CHAPTER 82 Knee
The knee is the largest synovial joint in the body. It consists of three distinct and partially separated compartments that collectively form a complex ‘hinge’ joint. This arrangement offers a fulcrum for the powerful extensor and flexor muscles that act on the joint during propulsion. A number of stabilizing factors counter the considerable biomechanical demands that are imposed upon the joint: most important is a complex arrangement of intracapsular and extracapsular ligaments. Our understanding of the anatomy of the knee has improved considerably in recent years, due in large measure to significant technological advances in diagnostic imaging and surgery.
SKIN AND SOFT TISSUE
SKIN
Cutaneous vascular supply and lymphatic drainage
The arterial supply of the skin covering the knee is derived from genicular branches of the popliteal artery, the descending genicular branch of the femoral artery, and the anterior recurrent branch of the anterior tibial artery, with small contributions from the arteries to vastus medialis and the hamstrings (Fig. 82.1). For further details consult Cormack & Lamberty (1994).
Cutaneous innervation
Infrapatellar branch of the saphenous nerve
The infrapatellar branch of the saphenous nerve reaches the anterior aspect of the knee from the medial side. It is invariably divided in the medial approach to the knee, which accounts for the numbness that inevitably occurs lateral to the scar of a medial knee incision. A painful neuroma may form if the nerve is partially sectioned, e.g. by the incision for an arthroscopy portal or a small medial arthrotomy. Unfortunately the position of the nerve relative to the line of the joint is variable. In most cases it crosses just below the joint line, passing over the patellar tendon at its insertion into the tibia. For further details see Tennant et al (1998).
SOFT TISSUE
Popliteal fossa
The popliteal fossa (Figs 82.2, 82.3) is a narrow intermuscular space posterior to the knee joint, with a diamond-shaped outline that is rendered more apparent when its boundaries are artificially separated during dissection. The boundaries are biceps femoris proximolaterally; semimembranosus and the overlying semitendinosus proximomedially; the lateral head of gastrocnemius with the underlying plantaris distolaterally, and the medial head of gastrocnemius distomedially. The anterior boundary (or floor) of the fossa is formed, in proximodistal sequence, by the popliteal surface of the femur, the oblique popliteal ligament (overlying the posterior surface of the capsule of the knee joint), and the posterior aspect of the proximal tibia covered by popliteus and the fascia overlying popliteus. The fossa is covered posteriorly by the popliteal fascia, which is referred to as the roof of the fossa. The popliteal fascia is continuous with the fascia lata proximally and with the fascia cruris distally. It is a dense layer that is strongly reinforced by transverse fibres and is often perforated by the short saphenous vein and sural nerve; these two structures are useful landmarks in the direct posterior approach to the knee joint. (Note that ‘popliteal fascia’ refers to the deep fascia that forms the ‘roof’ of the fossa, in contradistinction to the ‘fascia overlying popliteus’, which forms part of the floor.)
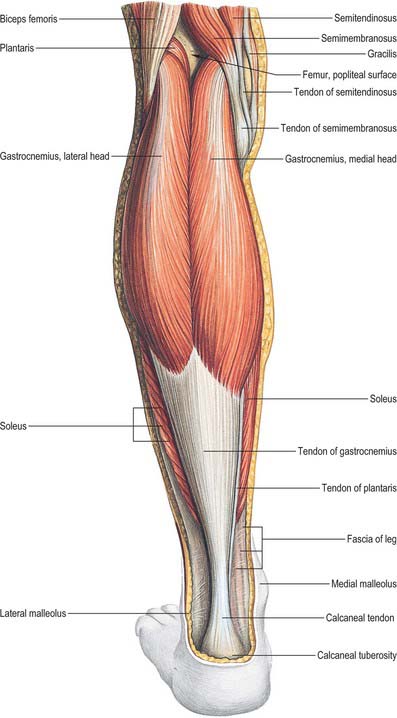
Fig. 82.3 Muscles of the calf: superficial view including boundaries of popliteal fossa.
(From Sobotta 2006.)
Contents
With its boundaries undisturbed, the popliteal fossa is typically 2.5 cm wide. Its contents are largely hidden, especially in its distal part, where the heads of gastrocnemius are in contact with each other. When its boundaries are separated, its contents are revealed as the popliteal vessels (Figs 82.2, 82.4), tibial and common fibular nerves, short saphenous vein, sural nerve, posterior femoral cutaneous nerve, an articular branch from the obturator nerve, lymph nodes, fat and a variable number of collapsed bursal sacs. The tibial nerve descends centrally immediately anterior to the popliteal fascia, crossing the vessels posteriorly from lateral to medial. The common fibular nerve descends laterally immediately medial to the tendon of biceps femoris. Popliteal vessels are deeply located and held together by dense areolar tissue. They lie on the floor of the fossa, the popliteal vein being superficial to the artery. Proximally, the thick-walled vein lies lateral to the artery, crossing to its medial side distally. At times the popliteal vein is duplicated, and the artery lies between the two veins, which are usually bridged by connecting channels. An articular branch from the obturator nerve descends on the artery to the knee. Six or seven popliteal lymph nodes are embedded in the fat, one under the popliteal fascia near the termination of the short saphenous vein, one between the popliteal artery and knee joint, the others around the popliteal vessels.
BONE
FEMUR, TIBIA AND FIBULA
The femur is described in Chapter 80 and the tibia and fibula are described in Chapter 83.
PATELLA
Topography
The patella is the largest sesamoid bone (Figs 82.5, 82.6) and is embedded in the tendon of quadriceps femoris, anterior to the distal femur (femoral condyles). It is flat, distally tapered, proximally curved, and has anterior and posterior surfaces, three borders and an apex which is the distal end of the bone. With the knee in extension, the apex is just proximal to the line of the knee joint.
JOINTS
SUPERIOR TIBIOFIBULAR JOINT
PATELLOFEMORAL JOINT
The patellofemoral joint is a synovial joint and is part of the knee joint.
Patellar tendon sheath and patellar tendon
The patellar tendon (patellar ligament) is the central band of the tendon of quadriceps femoris, and is continued distally from the patella to the tibial tuberosity (see Fig. 80.25). It is strong, flat and 6 to 8 cm in length. Proximally it is attached to the patellar apex and adjoining margins, to roughened areas on the anterior surface and to a depression on the distal posterior patellar surface. Distally it is attached to the superior smooth area of the tibial tuberosity. This insertion is oblique, and is more distal laterally. Its superficial fibres are continuous over the patella with the tendon of quadriceps femoris, the medial and lateral parts of which descend, flanking the patella, to the sides of the tibial tuberosity, where they merge with the fibrous capsule as the medial and lateral patellar retinacula. The patellar tendon is separated from the synovial membrane by a large infrapatellar fat pad and from the tibia by a bursa, and lies within its own well-defined sheath.
All other aspects of the patellofemoral joint are described with the tibiofemoral joint.
TIBIOFEMORAL JOINT
The tibiofemoral joint is a complex synovial joint and is part of the knee joint.
Articulating surfaces
Proximal tibial surface
The proximal tibial surface (often referred to as the tibial plateau) slopes posteriorly and downwards relative to the long axis of the shaft (Fig. 82.7). The tilt, which is maximal at birth, decreases with age, and is more marked in habitual squatters. The tibial plateau presents medial and lateral articular surfaces (facets) for articulation with the corresponding femoral condyles. The posterior surface, distal to the articular margin, displays a horizontal, rough groove to which the capsule and posterior part of the medial collateral ligament are attached. The anteromedial surface of the medial tibial condyle is a rough strip, separated from the medial surface of the tibial shaft by an inconspicuous ridge. The medial patellar retinaculum is attached to the medial tibial condyle along its anterior and medial surfaces, which are marked by vascular foramina.
Intercondylar area
The rough-surfaced area between the condylar articular surfaces is narrowest centrally where there is an intercondylar eminence, the edges of which project slightly proximally as the lateral and medial intercondylar tubercles. The intercondylar area widens behind and in front of the eminence as the articular surfaces diverge (Fig. 82.8).
Menisci
The menisci (semilunar cartilages) are crescentic, intracapsular, fibrocartilaginous laminae (Figs 82.9, 82.10). They serve to widen and deepen the tibial articular surfaces that receive the femoral condyles. Their peripheral attached borders are thick and convex and their free, inner borders thin and concave. Their peripheries are vascularized by capillary loops from the fibrous capsule and synovial membrane, while their inner regions are avascular. Tears of the menisci are common. Most occur in the avascular, inner zones and seldom heal spontaneously; if surgery is indicated, these menisci are best resected. Peripheral tears (i.e. in the vascularized zone) have the potential to heal satisfactorily if repaired surgically. The meniscal horns are richly innervated compared with the remainder of the meniscus. The central thirds are devoid of innervation (Gronblad et al 1985). The proximal surfaces are smooth and concave and in contact with the articular cartilage on the femoral condyles. The distal surfaces are smooth and flat, resting on the tibial articular cartilage. Each covers approximately two-thirds of its tibial articular surface. Canal-like structures open onto the surface of menisci in infants and young children and may transport nutrients to deeper avascular areas.
Two structurally different regions of the menisci have been identified. The inner two-thirds of each meniscus consists of radially organized collagen bundles, and the peripheral third consists of larger circumferentially arranged bundles (Ghadially et al 1983). The articular surfaces of the inner part are lined by thinner collagen bundles parallel to the surface, while the outer portion is covered by synovium. This structural arrangement suggests specific biomechanical functions for the two regions: the inner portion of the meniscus is suited to resisting compressive forces while the periphery is capable of resisting tensional forces. With ageing and degeneration, compositional changes occur within the menisci which reduce their ability to resist tensional forces. Outward displacement of the menisci by the femoral condyles is resisted by firm anchorage of the peripheral circumferential fibres to the intercondylar bone at the meniscal horns.
Medial meniscus
The medial meniscus broader posteriorly, is almost a semicircle in shape (Fig. 82.9). It is attached by its anterior horn to the anterior tibial intercondylar area in front of the anterior cruciate ligament; the posterior fibres of the anterior horn are continuous with the transverse ligament. The anterior horn is in the floor of a depression medial to the upper part of the patellar tendon. The posterior horn is fixed to the posterior tibial intercondylar area, between the attachments of the lateral meniscus and posterior cruciate ligament. Its peripheral border is attached to the fibrous capsule and the deep surface of the medial collateral ligament. The tibial attachment of the meniscus is known as the ‘coronary ligament’. Collectively these attachments ensure that the medial meniscus is relatively fixed and moves much less than the lateral meniscus.
Lateral meniscus
A discoid lateral meniscus occurs in up to 5% of the population, often bilaterally. The distinguishing features of a discoid lateral meniscus are its shape and posterior ligamentous attachments. The following classification of the abnormality is based on Watanabe et al (1979). In its mildest form, the partial discoid meniscus is simply a wider form of the normal lateral meniscus. The acute, medial free edge is interposed between femoral and tibial condyles, but it does not completely cover the tibial plateau. A complete discoid meniscus appears as a biconcave disc with a rolled medial edge and totally covers the lateral tibial plateau. The Wrisberg type of meniscus has the same shape as a complete discoid meniscus, but its only peripheral posterior attachment is by the meniscofemoral ligaments. The normal tibial attachment of the posterior horn of the lateral meniscus is lacking, but the posterior meniscofemoral ligament persists. As a result, this type of meniscus is attached anteriorly to the tibia and posteriorly to the femur, which renders the posterior horn unstable. Under these circumstances, the meniscus is liable to get caught between the femur and tibia: this accounts for the classic presenting symptom of the ‘clunking knee’ in some patients. The aetiology of discoid meniscus is not clear. Most are asymptomatic, and are often found by chance at arthroscopy. However, they may cause difficulty in gaining access to the lateral compartment at arthroscopy.
Transverse [intermeniscal] ligament
The transverse ligament connects the anterior convex margin of the lateral meniscus to the anterior horn of the medial meniscus (Figs 82.8, 82.9). It varies in thickness and is often absent. Its exact role is conjectural; presumably it helps to decrease tension generated in the longitudinal circumferential fibres of the menisci when the knee is subjected to load. A posterior meniscomeniscal ligament is sometimes present.
Meniscofemoral ligaments
The two meniscofemoral ligaments (MFLs) connect the posterior horn of the lateral meniscus to the inner (lateral) aspect of the medial femoral condyle (Figs 82.11, 82.12). The anterior MFL (aMFL; ligament of Humphry) passes anterior to the posterior cruciate ligament. The posterior MFL (pMFL; ligament of Wrisberg) passes behind the posterior cruciate ligament and attaches proximal to the margin of attachment of the posterior cruciate.
Anatomical studies found that at least one meniscofemoral ligament was present in 92% of cadaveric knees examined, whilst both coexisted in 32% (Gupte et al 2003). Biomechanical studies have revealed the cross-sectional area and strength of the meniscofemoral ligaments to be comparable to those of the posterior fibre bundle of the posterior cruciate ligament.
Soft tissues
Medial soft tissues
The medial soft tissues (see Fig. 80.25, Fig. 82.13) are arranged in three layers (Warren & Marshall 1979).
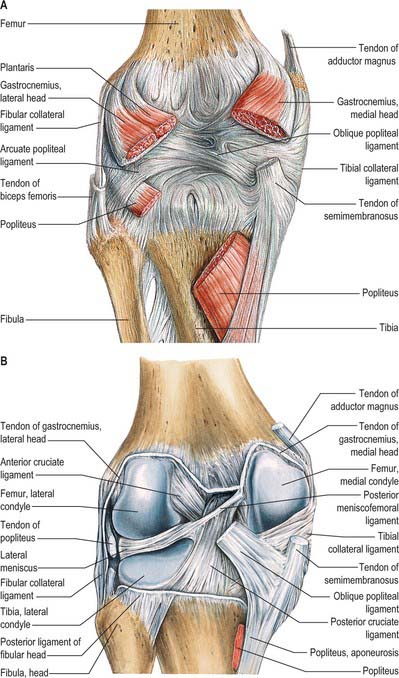
Fig. 82.13 Posterior dissection of the knee. A, Capsule intact. B, Capsule removed.
(From Sobotta 2006.)
Layer 1 is the most superficial and is the deep fascia that invests sartorius. The saphenous nerve and its infrapatellar branch are superficial to the fascia. Sartorius inserts into the fascia as an expansion rather than as a distinct tendon. The layer 1 fascia spreads inferiorly and anteriorly to lie superficial to the distinct and readily identifiable tendons of gracilis and semitendinosus and their insertions. The latter two tendons are commonly harvested for surgical reconstruction of damaged cruciate ligaments. To gain access to them the upper edge of sartorius can be identified. The sartorius (layer 1) fascia is then incised to reveal the tendons. Deep to the tendons is the pes bursa, which overlies the superficial medial collateral ligament: this bursa is sometimes the seat of inflammation, especially in track and field athletes. Posteriorly, layer 1 overlies the tendons of gastrocnemius and the structures of the popliteal fossa. Anteriorly, layer 1 blends with the anterior limit of layer 2 and the medial patellar retinaculum. More inferiorly, layer 1 blends with periosteum.
Lateral soft tissues
The lateral soft tissues (Figs 82.13, 82.15) are also arranged in three layers (Seebacher et al 1982). Most superficial is the lateral patellar retinaculum. The middle layer consists of the lateral collateral ligament, popliteofibular ligament, fabellofibular ligament and arcuate ligament. The deep layer is the lateral part of the capsule.
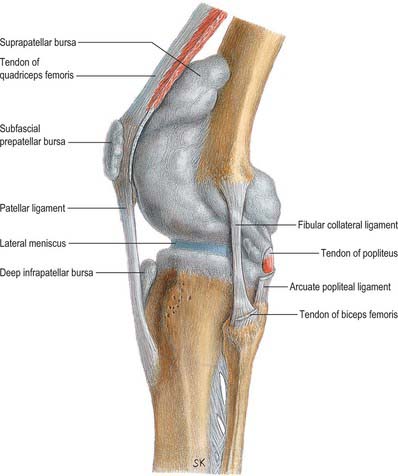
Fig. 82.15 Knee joint (lateral aspect); the synovial cavity is distended and synovial membrane appears grey.
(From Sobotta 2006.)
The fabellofibular ligament is a condensation of fibres that runs from the fabella, or from the lateral head of gastrocnemius if the fabella is absent, to the fibular styloid. The arcuate ligament is a condensation of fibres that runs from the fibular styloid, posteromedially over the emerging tendon of popliteus below the level of the tibial joint surface, to the tibial intercondylar area. The lateral joint capsule is thin and blends posteriorly with the arcuate ligament. Anteriorly it forms the weak, lax coronary ligament, which attaches the inferior border of the meniscus to the lateral tibia.
Ligaments
Cruciate ligaments
The cruciate ligaments, so named because they cross each other, are very strong intracapsular structures. The point of crossing is located a little posterior to the articular centre. They are named anterior and posterior with reference to their tibial attachments (Fig. 82.14). Synovial membrane almost surrounds the ligaments but is reflected posteriorly from the posterior cruciate to adjoining parts of the capsule: the intercondylar part of the posterior region of the fibrous capsule therefore has no synovial covering.
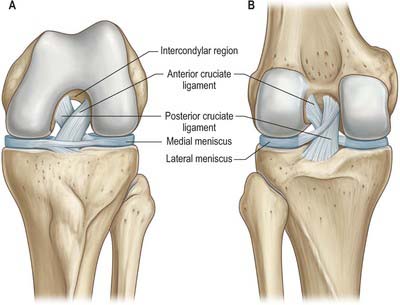
Fig. 82.14 The left knee joint. A, Anterior aspect in full flexion. B, Posterior aspect in extension.
(From Drake, Vogl, Mitchell, Tibbitts and Richardson 2008.)
Anterior cruciate ligament
The anterior cruciate ligament is attached to the anterior intercondylar area of the tibia, just anterior and slightly lateral to the medial tibial eminence, partly blending with the anterior horn of the lateral meniscus (Figs 82.8, 82.9). It ascends posterolaterally, twisting on itself and fanning out to attach high on the posteromedial aspect of the lateral femoral condyle (Girgis et al 1975). The average length and width of an adult anterior cruciate ligament are 38 mm and 11 mm respectively. It is formed of two, or possibly three, functional bundles that are not apparent to the naked eye, but can be demonstrated by microdissection techniques. The bundles are named anteromedial, intermediate, and posterolateral, according to their tibial attachments (Amis and Dawkins 1991).
Posterior cruciate ligament
The posterior cruciate ligament is thicker and stronger than the anterior cruciate ligament (Fig. 82.9), the average length and width of an adult posterior cruciate ligament being 38 mm and 13 mm respectively. It is attached to the lateral surface of the medial femoral condyle and extends up onto the anterior part of the roof of the intercondylar notch, where its attachment is extensive in the anteroposterior direction. Its fibres are adjacent to the articular surface. They pass distally and posteriorly to a fairly compact attachment posteriorly in the intercondylar region and in a depression on the adjacent posterior tibia. This gives a fan-like structure in which fibre orientation is variable. Anterolateral and posteromedial bundles have been defined: they are named (against convention) according to their femoral attachments. The anterolateral bundle tightens in flexion whilst the posteromedial is tight in extension of the knee. Each bundle slackens as the other tightens. Unlike the anterior cruciate ligament, it is not isometric during knee motion, i.e. the distance between attachments varies with knee position. The posterior cruciate ligament ruptures less commonly than the anterior cruciate; rupture is usually better tolerated by patients than that of the anterior cruciate ligament.
Synovial membrane, plicae and fat pads
The synovial membrane of the knee is the most extensive and complex in the body. It forms a large suprapatellar bursa between quadriceps femoris and the lower femoral shaft proximal to the superior patellar border (Fig. 82.16). The bursa is an extension of the joint cavity. The attachment of articularis genu to its proximal aspect prevents the bursa from collapsing into the joint. Alongside the patella the membrane extends beneath the aponeuroses of the vasti, especially under vastus medialis. It extends proximally a hand’s breadth above the superior pole of the patella. Distal to the patella, the synovial membrane is separated from the patellar tendon by an infrapatellar fat pad. Where it lies beneath the fat pad, the membrane projects into the joint as two fringes, alar folds, which bear villi. The folds converge posteriorly to form a single infrapatellar fold or plica (ligamentum mucosum), which curves posteriorly to its attachment in the femoral intercondylar fossa (Fig. 82.17). This fold may be a vestige of the inferior boundary of an originally separate femoropatellar joint. The extent of the infrapatellar plica ranges from a thin cord to a complete sheet that can obstruct the passage of instruments during knee arthroscopy. When substantial, it has been mistaken for the anterior cruciate ligament, which is directly posterior to it. The medial plica extends in the midline anteriorly from the medial alar fold medially to the suprapatellar pouch. Occasionally it can be thickened and inflamed, usually following acute or chronic trauma.
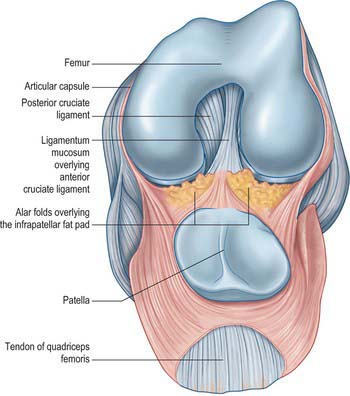
Fig. 82.17 Left knee joint in full flexion: the quadriceps tendon has been sectioned and the patellar flap retracted distally. Compare with Fig. 82.14A.
The infrapatellar fat pad is the largest part of a circumferential extrasynovial fatty ring which extends around the patellar margins (Newell 1991).
Bursae
Numerous bursae are associated with the knee. Anteriorly, there is a large subcutaneous prepatellar bursa between the lower half of the patella and skin; a small deep infrapatellar bursa between the tibia and patellar tendon; a subcutaneous infrapatellar bursa between the distal part of the tibial tuberosity and skin; and a large suprapatellar bursa which is the superior extension of the knee joint cavity (Fig. 82.16). Posterolaterally, there are bursae between the lateral head of gastrocnemius and the joint capsule (this bursa is sometimes continuous with the joint cavity); the fibular collateral ligament and the tendon of biceps femoris; the fibular collateral ligament and the tendon of popliteus; the tendon of popliteus and the lateral femoral condyle, which is usually an extension of the synovial cavity of the joint. The last two bursae may communicate with each other.
Movements
Conjunct medial rotation of the femur on the tibia in the later stages of extension is part of a ‘locking’ mechanism, the so-called ‘screw-home movement’, which is an asset when the fully extended knees are subjected to strain. Full extension results in the close-packed position, with maximal spiralization and tightening of the ligaments. The roles of the articular surfaces, musculature and ligaments in generating conjunct rotations remain controversial (Girgis et al 1975, Rajendran 1985), but the following points can be made. The lateral combined meniscotibial ‘receiving surface’ is smaller, more circular and more deeply concave. Since the articular surface is virtually convex in sagittal section the depth of the receiving surface is largely due to the presence of the lateral meniscus. The lateral femoral articular surface is also smaller. Consequently, the lateral femoral condyle approaches full congruence with the opposed surface some 30° before full extension (well before the medial condyle). Simple extension cannot continue, but medial rotation of the femur occurs on a vertical axis through its head and medial condyle: the medial femoral condyle moves very little in the sagittal plane and is stabilized by the ‘upslope’ of the anterior half of the medial tibia, while rotation of the lateral femoral condyle and meniscus brings the anterior horn of the latter onto the anterior ‘downslope’ of the lateral tibial condyle. Rotation and extension follow simultaneously and smoothly until final close packing of both condyles is accomplished. At the beginning of flexion from full extension (with the foot fixed) lateral femoral rotation occurs, which ‘unlocks’ the joint. While joint surfaces and many ligaments are involved, electromyographic evidence reveals that contraction of popliteus is important, and that it pulls down and backwards on the lateral femoral condyle, lateral to the axis of femoral rotation. It also retracts the posterior horn during lateral rotation and continuing flexion, via its attachment to the lateral meniscus, and so prevents traumatic compression.
Any position of extension adopted is a balance between forces (torque) extending the joint and passive mechanisms resisting them. The range near to close packing is functionally important. In symmetrical standing, the line of body weight is anterior to the transverse axes of the knee joints, but the passive mechanisms noted above preserve posture with minimal muscular effort (Joseph 1960). Active contraction of the extensors and a close-packed position only occur in asymmetrical postures, e.g. in leaning forward, heavy loading, or when powerful thrust is needed.
In extension, parts of both cruciate ligaments, the tibial and fibular collateral ligaments, the posterior capsular region, the oblique popliteal ligament, skin and fasciae are all taut. Passive and sometimes active tension exists in the hamstrings and gastrocnemius, and the anterior part of the medial meniscus is compressed between the femoral and tibial condyles. During extension the patellar tendon is tightened by quadriceps femoris but is relaxed in the erect attitude. When the knee flexes, the fibular collateral ligament and the posterior part of the medial collateral ligament relax but the cruciate ligaments and the anterior part of the medial collateral ligament remain taut: the posterior parts of the menisci are compressed between the femoral and tibial condyles. Flexion is checked by quadriceps femoris, anterior parts of the capsule, posterior cruciate ligament and compression of soft tissues behind the knee. In extreme passive flexion, contact of the calf with the thigh may be the limiting factor and parts of both cruciate ligaments are also tense. In addition to conjunct rotation with terminal extension or initial flexion, relaxed collateral ligaments also allow independent medial and lateral rotation (adjunct rotation) when the joint is flexed.
Muscles producing the movements
Factors maintaining stability
The control of the stability of the knee is of considerable importance.
BIOMECHANICS OF THE KNEE
KNEE JOINT KINEMATICS
The surfaces of the tibia and femur are not as conforming as those of the relatively congruent hip joint. Although this variation in geometry permits motion to occur in six degrees of freedom (Fig. 82.18), the primary motion of the knee occurs in the sagittal plane, and a relatively minor degree of movement occurs in the transverse plane. The knee joint may therefore be described simplistically as a modified hinge joint allowing flexion–extension and a measure of rotatory motion. Knee motion is normally defined as starting from 0° (the neutral position), when the tibia and femur are in line in the sagittal plane. Biomechanically, it is important that the knee reaches the neutral position in extension, because that allows the leg to support the body weight like a simple strut when standing still. When standing upright, if the knee is flexed, the vertical line of action of the body weight passes posterior to the centre of rotation of the knee, tending to cause the body to tilt posteriorly. To counterbalance this, continuous quadriceps contraction is required, causing expenditure of energy.
The most frequently employed knee movement occurs when walking (Fig. 82.19). When the leg is swinging past the supporting leg, the knee must be flexed in order to avoid dragging the toes on the ground; this requires approximately 67° knee flexion. When the swinging leg approaches the first contact with the ground, the knee extends, to move the foot forwards for heel strike. If the knee remained extended, this would then cause the body to move in a circular arc, centred at the ankle, causing the centre of gravity to move upwards and then back down again, leading to more energy expenditure. It would also increase the forces on the knee, because the leg would act more like a rigid strut unable to dissipate the impact forces when the foot hit the ground. All these problems are overcome by the knee flexing 15° in the mid stance phase: the centre of gravity of the body can move forwards at approximately constant height, and the impact energy is absorbed by stretching the quadriceps (Fig. 82.19).
Tibial internal-external rotation also occurs during gait: the tibia rotates externally during terminal extension, a phenomenon known as ‘screw home’ (Fig. 82.20). It is surmised that this rotation helps to lock the geometry and tighten the soft tissues, thereby maintaining the knee in a stable position prior to the impact load of weight bearing. The knee acts as one link in a chain of limb segments, and this screw-home relates to rotation of both foot and hip. When the foot is swung forwards for heel strike, the pelvis rotates so that the hip is moved forwards, a movement that entails external rotation of the hip. During stance, the femur is internally rotated against the locked knee. Tibial external rotation also causes inversion of the foot at the subtalar joint, raising the arch and locking the structure of the foot. The knee flexion that occurs after the impact on the ground allows the tibia to rotate internally so that the foot everts, softening its structure and allowing it to deform and absorb energy. Conversely, towards toe-off, the knee extends, rotating the tibia externally, so the foot is again a rigid lever with which to push the body forwards.
Articular kinematics
Sagittal sections of the knee reveal that the arcs of the femoral condyles are much longer than the anterior–posterior length of the tibial plateau. This means that if the knee flexed with a purely rolling motion, then the femur would roll off of the back of the tibia long before the knee reaches full flexion (Fig. 82.20). This does not happen because the femur slides anteriorly at the same time as it rolls posteriorly, and thus remains in correct articulation. If the knee were to possess a fully conforming roller-in-trough geometry, as in the humeroulnar joint, then flexion would occur by pure sliding movement between the joint surfaces. This conformity is not possible at the knee because it would inhibit the tibial internal-external rotation that is needed during locomotion.
In the locked, fully-extended knee, the anterodistal femoral articular surfaces press onto the anterior horns of the menisci. This tends to cause the femur to slide posteriorly, tensing the ACL and slackening the PCL. With knee flexion, the femur lifts off of the anterior horns of the menisci, leading to contact between the smaller radii of the posterior parts of the femoral condyles, and the tibial plateau plus posterior horns of the menisci. This means that the centre of contact moves posteriorly in early knee flexion. After this, the femoral condyles have approximately circular sagittal sections. The femur is now prevented from rolling back any further by tension in the ACL. The action of the ACL is similar to that of a rope attaching the back of a car to the ground: when the car tries to drive away, the wheels roll until the rope tightens, and then spin in one position (sliding motion).
Articular mechanics
Compression
The compressive force is distributed over an area to produce a contact pressure (contact stress). The contact pressure is, therefore, dependent on the area of contact as well as the load itself. Fully-conforming articular surface geometry would allow the greatest area of contact, and thus would minimize contact pressure, however, this is not present in the knee. The medial compartment of the knee is semi-conforming with a convex femoral condyle articulating over a concave medial tibial plateau. The lateral compartment has less conformity: the lateral tibial plateau is flat or slightly convex in sagittal sections. These different shapes reflect the differential movement of the medial and lateral compartments. In normal movement, the screw home rotation of the tibia occurs about a medial axis which means that most of the rotation of the tibia is due to an anterior–posterior translation of the lateral compartment (Fig. 82.21).
In order to maintain some degree of conformity, and thus minimize contact pressure, the menisci have wedge-shaped cross-sections that fit between the femur and tibia around the bones. This serves to increase the area over which the compressive force on the knee is distributed. In the absence of the menisci, the load is carried by a much smaller area of cartilage, resulting in higher contact stresses on the articular cartilage. This explains the prevalence of osteoarthrosis following meniscectomy (Fig. 82.22).
The distal aspect of the femur, resting on the menisci in the extended knee, has a large radius of curvature, and so it fits against the entire area of the menisci. However, as the knee flexes, the smaller radii of the posterior parts of the femoral condyles cause the femur to lift off the anterior horns of the menisci, and the femoral contact is now solely on the posterior horns in the flexed knee. This, combined with the large joint forces that are generated when arising from a seated position, explains why there are often spontaneous tears of the posterior horns in older patients, when standing up from a squatting position.
The load-carrying mode of the menisci is shown in Fig. 82.23. When the femoral condyle presses down on the meniscus, it tends to squeeze the meniscus out of the joint, because of its tapered cross-section. This causes the diameter of the circular shape of the meniscus (and therefore the meniscal circumference) to increase. This is resisted by ‘hoop tension’ in strong fibres that pass around the periphery of the meniscus, and transmit the tension to the tibial plateau via strong insertional ligaments. Because the tissue is adapted to resist hoop stresses, it has much greater hoop strength (approximately 100 MPa) than radial strength (approximately 3 MPa), which explains why bucket handle tears occur. It also explains why a circumferential tear does not have such a serious effect on meniscal function, because the circumferential fibres can still transmit the loads, whereas a radial tear breaks the load-carrying fibres.
Friction
During walking, every step involves phases of action that vary the loading and velocity conditions at the knee joint. A variety of lubrication mechanisms normally prevent joint surface damage. Thus, in the swing phase, when the foot is off the ground, the joint surfaces are loaded lightly, and have high relative velocity while the knee flexes and extends. This allows the synovial fluid to separate the surfaces, giving fluid film lubrication, with very low friction and no wear (Fig. 82.24). At the time of heel strike, a large impact load acts to compress the surfaces together. At a microscopic level, the surfaces do not come into contact, because of the squeeze film effect: in essence, the impact occurs so rapidly (less than 0.1 sec) that the synovial fluid cannot all be squeezed out of the joint space, because of its viscosity and the narrowness of the space. In the mid stance phase, the flexion–extension motion again entrains the synovial fluid in between the joint surfaces, producing what is known as a hydrodynamic effect: the fluid is trapped between the surfaces by the motion, and therefore it acts to separate them. Finally, when the foot is pushing off the body weight, there is little motion, and the thin fluid film diminishes under the compressive load. If the squeeze-film effect is insufficient, then the joint surfaces would now come into direct contact, were it not for the fact that the synovial fluid contains large protein molecules that are trapped on the cartilage surfaces when the fluid is expelled. This molecular layer acts as a boundary lubricant, protecting the cartilage in the same way that grease protects a synthetic bearing.
SOFT-TISSUE MECHANICS
Primary and secondary restraints
On testing the laxity in any of the degrees of freedom of the joint (e.g. in the anterior drawer test, a bedside clinical test in which the subject is placed in the supine position with the knee to be tested flexed to 80–90° before passive forward traction is applied to the tibia), there are usually combinations of ligaments that are tensed. Some of these are better aligned to resist the applied load or displacement. These are termed primary restraints, and are exemplified by the cruciate ligaments and the medial and lateral collateral ligaments. Secondary restraints are less well-aligned, but still have a significant restraining effect. These are exemplified by the menisci, and by the meniscofemoral ligaments. In the example in Fig. 82.25, the ACL is well-aligned to resist the applied anterior drawer force. With an absent ACL, the superficial MCL can resist the applied force, however, it does so by being loaded to a much higher level than the original loading on the ACL. The size of the lines in the vector diagram demonstrate this principle: although joint laxity may remain normal initially following rupture of a primary restraint, it may subsequently result in the overload of a secondary restraint, and ultimately, in further soft-tissue failure.
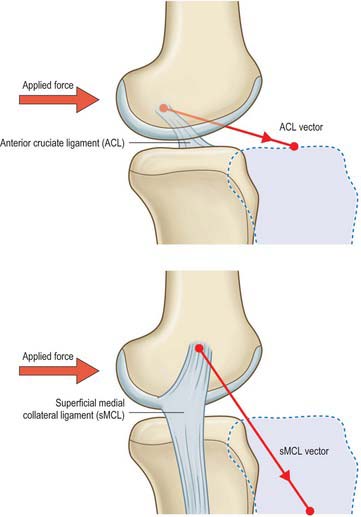
Fig. 82.25 Primary and secondary restraints to anteroposterior forces – example of the ACL and superficial MCL.
In the frontal plane the quadriceps muscles and patellar tendon tensions combine to cause a lateralizing force vector termed the Q-angle effect. The Q angle is defined as the difference between the resultant force vector of the quadriceps, which is normally parallel to the femoral shaft, and the patellar tendon (Fig. 82.26). Clinically, the Q angle is changed by the position of hip rotation, tibial rotation, and quadriceps tension. The clinical Q-angle is 12–15° (males) and 15–18° (females), which means that there is a greater lateralizing force vector on the patellofemoral joint in females. Contraction of the quadriceps, therefore, tends to displace the patella laterally; this is resisted by the geometry of the joint and by the passive stabilizers. Vastus medialis obliquus (VMO) acts medially and posteriorly as much as it acts proximally, and so its tension helps to resist the Q angle effect.
MUSCLES
The majority of muscles that act on the knee joint are described in either Chapter 80 (quadriceps femoris, semimembranosus, biceps femoris, semitendinosus, articularis genu) or Chapter 83 (gastrocnemius). Popliteus is described below.
Popliteus
An additional head may arise from the sesamoid in the lateral head of gastrocnemius. Very rarely, two other muscles may be found deeply situated behind the knee. Popliteus minor runs from the posterior surface of the lateral tibial condyle, medial to plantaris, to the oblique popliteal ligament. Peroneotibialis runs deep to popliteus from the medial side of the fibular head to the upper end of the soleal line.
VASCULAR SUPPLY AND LYMPHATIC DRAINAGE
ARTERIES
There is an intricate arterial anastomosis around the patella and femoral and tibial condyles. A superficial network spreads between the fascia and skin around the patella and in the fat deep to the patellar tendon. A deep network lies on the femur and tibia near the adjoining articular surfaces, and supplies the bone and marrow, the articular capsule, synovial membrane and the cruciate ligaments (Fig. 82.1). The vessels involved are the superior, middle and inferior genicular branches of the popliteal artery, descending genicular branches of the femoral artery, the descending branch of the lateral circumflex femoral artery, the circumflex fibular artery and the anterior and posterior tibial recurrent arteries. For details consult Scapinelli (1968).
Popliteal artery
The popliteal artery is the continuation of the femoral artery and crosses the popliteal fossa (Fig. 82.4). It descends laterally from the opening in adductor magnus to the femoral intercondylar fossa, inclining obliquely to the distal border of popliteus, where it divides into the anterior and posterior tibial arteries. This division usually occurs at the proximal end of the asymmetrical crural interosseous space between the wide tibial metaphysis and the slender fibular metaphysis. The artery is relatively tethered at the adductor magnus hiatus and again distally by the fascia related to soleus, and is therefore susceptible to traction damage in knee injuries, e.g. dislocation.
Branches (other than terminal)
There is an intricate arterial anastomosis around the patella and femoral and tibial condyles (Fig. 82.1). A superficial network spreads between the fascia and skin around the patella and in the fat deep to the patellar tendon. A deep network lies on the femur and tibia near the adjoining articular surfaces, and supplies the bone and marrow, articular capsule and synovial membrane. The vessels involved are the medial and lateral superior genicular, the medial and lateral inferior genicular, the descending genicular, the descending branch of the lateral circumflex femoral, the circumflex fibular and the anterior and posterior tibial recurrent arteries.
The superior genicular arteries branch from the popliteal artery, curving round proximal to both femoral condyles to reach the anterior aspect of the knee. The medial superior genicular artery lies under semimembranosus and semitendinosus, proximal to the medial head of gastrocnemius and deep to the tendon of adductor magnus. It divides into a branch to vastus medialis that anastomoses with the descending genicular and medial inferior genicular arteries, and a branch that ramifies on the femur and anastomoses with the lateral superior genicular artery. Its size varies inversely with that of the descending genicular artery. The lateral superior genicular artery passes under the tendon of biceps femoris and divides into superficial and deep branches. The superficial branch supplies vastus lateralis and anastomoses with the descending branch of the lateral circumflex femoral and lateral inferior genicular arteries, while the deep branch anastomoses with the medial superior genicular artery, forming an anterior arch across the femur with the descending genicular artery. The superficial branch is vulnerable if the lateral patellar retinaculum is divided surgically.
Cutaneous branches: the superficial sural arteries
Fasciocutaneous free and pedicled flaps may be raised on the superficial sural arteries.
VEINS
Popliteal vein
The popliteal vein ascends through the popliteal fossa to the opening in adductor magnus where it becomes the femoral vein (see Fig. 79.9B). Its relationship to the popliteal artery changes as the vein ascends. Distally it is medial to the artery, between the heads of gastrocnemius it is superficial (posterior) to it, and proximal to the knee joint it is posterolateral to the artery. Its tributaries are the short saphenous vein, veins corresponding to branches of the popliteal artery, and muscular veins, including a large branch from each head of gastrocnemius. There are usually four or five valves in the popliteal vein.
Short saphenous vein
The short saphenous vein (small saphenous vein) begins posterior to the lateral malleolus as a continuation of the lateral marginal vein (see Fig. 79.9B). In the lower third of the calf it ascends lateral to the calcaneal tendon, lying on the deep fascia and covered only by superficial fascia and skin. Inclining medially to reach the midline of the calf, it penetrates the deep fascia, within which it ascends on gastrocnemius, only emerging between the deep fascia and gastrocnemius gradually at about the junction of the intermediate and proximal thirds of the calf (usually well below the lower limit of the popliteal fossa). Continuing its ascent, it passes between the heads of gastrocnemius then proceeds to its termination in the popliteal vein in the popliteal fossa, 3–7.5 cm above the knee joint.
INNERVATION
The knee joint is innervated by branches from the obturator, femoral, tibial and common fibular nerves (Freeman & Wyke 1967). The genicular branch of the obturator nerve is the terminal branch of its posterior division. Muscular branches of the femoral nerve, especially to vastus medialis, supply terminal branches to the joint. Genicular branches from the tibial and common fibular nerves accompany the genicular arteries: those from the tibial nerve run with the medial and middle genicular arteries, while those from the common fibular nerve run with the lateral genicular and anterior tibial recurrent arteries.
The femoral and obturator nerves are described on page 1382–1383, and the tibial and common fibular nerves are described on page 1427).
Saphenous nerve
The saphenous nerve is the largest and longest cutaneous branch of the femoral nerve. It descends lateral to the femoral artery in the femoral triangle and enters the adductor canal, where it crosses in front of the artery to lie medial to the artery. At the distal end of the canal it leaves the artery and emerges through the aponeurotic covering with the saphenous branch of the descending genicular artery. As it leaves the adductor canal it gives off an infrapatellar branch that contributes to the peripatellar plexus (p. 1393) and then pierces the fascia lata between the tendons of sartorius and gracilis, becoming subcutaneous to supply the prepatellar skin. It descends along the medial tibial border with the long saphenous vein and divides distally into a branch which continues along the tibia to the ankle and a branch which passes anterior to the ankle to supply the skin on the medial side of the foot, often as far as the first metatarsophalangeal joint. The saphenous nerve connects with the medial branch of the superficial fibular nerve. Near midthigh, it gives a branch to the subsartorial plexus. The nerve may be subject to an entrapment neuropathy as it leaves the adductor canal.
Amis AA, Dawkins GP. Functional anatomy of the anterior cruciate ligament. Fibre bundle actions related to ligament replacements and injuries. J Bone Joint Surg. 1991;73B:260-267.
Cormack GC, Lamberty BGH. The Arterial Anatomy of Skin Flaps. Edinburgh: Churchill Livingstone, 1994.
Crock HV. The blood supply of the lower limb bones in man. London: Livingstone, 1967.
Freeman MAR, Wyke B. The innervation of the knee joint. J Anat. 1967;101:505-532.
Ghadially FN, Lalonde J-M A, Wedge JH. Ultrastructure of normal and torn menisci of the human knee joint. J Anat. 1983;136:773-791.
Girgis FG, Marshall JL, Al Monajem ARS. The cruciate ligaments of the knee joint. Clin Orthop. 1975;106:216-231.
Gronblad M, Korkala O, Leisi P, Karaharju E. Innervation of synovial membrane and meniscus. Acta Orthop Scand. 1985;56:484-486.
Gupte CM, Bull AMJ, Thomas RD, Amis AA. A review of the function and biomechanics of the meniscofemoral ligaments. Arthroscopy. 2003;19:161-171.
A comprehensive review of the properties of these hitherto poorly understood ligaments..
Joseph J. Man’s Posture: Electromyographic Studies. Springfield, Illinois: Thomas, 1960.
Newell RLM. A complete intra-articular fat pad around the human patella. J Anat. 1991;179:232.
Shows that the infrapatellar and suprapatellar fat pads are not isolated and separate structures..
Rajendran K. Mechanism of locking at the knee joint. J Anat. 1985;143:189-194.
Scapinelli R. Studies on the vasculature of the human knee joint. Acta Anat. 1968;70:305-331.
Seebacher JR, Inglis AE, Marshall JL, Warren RF. The structure of the posterolateral aspect of the knee. J Bone Joint Surg. 1982;64A:536-541.
Work that established the current interpretation of the anatomy of the soft tissues of the knee..
Tennant TD, Birch NC, Holmes MJ, Birch R, Goddard NJ. Knee pain and the infrapatellar branch of the saphenous nerve. J Roy Soc Med. 1998;91:573-575.
Recognizes the clinical significance of this small but important nerve branch..
Thomas P, Jackson AM, Aichroth PM. Congenital absence of the anterior cruciate ligament. J Bone Joint Surg. 1985;67B:572-575.
A rare but important congenital anomaly..
Warren LF, Marshall JL. The supporting structures and layers on the medial side of the knee: an anatomical analysis. J Bone Joint Surg. 1979;61A:56-62.
Watanabe M, Takeda S, Ikeuchi H. Atlas of Arthroscopy. Berlin: Springer-Verlag, 1979.