4 Knee Injuries
Anterior Cruciate Ligament Injuries
Background
The anterior cruciate ligament (ACL) is the most frequently completely disrupted ligament in the knee; most of these injuries occur in athletes (Fig. 4-1). More than 100,000 ACL reconstructions are done each year in the United States.
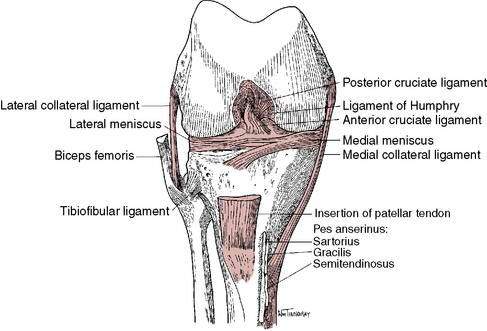
Figure 4-1 Anterior cruciate ligament and anatomic knee structures.
(Redrawn with permission from Miller MD, Howard RF, Planchar KD. Surgical Atlas of Sports Medicine. Philadelphia, 2003, Saunders, p. 74, Fig. 10-3.)
Hewett et al. (2005) in a level II study found that prescreened female athletes with subsequent ACL injury demonstrated increased dynamic knee valgus (Fig. 4-2) and high knee abduction loads on landing from a jump. Knee abduction moments, which directly contribute to lower extremity dynamic valgus and joint knee load, had a sensitivity of 78% and specificity of 73% for predicting future ACL injury. Neuromuscular training has been shown to decrease knee adduction moments at the knee (Hewitt et al. 1996), and this will be addressed at great length in the ensuing chapter.
Although a number of studies have suggested that OA eventually develops in 60% to 90% of individuals with ACL injuries (Beynnon 2005 Part 1, Andersson et al. 2009), a recent systematic review of the literature (Ojestad et al. 2009) concerning OA of the tibiofemoral joint more than 10 years after ACL injury suggests that these estimates are too high. The lack of a universal methodologic radiographic classification made it difficult to draw firm conclusions, but these investigators determined that in the highest-rated studies the reported prevalence of knee OA after isolated ACL injury was between 0% and 13%, and with meniscal injury, it was between 21% and 48% (level II evidence).
Treatment of ACL Injuries
Nonoperative Treatment (ACL-Deficient Knee)
Several authors have suggested criteria for nonoperative treatment in ACL tears: Fitzgerald et al. (2000) developed guidelines for selecting appropriate candidates for nonoperative ACL deficiency management (e.g., initiation of perturbation and strengthening program). The primary criteria were no concomitant ligament (e.g., medial collateral ligament) or meniscal damage and a unilateral ACL injury. Other criteria include the following:
Of four randomized controlled studies comparing nonoperative to operative treatment (level I evidence), one reported no difference in outcomes (Sandberg et al. 1987) and three reported superior results with operative treatment (Andersson et al. 1989 and 1991, Odensten et al. 1984).
Operative ACL Reconstruction
Timing of surgery. Because many patients had difficulty regaining full knee motion after acute or early reconstruction, delayed reconstruction has been suggested to minimize the possibility of postoperative arthrofibrosis. Good results have been reported after both acute and delayed reconstruction, mostly in retrospective case series. A prospective study compared outcomes in patients who had ACL reconstruction at four time points after injury (Hunter et al. 1996): within 48 hours, between 3 and 7 days, between 1 and 3 weeks, and more than 3 weeks. They found that restoration of knee motion and ACL integrity after ACL reconstruction was independent of the timing of surgery. Shelbourne and Patel (1995) suggested that the timing of ACL surgery should not be based on absolute time limits from injury. They reported that patients who had obtained an excellent range of motion (ROM), little swelling, good leg control, and an excellent mental state before surgery generally had good outcomes, regardless of the timing of surgery. Mayr et al. (2004) confirmed these observations in a retrospective review of 223 patients with ACL reconstructions: 70% of patients with a swollen, inflamed knee at the time of undergoing ACL reconstruction developed postoperative arthrofibrosis. It appears that the timing of reconstruction is not as important as the condition of the knee before surgery: full ROM, minimal effusion, and minimal pain are required (Beynnon et al. 2005, Part 1).
Graft choice. Bone-patellar tendon-bone (BPTB) autografts (Fig. 4-3) have been historically considered the “gold standard” for ACL reconstructions, although good outcomes have been reported with other graft choices, particularly hamstring grafts (Fig. 4-4 A–H). A number of studies have compared BPTB grafts with four-strand hamstring grafts, with most reporting no significant difference in functional outcomes, although difficulty with kneeling was more commonly reported by those with BPTB grafts.
Single-or double-bundle reconstruction. The rationale for two-bundle reconstruction is based on the identification of two distinct ACL bundles: the anteromedial (AM) and the posterolateral (PL) bundle (Fig. 4-5). The femoral insertion sites of both bundles are oriented vertically with the knee in extension, but they become horizontal when the knee is flexed 90 degrees, placing the PL insertion site anterior to the AM insertion site. When the knee is extended, the bundles are parallel; when the knee is flexed, they cross. In flexion, the AM bundle tightens as the PL bundle becomes lax, while in extension the PL bundle tightens and the AM bundle relaxes.
Cited advantages of single-bundle techniques include proven success, less technical difficulty, less tunnel widening, fewer complications, easier revision, lower graft cost when allograft is used, lower implant cost, and shorter surgical time (Prodromos et al. 2008).
Method of fixation. A variety of fixation devices are used for ACL reconstruction, with no consensus as to what is best. Generally, fixation can be classified as interference screw-based, cortical, or cross-pin (Prodromos et al. 2008). Interference screw and cortical fixation can be used in both the femur and the tibia. Interference screw fixation functions by generating frictional holding power between the graft and the bone tunnel wall (Prodromos et al. 2008). Cortical fixation can be direct, compressing the graft against the cortex, or indirect, connecting the graft to the cortex with some sort of interface, often a fabric or metal loop through which the graft is passed. Cross-pinning is a relatively new fixation technique for which advocates cite the advantage of being closer to the tunnel opening than cortical fixation. This advantage, however, has not been proved. A meta-analysis showed that cortical fixation provided more stability than aperture fixation (Prodromos et al. 2005), and a prospective comparison of three fixation devices, including cross-pin fixation, found no statistically or clinically relevant differences in results at 2-year follow-up (Harilainen and Sandelin 2009). All currently used fixation techniques appear to provide adequate stability to allow early aggressive rehabilitation after ACL reconstruction (Hapa and Barber 2009).
ACL Rehabilitation Rationale
Protocols for rehabilitation after ACL reconstruction follow several basic guiding principles:
REHABILITATION PROTOCOL 4-1 Criteria-Based Postoperative ACL Reconstruction Rehabilitation Protocol
Exercises
Phase III (Weeks 2–4)
Exercises
Phase IV (Weeks 4–8)
Precautions
Exercises
Phase V (Weeks 8–12)
Exercises
Phase VII (Weeks 16–24)
For Revision ACL Reconstructions
Per specific physician recommendation, follow typically similar protocol until 12 weeks, then extend weeks 12 to 16 through to 5- to 6-month timeline, when patients can then begin running and progress to functional sports activities. See Figure 4-90 for an illustration of abduction contrakicks/steamboats (flexion, extension, and adduction contrakicks can be performed by rotating patient 90 degrees at a time).
Single-leg triple hop for distance: 80% for running, 90% for return to sport
Triple crossover hop for distance: 80% for running, 90% for return to sport
Timed 10-m single-leg hop: 80% for running, 90% for return to sport