CHAPTER 74 Kidney and ureter
KIDNEY
In the fresh state, the kidneys are reddish-brown. They are situated posteriorly behind the peritoneum on each side of the vertebral column and are surrounded by adipose tissue. Superiorly they are level with the upper border of the 12th thoracic vertebra, inferiorly with the third lumbar vertebra. The right is usually slightly inferior to the left, reflecting its relationship to the liver. The left is a little longer and narrower than the right and lies nearer the median plane (Fig. 74.1). The long axis of each kidney is directed inferolaterally and the transverse axis posteromedially, which means that the anterior and posterior aspects usually described are in fact anterolateral and posteromedial. An appreciation of this orientation is important in percutaneous and endo-urologic renal surgery.
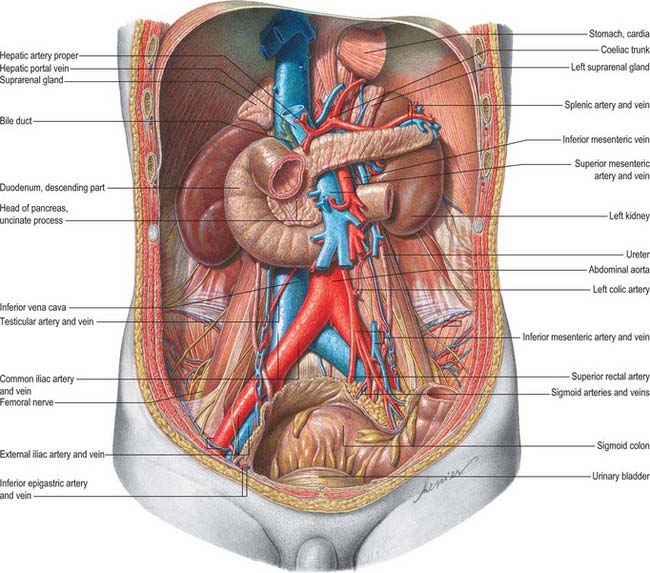
Fig. 74.1 Relationships of the kidneys and ureters in the male retroperitoneum.
(From Sobotta 2006.)
Very rarely, and despite the normal location of the ureteric orifices within the bladder, the two renal masses may be on the same side. This is termed crossed renal ectopia and usually the two renal masses are fused in such circumstances. A solitary crossed renal ectopia may be associated with skeletal and other genitourinary anomalies. A number of different anatomical patterns can result, all of which are extremely rare (Fig. 74.2).
Horseshoe kidneys are found in 1 in 400 individuals. A transverse bridge of renal tissue, the isthmus, which usually but not invariably contains functioning renal substance, connects the two renal masses. The isthmus lies between the inferior poles, most commonly anterior to the great vessels. The ureters curve anterior to the isthmus and often have a high insertion into the renal pelvis (Fig. 74.3A,B).
PERIRENAL FASCIA
The perirenal fascia is a dense, elastic connective tissue sheath which envelops each kidney and suprarenal gland together with a layer of surrounding perirenal fat (Fig. 74.4A,B; see Fig. 62.1). The kidney and its vessels are embedded in perirenal fat, which is thickest at the renal borders and extends into the renal sinus at the hilum.
The perirenal fascia was originally described as being made up of two separate entities, the posterior fascia of Zuckerkandl and the anterior fascia of Gerota, which fused laterally to form the lateral conal fascia. According to this view, the lateral conal fascia continued anterolaterally behind the colon to blend with the parietal peritoneum. However, work by Mitchell (1950) showed that the perirenal fascia is not made up of distinct fused fasciae, but is in fact a single multilaminated structure which is fused posteromedially with the muscular fasciae of psoas major and quadratus lumborum. It then extends anterolaterally behind the kidney as a bilaminated sheet, which at a variable point divides into a thin anterior lamina, passing around the front of the kidney as the anterior perirenal fascia, and a thicker posterior lamina which continues anterolaterally as the lateral conal fascia, fusing with the parietal peritoneum.
There is some debate concerning the inferior fusion of the perirenal fascia. Many investigators believe that inferiorly the anterior and posterior leaves of the perirenal fascial fuse to produce an inverted cone which is open to the pelvis at its apex. Laterally the anterior and posterior leaves fuse with the iliac fascia, and medially they fuse with the periureteric connective tissue. The inferior apex of the cone is open anatomically towards the iliac fossa but rapidly becomes sealed in inflammatory disease. An alternative view is based on the dissection of recently deceased cadavers after injections of coloured latex into the perirenal space: these have shown that the anterior and posterior perirenal fasciae merge to form a single multilaminar fascia which contains the ureter in the iliac fossa. Anteriorly this common fascia is loosely connected to the parietal peritoneum, and so denies free communication between the perirenal space and the pelvis, and also denies communication between the perirenal and pararenal spaces.
RELATIONS
The superior poles of both kidneys are thick and round and each is related to its suprarenal gland (Fig. 74.5A,B). The inferior poles are thinner and extend to within 2.5 cm of the iliac crests. The lateral borders are convex. The medial borders are convex adjacent to the poles, concave between them and slope inferolaterally. In each a deep vertical fissure opens anteromedially as the hilum, which is bounded by anterior and posterior lips and contains the renal vessels and nerves and the renal pelvis. The relative positions of the main hilar structures are the renal vein (anterior), the renal artery (intermediate) and the pelvis of the kidney (posterior). Usually an arterial branch from the main renal artery runs over the superior margin of the renal pelvis to enter the hilum on the posterior aspect of the pelvis, and a renal venous tributary often leaves the hilum in the same plane. Above the hilum the medial border is related to the suprarenal gland and below to the origin of the ureter.
The convex anterior surface of the kidney actually faces anterolaterally and its relations differ on the right and left. Likewise the posterior surface of the kidneys in reality faces posteromedially. Its relations are similar on both sides of the body (Fig. 74.6A–D).
A small medial area of the superior pole is related to the left suprarenal gland. The lateral half of the anterior surface is related to the spleen from which it is separated by a layer of peritoneum. A central quadrilateral area lies in direct contact with the retroperitoneal pancreas and the splenic vessels. Above this a small variable triangular region, between the suprarenal and splenic areas, is in contact with the stomach separated by a layer of peritoneum. Below the pancreatic and splenic areas, a narrow lateral strip which extends to the lateral border of the kidney is directly related to the retroperitoneal left colic flexure and the beginning of the descending colon. An extensive medial area is related to intraperitoneal loops of jejunum. The gastric area is covered with the peritoneum of the lesser sac (omental bursa) and the splenic and jejunal areas are covered by the peritoneum of the greater sac. Behind the peritoneum covering the jejunal area, branches of the left colic vessels are related to the kidney (Fig. 74.7A,B).
INTERNAL MACROSTRUCTURE
The postnatal kidney has a thin fibrous capsule composed of collagen-rich tissue with some elastic and smooth muscle fibres. In renal disease the capsule may become adherent (Fig. 74.8A,B).
The renal cortex is subcapsular, arching over the bases of the pyramids and extending between them towards the renal sinus as renal columns. Its peripheral regions are termed cortical arches and are traversed by radial, lighter-coloured medullary rays, separated by darker tissue, the convoluted part. The rays taper towards the renal capsule and are peripheral prolongations from the bases of renal pyramids. The cortex is histologically divisible into outer and inner zones. The inner zone is demarcated from the medulla by tangential blood vessels (arcuate arteries and veins), which lie at the junction of the two, however a thin layer of cortical tissue (subcortex) appears on the medullary side of this zone. The cortex close to the medulla is sometimes termed the juxtamedullar cortex.
Renal pelvis and calyces
The hilum of the kidney leads into a central renal sinus, lined by the renal capsule and almost filled by the renal pelvis and vessels, the remaining space being filled by fat. Dissection into this plane can be challenging but is important in surgery on the renal pelvis, particularly open stone surgery. Within the renal sinus, the collecting tubules of the nephrons of the kidney open onto the summits of the renal papillae to drain into minor calyces, which are funnel-shaped expansions of the upper urinary tract. The renal capsule covers the external surface of the kidney and continues through the hilum to line the sinus and fuse with the adventitial coverings of the minor calyces. Each minor calyx surrounds either a single papilla or, more rarely, groups of two or three papillae. The minor calyces unite with their neighbours to form two or possibly three larger chambers, the major calyces. There is wide variation in the arrangement of the calyces. As the posterior aspect of the kidney rotates laterally during its ascent in utero, the calyces which were lateral in utero become positioned anteriorly, and the medial calyces move more posteriorly. The calyces drain into the infundibula. The renal pelvis is normally formed from the junction of two infundibula, one from the upper and one from the lower pole calyces, but there may be a third, which drains the calyces in the mid-portion of the kidney. The calyces are usually grouped so that three pairs drain into the upper pole infundibulum and four pairs into the lower pole infundibulum. If there is a middle infundibulum, the distribution is normally three pairs at the upper pole, two in the middle, and two at the lower pole. There is considerable variation in the arrangement of the infundibula and in the extent to which the pelvis is intrarenal or extrarenal. The funnel-shaped renal pelvis tapers as it passes inferomedially, traversing the renal hilum to become continuous with the ureter (Fig. 74.8A,B; see Fig. 74.9). It is rarely possible to determine precisely where the renal pelvis ceases and the ureter begins: the region is usually extrahilar and normally lies adjacent to the lower part of the medial border of the kidney. Rarely, the entire renal pelvis has been found to lie inside the sinus of the kidney so that the pelviureteric region occurs either in the vicinity of the renal hilum or completely within the renal sinus.
The calyces, renal pelvis and ureter are well-demonstrated radiologically following an intravenous injection of radio-opaque contrast which is excreted in the urine (intravenous urography – IVU) (Fig. 74.9A,B); or after the introduction of radio-opaque contrast into the ureter by catheterization through a cystoscope (ascending or retrograde pyelography. Normal cupping of the minor calyces by projecting renal papillae may be obliterated by conditions that cause hydronephrosis, chronic distension of the ureter and renal pelvis due to upper or lower urinary tract obstruction resulting in elevated intrapelvic pressure. An appreciation of the rotation of the kidneys which results in the posterior calyces lying relatively medially and the anterior calyces lying laterally is essential when interpreting contrast imaging of the collecting system of the kidneys.
VASCULAR SUPPLY AND LYMPHATIC DRAINAGE
Renal arteries
The paired renal arteries take about 20% of the cardiac output to supply organs that represent less than one-hundredth of total body weight. They branch laterally from the aorta just below the origin of the superior mesenteric artery (see Fig. 62.8; Fig. 74.10A). Both cross the corresponding crus of the diaphragm at right angles to the aorta. The right renal artery is longer and often higher, passing posterior to the inferior vena cava, right renal vein, head of the pancreas, and descending part of the duodenum. The left renal artery is a little lower and passes behind the left renal vein, the body of the pancreas, and splenic vein. It may be crossed anteriorly by the inferior mesenteric vein.
A single renal artery to each kidney is present in approximately 70% of individuals (Fig. 74.11). The arteries vary in their level of origin and in their calibre, obliquity and precise relations (Fig. 74.10B). In its extrarenal course each renal artery gives off one or more inferior suprarenal arteries, a branch to the ureter and branches which supply perinephric tissue, the renal capsule, and the pelvis. Near the renal hilum, each artery divides into an anterior and a posterior division, and these divide into segmental arteries supplying the renal vascular segments. Accessory renal arteries are common (30% of individuals), and usually arise from the aorta above or below (most commonly below) the main renal artery and follow it to the renal hilum. They are regarded as persistent embryonic lateral splanchnic arteries. Accessory vessels to the inferior pole cross anterior to the ureter and may, by obstructing the ureter, cause hydronephrosis. Rarely, accessory renal arteries arise from the coeliac or superior mesenteric arteries near the aortic bifurcation or from the common iliac arteries.
The subdivisions of the renal arteries are described sequentially as segmental, lobar, interlobar, arcuate and interlobular arteries and afferent and efferent glomerular arterioles (see Fig. 74.14).
Segmental arteries
Renal vascular segmentation was originally recognized by John Hunter in 1794, but the first detailed account of the primary pattern was produced in the 1950s from casts and radiographs of injected kidneys. Five arterial segments have been identified (Fig. 74.12). The apical segment occupies the anteromedial region of the superior pole. The superior (anterior) segment includes the rest of the superior pole and the central anterosuperior region. The inferior segment encompasses the whole lower pole. The middle (anterior) segment lies between the anterior and inferior segments. The posterior segment includes the whole posterior region between the apical and inferior segments. This is the pattern most commonly seen, and although there can be considerable variation it is the pattern that clinicians most frequently encounter when performing partial nephrectomy. Whatever pattern is present, it must be emphasized that vascular segments are supplied by virtual end arteries. In contrast, larger intrarenal veins have no segmental organization and anastomose freely.
Brödel (1911) described a relatively avascular longitudinal zone (the ‘bloodless’ line of Brödel) along the convex renal border, which was proposed as the most suitable site for surgical incision. However, many vessels cross this zone, and it is far from ‘bloodless’: planned radial or intersegmental incisions are preferable. Knowledge of the vascular anatomy of the kidney is important when undertaking partial nephrectomy for renal cell cancers. In this surgery the branches of the renal artery are defined so that the surgeon may safely excise the renal substance containing the tumour while not compromising the vascular supply to the remaining renal tissue.