16 Metabolism of some ester local anesthetics results in the production of p-aminobenzoic acid (PABA), a known allergen. It is this by-product of metabolism that makes ester local anesthetics more likely than amides to result in an allergic reaction.4,14
Maximum doses
Maximum doses are general guidelines derived from animal studies in an attempt to identify median effective and toxic doses. Health care providers should take into account the site of injection, the addition or absence of epinephrine, and individual medical conditions such as age, renal, hepatic, cardiac disease, and pregnancy.15 Refer to Table 24-2 for maximum doses of local anesthetics.
Individual local anesthetics
Table 24-2 contains a summary of local anesthetic information.
Additional local anesthetics
Etidocaine (Duranest) is a long-duration, slow-onset amide local anesthetic introduced in 1972. It can be used for infiltration, peripheral nerve block, and epidural anesthesia. Maximum dose is 300 mg plain and 400 mg with epinephrine. Etidocaine does not enjoy widespread use because it produces a profound and long motor block.2,3 Prilocaine (Citanest) is a moderate-duration, slow-onset amide local anesthetic introduced in 1960. It can be used for infiltration, intravenous regional block, peripheral nerve block, and epidural anesthesia. The limiting factor is its metabolism to O-toluidine, which can cause methemoglobinemia (see Complications). Prilocaine is not used in the United States, with the exception of eutectic mixture of local anesthetics (EMLA) cream.3,7,11 Benzocaine is an ester local anesthetic that exists as a weak acid. Because it is non-ionized, it is used only as a topical local anesthetic. Onset is rapid, duration is short, and the maximum dose is 200 to 300 mg. Benzocaine is the most commonly implicated local anesthetic in methemoglobinemia. When using benzocaine, it is important that the manufacturer’s recommendations are strictly followed and patients are monitored for this complication.9,14 EMLA cream is a mixture of 2.5% prilocaine and 2.5% lidocaine that can be applied topically to decrease the discomfort associated with venipuncture, and it is particularly useful when starting intravenous lines on children. Dosing is based on patient weight and surface area, and manufacturer dosing recommendations require strict adherence. Application should occur on intact skin because absorption may be rapid with breaks in the integument. Onset is slow, and depth of penetration is limited. EMLA should not be used in infants younger than 3 months, infants 3 to 12 months old receiving additional medications that may predispose them to methemoglobinemia (i.e., nitrates, dapsone, sulphonamides, benzocaine), and children who may be predisposed to hereditary or congenital methemoglobinemia.11,25–27
Complications
Vigilant monitoring for and recognition of allergic reactions, methemoglobinemia, and local anesthetic toxicity are essential to the institution of life saving interventions (Table 24-3).
Allergic reactions
True allergic reactions to local anesthetics are rare.28–30 Allergy to ester local anesthetics are more common than amides because they are metabolized to PABA, a known allergen.4,14 Allergic reactions may be related to preservatives added to local anesthetics. Methylparaben, propylparaben, and sulfites are added to a number of consumer goods, including local anesthetics, to enhance shelf life. Prior exposure to methylparaben or propylparaben is likely, and there is a known cross-reactivity between PABA and these preservatives. In addition, sulfites are known to cause allergic reactions.10,31 Sulfonamides are similar to PABA. Patients reporting allergic reactions to sulfonamides should not be exposed to ester- or paraben-containing local anesthetics.31 Allergic reactions are more likely to occur related to exposure to substances such as skin preps, latex, or other medications administered in close proximity.32 Additional patient-reported allergic reactions may be related to prior experiences resulting in anxiety or rapid systemic absorption (e.g., dental procedures). Anxiety can lead to vasovagal reactions during injection, and rapid systemic absorption of epinephrine containing local anesthetics can lead to palpitations, shortness of breath, syncope, and toxicity.31
Allergic reactions can be divided into four types based on the causative agent and immune systems response (see Chapter 18). Of immediate concern are anaphylactic reactions. Anaphylaxis is a type I reaction that results in the release of a number of substances, including histamine and leukotrienes, through mast cell and basophil activation. Clinical signs and symptoms include hypotension, tachycardia, dysrhythmias, cardiovascular collapse, shock, bronchospasm, cyanosis, dyspnea, hypoxia, angioedema, urticaria, and pruritus. Immediate treatment is required to reduce mortality and morbidity.33–35
Hemodynamics should be supported by vasopressors. Epinephrine is the first medication of choice because it helps to restore vascular tone, support blood pressure, and reverse bronchoconstriction. Initial bolus doses of 50 to 100 mcg in adult patients are administered and subsequently tailored to patient response. Antihistamines (e.g., diphenhydramine, 1 to 2 mg/kg intravenously; usual dose is 25 to 50 mg, with a maximum dose of 100 mg), bronchodilators, and glucocorticoids are considered and administered as needed. When the reaction has been controlled, the patient is closely monitored for a rebound of symptoms.34,35
Methemoglobinemia
Methemoglobinemia can be caused by benzocaine (most common), prilocaine, lidocaine, and tetracaine.36,37 Some institutions have discontinued the use of benzocaine, and others have suggested that it not be used.36,38 Methemoglobin is a form of hemoglobin that is unable to carry oxygen. When an agent such as benzocaine is administered, ferrous iron (Fe+3) is oxidized to ferric iron (Fe+2) either directly or during metabolism. Signs and symptoms include: cyanosis, chocolate-colored blood, headache, weakness, dizziness, tachycardia, dyspnea, seizures, coma, dysrhythmias, and heart failure. Pulse oximetry readings range from 80% to 85% regardless of the severity. The gold standard in diagnosis is cooximetry, available with arterial blood gas analysis. Supportive treatment includes supplemental oxygen and hemodynamic support. Definitive treatment is methylene blue. The dose for adults is 1 to 2 mg/kg over 5 minutes; this can be repeated once every hour up to a maximum of 7 mg/kg. For newborns, the dose should be reduced to 0.5 mg/kg IV. Methylene blue should not be used in patients with a history of glucose-6-phosphate dehydrogenase (G6PD) deficiency; ascorbic acid should be used instead. Patients are monitored for 24 hours for possible recurrence.36,38–40 When benzocaine is administered, manufacturers’ guidelines should be followed and minimal amounts should be delivered. Contraindications include patients with cardiopulmonary disease (e.g., smokers, asthma, bronchitis, emphysema, heart disease), extremes of age (elderly and infants), and patients with known G6PD deficiency, hemoglobin M disease, NADH-MHb reductase deficiency, or pyruvate-kinase deficiency.38
Local anesthetic toxicity
Local anesthetic (LA) toxicity occurrence has been estimated at 4 per 10,000 epidurals and 7.5 to 20 per 10,0000 peripheral nerve blocks.41 Toxicity is due to high plasma concentrations affecting neurologic and cardiovascular systems. Toxicity may be due to inadvertent intravascular injection.9 A test dose before injecting local anesthetics help to confirm that the needle–catheter is outside a vessel; it contains 3 mL of 2% lidocaine with 1:200,000 (15 mcg) concentration of epinephrine. If positive, the heart rate and systolic blood pressure will increase by at least 20 beats/min and 15 mm Hg. Test doses might not be reliable in the presence of beta blockers, advanced age, and general anesthesia.41 Excessive dosing is a common cause and combining local anesthetics are cumulative and do not lessen the risk.14 Systemic absorption can result in toxicity and is related to total dose, vascularity of injection site, vasoconstrictor use, and individual local anesthetic used.9
Classic signs and symptoms are described as progressive as blood concentrations of local anesthetics increase. CNS symptoms progress from circumoral or tongue numbness and tinnitus up to seizures and coma (Fig. 24-9).4 The most common CNS symptoms are seizures; followed by dizziness, drowsiness, tinnitus, circumoral numbness, confusion, dysphoria, agitation, and loss of counciousness.42 Threshold for CNS toxicity is lower for potent local anesthetics (bupivacaine, tetracaine, and etidocaine) and in patients experiencing hypercarbia or metabolic acidosis.4 Higher blood concentrations lead to cardiovascular symptoms.4,14 Bradycardia and asystole are the most common manifestations, followed by hypotension, tachycardia, ventricular fibrillation or ventricular tachycardia, wide complex arrhythmias, ST changes, pain dyspnea, hypertension, and ventricular ectopy.4,14,42 Blood concentrations resulting in cardiovascular toxicity are generally three times the amount that result in seizures. Hypoxia, hypercarbia, acidosis, cardiovascular disease, and pregnancy are predisposing factors that increase the risk for cardiovascular toxicity.3,4,14,42
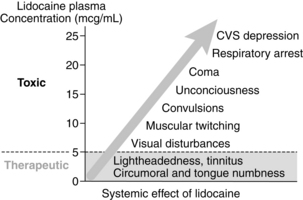
FIG. 24-9 Toxicity progression of lidocaine.
(From Nagelhout J, Plaus K, editors: Nurse anesthesia, ed 4, St. Louis, 2010, Saunders.)
The most common regional anesthetic techniques that result in LA toxicity are epidural, axillary, and interscalene blocks. Toxicity occurs more frequently in females, and age plays a role with 45% of the patients younger than 16 or older than 60 years. Most signs and symptoms of LA toxicity occur within 5 minutes, but up to 25% may occur later. Patients receiving local anesthetic infusions through epidural or peripheral nerve catheters may exhibit signs and symptoms of toxicity hours to days later. Signs and symptoms of LA toxicity can occur in any order, including CNS, CNS and cardiovascular symptoms occurring at the same time, or cardiovascular toxicity occurring without CNS symptoms. This highlights the importance of vigilance regarding changes in the patient’s condition that indicate toxicity is occurring.42
Initial treatment of LA toxicity should focus on airway, breathing, and circulation. The patient should have a patent airway and 100% oxygen administered. Seizure activity should be controlled with a benzodiazepine. Propofol should not be used if cardiovascular depression is occurring or impending. CPR and ACLS should be instituted. In patients with LA toxicity, epinephrine may increase dysrhythmias; lidocaine will add to the toxicity; and phenytoin, beta blockers, and calcium channel blockers may contribute to cardiovascular depression.43–46
Cardiovascular collapse is the most serious and difficult complication to treat. Bupivacaine, etidocaine, and tetracaine are the most cardiotoxic. Treatment for local anesthetic toxicity has recently been standardized.46,47 Lipid emulsion therapy is a crucial component for successful resuscitation and is theorized to create an additional compartment for local anesthetic molecules to bind, reducing the amount of molecules that bind within the cardiovascular system.
Complications of lipid emulsion therapy in this setting have not been reported.43–46 Current recommendations include an initial bolus of 20% lipid emulsion in a dose of 1.5 mL/kg rapidly over 1 minute, followed by a continuous infusion at 0.25 mL/kg/min. If cardiovascular collapse continues, up to two additional boluses can be administered. If the patient remains hypotensive, the infusion can be increased to 0.5 mL/kg/min. Lipid infusion should continue for a minimum of 10 minutes after the patient has been stabilized. The maximum dose administered in the initial 30 minutes is 10 mL/kg. Preparation should be made for cardiopulmonary bypass. After stabilization, the patient should be monitored in an intensive care setting for potential recurrence of signs and symptoms of toxicity.43–46
The perianesthesia nurse is crucial in assisting the anesthesia provider in monitoring the patient during the placement of a regional anesthetic. If epinephrine-containing solutions or a test dose with epinephrine are administered, close monitoring of changes in heart rate and blood pressure should occur. Maintaining verbal contact with the patient is essential to assess the patient for changes in level of consciousness or initial CNS signs and symptoms that accompany toxicity. Continuous monitoring of the patient’s electrocardiogram and blood pressure should occur to detect changes in rhythm or hypotension. A high level of suspicion for LA toxicity should be maintained during block placement and for up to 30 minutes thereafter.4,42,46
Summary
Perianesthesia nurses have an important role in the treatment of acute pain. Several modes of analgesia are combined to bring comfort to the patient recovering from surgery.48 Local anesthetics continue to be an important pharmacologic mainstay, and their use appears to be increasing as a result of advances in their administration. It is imperative that perianesthesia nurses understand the physiology of nerve conduction, pharmacology of local anesthetics, and identification and treatment of potential complications related to their administration.
1. Morgan GE, et al. The practice of anesthesiology. Morgan GE, et al. Clinical anesthesiology, ed 4, New York: McGraw-Hill, 2006.
2. Ruetsch YA, et al. From cocaine to ropivacaine: the history of local anesthetic drugs. Curr Top Med Chem.2001;1:175–182.
3. Brown C. Anesthetic agents and adjuncts. Schick L, Windle PE. Perianesthesia nursing core curriculum: preprocedure, phase I and phase II PACU nursing. ed 2. St. Louis: Saunders; 2010.
4. Nagelhout J. Local anesthetics. Nagelhout J, Plaus K. Nurse anesthesia, ed 4, St. Louis: Saunders, 2010.
5. Venes D, ed. Tabor’s cyclopedic medical dictionary, ed 21, Philadelphia: F.A. Davis Company, 2009.
6. Hadzic A, Vloka JD. Essential regional anesthesia anatomy. Hazic A, Vloka JD. Peripheral nerve blocks: principles and practice. New York: McGraw-Hill; 2004.
7. Berde CB, Strichartz GR. Local anesthetics. Miller RD, ed. Miller’s anesthesia, ed 7, Philadelphia: Churchill Livingstone, 2010.
8. Hall JE. Membrane potentials and action potentials. Hall JE, ed. Guyton and Hall textbook of medical physiology, ed 12, Philadelphia: Saunders, 2011.
9. Stoelting RK, Hillier SC. Local anesthetics. Stoetling RK, Hillier SC. Pharmacology & physiology in anesthetic practice, ed 4, Philadelphia: Lippincott Williams & Wilkins, 2006.
10. Friel CJ, et al. Local anesthetics use in perioperative areas. Perioperative Nursing Clinics. 2010;5:203–214.
11. Drasner K. Local anesthetics. Stoelting RK, Miller RD. Basics of anesthesia, ed 5, Philadelphia: Churchill Livingstone, 2007.
12. Joyce JA. A pathway toward safer anesthesia: stereochemical advances. AANA J.2002;70:63–67.
13. Zink W, Graf BM. The toxicity of local anesthetics: the place of ropivacaine and levobupivacaine. Curr Opin Anaesthesiol.2008;21:645–650.
14. Morgan GE, et al. Local anesthetics. Morgan GE, et al. Clinical anesthesiology, ed 4, New York: McGraw-Hill, 2006.
15. Rosenberg PH, et al. Maximum recommended doses of local anesthetics: a multifactoral concept. Reg Anesth Pain Med.2004;29:564–575.
16. Soliday FK, et al. Pseudocholinesterase deficiency: a comprehensive review of genetic, acquired, and drug influences. AANA J.2010;78:313–320.
17. Novacain (procaine hydrochloride injection solution) (package insert). Lake Forest, Ill: Hospira, Inc; 2005.
18. Nesacaine (chloroprocaine hydrochloride injection) (product monograph). Mississauga, Ontario: AstraZeneca; 2006.
19. Lidocaine hydrochloride injection (package insert). Shirley, NY: American Regent, Inc; 2003.
20. Carbocaine (mepivacaine hydrochloride injection) (package insert). Lake Forest, Ill: Hospira, Inc; 2009.
21. Allegri M, et al. Efficacy of drugs in regional anesthesia: a review. Eur J Pain.2009;3:41–48.
22. Naropin (ropivacaine hydrochloride monohydrate injection solution) (package insert). Schaumburg, Ill: APP Pharmaceutical, LLC; 2009.
23. Marcaine (bupivacaine hydrochloride injection,solution) (package insert). Lake Forest, Ill: Hospira, Inc; 2004.
24. Chirocaine (levobupivacaine injection) (package insert). Stamford, Conn: Purdue Pharma L.P; 2000.
25. Couper RTL. Methaemoglobinaemia secondary to topical lignocaine/prilocaine in a circumcised neonate. J Paediatr Child Health. 2000;4:206–207.
26. Pesatura KA, Matthews M. Topical anesthesia use in children. US Pharm. 2009;34:HS-4–HS-7.
27. EMLA Patch (transdermal therapeutic system) (package insert). Wilmington, Del: AstraZeneca; 2004.
28. Balestrieri PJ, Ferguson JE. Management of a parturient with a history of local anesthetic allergy. Anesth Analg. 2003;96:1489–1490.
29. Morais-Almeida M, et al. Allergy to local anesthetics of the amide group with tolerance to procaine. Allergy. 2003;58:827–828.
30. Caron AB. Allergy to multiple local anesthetics. Allergy Asthma Proc. 2007;28:600–601.
31. Finucane BT. Allergies to local anesthetics-the real truth. Can J Anaesth. 2003;50:869–874.
32. Harboe T, et al. Suspected allergy to local anesthetics: follow up in 135 cases. Acta Anaesthesiol Scand.2010;54:536–542.
33. Nitti JT, Nitti GL. Anesthetic complications. Morgan GE, et al. Clinical anesthesiology, ed 4, New York: McGraw-Hill, 2006.
34. O’Donnel MP. The immune system and anesthesia. Nagelhout J, Plaus K. Nurse anesthesia, ed 4, St. Louis: Saunders, 2010.
35. Stevenson J. Trauma care. Schick L, Windle PE. Perianesthesia nursing core curriculum: preprocedure, phase I and phase II PACU nursing. ed 2. St. Louis: Saunders; 2010.
36. Guay J. Methemoglobinemia related to local anesthetics: a summary of 242 episodes. Anesth Analg.2009;108:837–845.
37. Golembiewski J. Local anesthetics. J Perianesth Nurs. 2007;22:285–288.
38. Food and Drug Administration: FDA Public Health Advisory Benzocaine sprays marketed under different names including Hurricaine, Topex, and Cetacaine. available at: http://www.fda.gov/cder/drug/advisory/benzocaine.htm, September 23, 2010. Accessed
39. El-Husseini A, Basin P. Is threshold for treatment of methemoglobinemia the same for all? A case report and literature review. Am J Emerg Med. 2010;28:748.
40. Moos DD, Cuddeford JD. Methemoglobinemia and benzocaine. Gastroenterol Nurs. 2007;30:342–345.
41. Mulroy MF. Systemic toxicity and cardiotoxicity from local anesthetics: incidence and preventative measures. Reg Anesth Pain Med.2002;27:556–561.
42. DiGregorio G, et al. Clinical presentation of local anesthetic systemic toxicity: a review of published cases 1979-2009. Reg Anesth Pain Med.2010;35:179–185.
43. Clark MK. Lipid emulsion as rescue for local anesthetic-related cardiotoxicity. J Perianesth Nurs. 2008;23:111–117.
44. Manavi MV. Lipid infusion as a treatment for local anesthetic toxicity. AANA J.2010;78:69–78.
45. Winberg G. Lipid Rescue-Practice Advisory. available at http://lipidrescue.org, July 13, 2010. Accessed
46. Neal JM, et al. ASRA practice advisory on local anesthetic systemic toxicity. Reg Anesth Pain Med. 2010;35:152–161.
47. Corcoran W, et al. Local anesthetic-induced cardiac toxicity: a survey of contemporary practice strategies among academic anesthesiology departments. Anesth Analg.2006;103:1322–1326.
48. Krenzischek DA, et al. Pharmacology for acute pain: implications for practice. J Perianesth Nurs.2008;23:S28–S42.