42 Key Issues in Pediatric Neurointensive Care
In this chapter we outline the epidemiology, presentation, course, and management of key disorders in pediatric neurointensive care. Critically ill infants and children with a compromised central nervous system (CNS) are complex patients and are often highly vulnerable to secondary brain injury. Minimizing physiologic derangements and optimizing therapy are essential from the scene through the pediatric intensive care unit (ICU). In most cases, transport to a specialized pediatric facility is desirable. Trained specialists in pediatric critical care medicine, pediatric neurologic surgery, and child neurology should deliver the ICU care to these infants and children, with appropriate pediatric ancillary support. The information provided in this chapter is germane to practitioners involved in stabilization, emergency treatment, and transport, and to pediatric subspecialists at the tertiary care centers. Recommendations in the areas of pediatric trauma (head and spinal cord injury), procedures, and monitoring are addressed in Chapters 30, W24 (Pediatric Intensive Care Procedures), and 210. Neurointensive care issues relevant to the field of neonatology are outside the scope of this chapter; specialized textbooks and/or reviews in this area should be sought for information in that field.
Issues Unique to Pediatrics
Central nervous system Insults in Infants and Children
Unlike in adults, atherosclerotic vascular disease resulting in stroke, intracerebral hemorrhage, and cardiopulmonary arrest plays little role in pediatric neurointensive care. For example, cardiopulmonary arrest in infants and children results primarily from asphyxia rather than myocardial infarction. Similarly, traumatic brain injury (TBI) in infants younger than 2 years of age is largely the result of abusive head trauma (shaken baby syndrome, child abuse). Unique issues in victims of child abuse, such as chronic injury or delay in presentation, contribute to important differences in diagnosis, treatment, and outcome. The specific CNS insults relevant to pediatric neurointensive care include TBI and spinal cord injury, cardiopulmonary arrest, status epilepticus, stroke, critical CNS infections, postoperative neurosurgical conditions, and several other less common disorders; traumatic brain and spinal cord injury are addressed in Chapters 38 and 39.
Age-Related Differences in the Response to Central nervous system Insults
Brain Water and Blood-Brain Barrier
Many biochemical, physiologic, and physical factors exhibit large fluctuations during brain development. Although the magnitude of these changes are most dramatic during prenatal development, they may contribute to age-related differences in response to critical CNS disorders.1,2 Large decreases in brain water content occur during postnatal development into adult life.3–5 These changes are global and correlate with the amount of myelination. The impact of these changes on edema formation after brain injury is unclear; however, the rapid and diffuse cerebral swelling phenomenon described in many CNS insults in infants and children may be related to this high water content in the immature brain. This is suggested by studies showing that parenchymal injection of glutamate into the immature (but not adult) rat brain rapidly produces a large area of edema.6 The rapidity of development and the great magnitude of edema may result in part from rapid diffusion of glutamate and other mediators through the immature brain. In contrast to the changes in brain water during development, there is little evidence to support similar changes in blood-brain barrier permeability.7,8 However, studies in experimental models suggest that the immature blood-brain barrier is highly vulnerable to injury.9–11 Blood-brain barrier permeability after CNS insults has received little study in pediatric patients.
Cerebral Blood Flow and Energy Metabolism
Postnatal changes in cerebral blood flow (CBF) and energy metabolism have been reported in numerous mammalian species including humans.12–19 In all cases, CBF is quite low both before birth and during infancy, rapidly increases to a peak during childhood, and then decreases to a plateau with a gradual decline with increasing age during adulthood. In a study of 42 normal infants and children, cortical CBF in newborns was between 30 and 45 mL/100 g/min—lower than that reported in adults. In contrast, cortical flow in children between the ages of 5 and 6 years was between 50% and 85% higher than in adults. CBF decreased to adult values by about age 15 years (Figure 42-1).20,21 Increased CBF in children (versus either adults or infants) corresponds to the period of maximal postnatal “brain growth,” specifically, maximal increases in the number of synapses.22–24 Similarly, cerebral metabolic rate for glucose is maximal in children between the ages of 3 and 9 years.17 The impact of these factors in CNS injury is poorly understood. Hyperemia after injury has been implicated as an important facet of the pathophysiology of pediatric CNS injury. Because the level of CBF in the normal child is greater than in adults, the frequency of hyperemia in children is probably lower than has been suggested. Hyperemia in most gray matter structures in children between the ages of 3 and 10 years should probably be based on a flow value greater than about 70 mL/100 g/min19–2124 rather than the value of about 45 mL/100 g/min suggested for adults.25 Alterations in metabolic demands after injury must also be considered.
Cerebral Perfusion Pressure
Cerebral perfusion pressure (CPP; mean arterial blood pressure–intracranial pressure [ICP]) is a critical determinant of CBF outside the limits of autoregulation or when autoregulation of blood pressure (BP) is disturbed. In adults, the normal range for CPP is generally accepted to be between 60 and 150 mm Hg.26,27 Based on studies in normal immature animals, the lower limit for BP autoregulation of CBF is directly related to age.28–30 This is anticipated, since CPP is a function of arterial blood pressure, which is dependent on age. Unfortunately, few data are available on normal values for CPP in infants and children. A mean value of 37.5 ± 4.9 mm Hg (±SD) was reported in normal preterm infants.31 The lower limit of BP autoregulation was not determined. There are also limited data available on the lower limit of BP autoregulation of CBF in brain-injured infants and children. A study carried out in 1983 in 17 infants and children with meningitis and encephalitis showed a critical threshold for CPP of about 30 mm Hg.32 However, survival, not CBF, was the outcome variable in that study. Muizelaar and coworkers20,21 and Sharples and colleagues33 examined CBF autoregulation after TBI in children; however, values for BP autoregulation of CBF for infants and children were not determined. Recently, Vavilala et al.34 studied CBF autoregulation in 53 healthy infants and children. Surprisingly, the lower limit of autoregulation was between 50 and 60 mm Hg across the age groups of younger than 2 years, 2 to 5 years, 6 to 9 years, and 10 to 14 years. This important study suggests that there is substantially less autoregulatory reserve (the difference between baseline MAP and the lower limit of autoregulation) in infants and young children than in older children or adults. It would suggest that modest BP reductions in infants with severe TBI could compromise CBF. This may help explain the important deleterious effects of hypotension as a side effect in recent RCTs in pediatric TBI.35 It also suggests that the lower limit for CPP in brain injured infants and young children of ~50 mm Hg might be wise. Two recent studies also suggest that the presence of mild hypertension after severe TBI is associated with improved outcome in infants and children.36,37 However, the impact of inducing mild hypertension in this setting on outcome remains to be studied.
Myelination
In humans, considerable myelination occurs during postnatal life.23 The impact of this process on the age-related response in pediatric CNS injury is not known but has been suggested by many to contribute to enhanced plasticity in the pediatric brain.
Excitotoxicity
Increases in brain interstitial concentrations of excitatory amino acids such as glutamate are part of a fundamental response to CNS insults across all ages.38–47 Excitotoxicity-mediated damage after brain injury has been reported in laboratory models in mature and immature animals and is suggested in clinical reports in children.39,43–47 There are, however, important age-dependent facets of excitotoxicity. At several periods in development, large numbers of excitatory amino acid receptors are produced, and these periods correlate temporally with increased synaptic plasticity.40–43 Experimental data strongly suggest that the immature brain is at great risk for excitotoxicity.39–41 In hypoxia-ischemia models, studies in immature animals (particularly those modeling the newborn) suggest that glutamate receptor antagonists such as MK-801 are potent neuroprotectants.40,41,43 The results of clinical trials in adults of agents targeting this receptor may not predict their effectiveness in infants or children with critical CNS insults. Further study in children is warranted.
Apoptosis
Experimental models and human data have made it increasingly clear that cells dying after CNS insults can be categorized on a morphologic continuum from necrosis to apoptosis.48–50 The event involved in the cascades of neuronal death after CNS insults is discussed in detail in Chapter 29. The importance of balanced apoptosis (or programmed cell death) in embryogenesis and recent reports examining apoptosis in experimental TBI suggest that there may be important age-related differences in the cell death cascades in response to traumatic or ischemic brain injury.51 For example, neurons in developing animals appear to be more vulnerable to apoptosis than in mature animals.48,51 There are also data supporting the concept that physiologic levels of excitatory amino acids are necessary for neuronal survival in the developing brain.52 The implications on these data in experimental animals must be assessed with caution, but they raise concern about the ability of therapies such as barbiturates or inhibitors of excitatory amino acid receptors to actually induce neuronal death during development. The fetal alcohol syndrome is the prototypical condition cited in this regard.53 What remains unclear, however, is if this enhanced apoptotic response to CNS injury is limited to prenatal development or if it is important during treatment of infants and children in the pediatric ICU. Nevertheless, an important role for apoptosis in pediatric brain injury is suggested by the fact that analysis of cerebrospinal fluid (CSF) in infants and children with severe TBI has provided some of the most compelling molecular data for the participation of these pathways in humans.2 These data include participation of death effectors such as cytochrome-c and Fas receptor/ligand interactions and failure of antiapoptotic pathways in infants and children with poor outcome after severe brain injury.2,54–57 How these findings will influence our therapies remains to be determined, but they suggest that apoptotic neuronal death may represent a particularly important therapeutic target in pediatric neurointensive care.
Extracerebral Factors
Hypotension and hypoxemia are the two most important secondary insults in patients with critical CNS disorders. Hypotension is the most important extracerebral factor associated with poor outcome after severe TBI.58 This may contribute to the high mortality rate (62%) in this condition in children younger than age 4 years.59 Nearly 50% of these children present with shock, versus only 30% of adults.59 The limited blood volume of infants and young children make relatively small amounts of blood loss from scalp lacerations or other foci important. In contrast, the immature brain and cardiovascular systems are resistant to hypoxic-ischemic insults compared with mature individuals.60 The duration of asphyxia resulting in cardiac arrest is inversely related to age.61–64 Resistance to asphyxia-induced cardiac arrest in the immature individual, however, could have complex effects. For example, children may survive protracted episodes of hypoxemia and hypotension that would be lethal in adults. Resistance of the immature myocardium to asphyxia does not preclude the development of cerebral damage from hypoxemia, because between 25% and 56% of children who suffer asphyxia without cardiac arrest have poor neurologic outcome.65 This might also explain some of the severe pathology seen in infants after abusive head trauma, in which apnea, seizures, and agonal states occur.66 Recently Ichord et al.67 showed that a hypoxic-ischemic injury pattern was commonly seen on diffusion-weighted magnetic resonance imaging (MRI) in victims of abusive head trauma. Similarly, Berger et al.68 showed that the serum biomarker profile of neuron-specific enolase in infants with abusive head trauma was more similar to that seen in children with asphyxia than TBI.
Unlike adults, atherosclerotic vascular changes are largely absent in children. This influences pathophysiology. Although normal aging produces a gradual decline in CBF, this decline is accentuated in adults by the presence of risk factors for stroke (e.g., diabetes, cigarette smoking, hypertension), which enhance incipient cerebrovascular disease.69 Atherosclerosis also limits the ability of cerebral circulation to respond to a metabolic challenge.70–73 Some adults may even have maximally dilated cerebral vessels in the resting state. The potential of these factors to unfavorably affect outcome in adults (versus children) is obvious. Ethanol consumption is associated with severe TBI in adults, with as high as 50% of patients having positive blood alcohol levels.74–77 Chronic and acute alcohol consumption can have either detrimental or beneficial effects on brain injury.77 Ethanol use or intoxication is uncommon in pediatric TBI, particularly in infants and young children.
Specific Diseases or Conditions
Cardiopulmonary Arrest
Cardiopulmonary arrest in adults is addressed in detail in Chapter 33. Although some of that chapter is germane to pediatric patients, the importance of asphyxia as the etiology in children mandates a separate discussion.
Epidemiology
The causes of cardiopulmonary arrest in childhood are heterogeneous. Causes of arrest in the prehospital setting include trauma, sudden infant death syndrome, poisoning, and respiratory distress secondary to drowning, choking, severe asthma, or pneumonia.78 Traumatic arrest secondary to exsanguination, massive head injury, or airway compromise is the leading cause of death in childhood and young adulthood. Nontraumatic arrest typically occurs as a consequence of hypoxemia and hypercarbia, leading to respiratory arrest, bradycardia, and ultimately asystole or pulseless electrical activity.78–80 Ventricular tachycardia or fibrillation occurs less commonly in children than adults, but it is not rare; 5% to 15% of children with prehospital arrest have these rhythms.81–83 The majority of arrests in the prehospital setting occur in previously healthy patients, whereas most in-hospital arrests occur in children with preexisting medical conditions.84 Children with special healthcare needs are especially vulnerable to acute deterioration.
Outcome
The rate of survival from pediatric cardiopulmonary arrest is about 13%, with survival from in-hospital arrest greater than that from prehospital arrest (24% versus 9%).80 Asystolic patients have the lowest rate of survival (~5%), whereas patients with ventricular fibrillation or ventricular tachycardia have higher rates of survival (~30%). Patients presenting with isolated respiratory arrest have the highest rate of survival (~75%).85,86 Witnessed arrest and bystander cardiopulmonary resuscitation (CPR) are associated with survival, whereas CPR of greater than 30 minutes and administration of more than two doses of epinephrine are associated with poor outcome.78,81,87,88 About 60% of survivors will have good neurologic outcome, with the remainder showing severe disabilities. Intermediate outcomes are uncommon. Reported mortality rates for children remaining comatose after brain injury range between 34% and 73% dependent on whether TBI is included.89–94 Accurate prediction of poor outcome in this group can enable withdrawal of support and decrease the possibility of “rescuing” children to survival in a neurologically devastated state.95,96 Predictors of poor outcome in children include remaining comatose at 24 hours, a Glasgow Coma Scale (GCS) score of less than 5, absence of spontaneous respirations, absence of pupillary reflex, and specific abnormalities found on electroencephalography (EEG) or after testing of somatosensory evoked potentials. Predictors of poor outcome should be applied with caution to children suffering cardiopulmonary arrest caused by drug overdose or hypothermic exposure (ice-cold water drowning) in which good outcomes have been reported in some cases after even prolonged durations of arrest.
Treatment
If cardiopulmonary arrest occurs, the most important first step is to provide immediate CPR. Many infants and children, especially in the prehospital setting, will be rescued solely by the administration of CPR.78 Important differences are emerging in resuscitation of adults versus children with cardiac arrest. Although there has been a general movement toward bystander compression-only CPR in adults, recently Kitamura et al.97 compared conventional versus compression-only CPR in over 5000 children in Japan. In arrests of noncardiac origin, both survival and favorable neurologic outcomes were better in children given conventional CPR. In addition, outcomes were similar in the setting of arrests of cardiac origin. This study strongly suggests that the lay public should be taught conventional CPR for all children who suffer cardiac arrest. In addition the technique for compressions in children is different than in adults. Only one hand is used to deliver chest compressions to children younger than age 8 years. Two methods are approved for delivering chest compressions to infants. When two or more rescuers are available, one rescuer provides chest compressions by encircling the chest with two hands and depressing the sternum with both thumbs while the other rescuer provides ventilation (Figure 42-2). When only one rescuer is present, two fingers from one hand are used to provide chest compressions and the other hand is used to maintain the head-tilt. Providing adequate ventilation is especially important for children, because most pediatric arrests are secondary to airway compromise. In contrast, adults frequently suffer from cardiac causes of arrest and require intensified efforts at providing chest compressions and early defibrillation. Thus, the recommended ratio of chest compressions to ventilations for young children is 5 : 1, compared with a ratio of 15 : 2 for older children and adults. Once the patient is intubated, ventilations should be asynchronous. Although ventricular fibrillation and ventricular tachycardia are uncommon in children, survival with this rhythm is high (about 30%), so cardiac rhythm should be ascertained as early as possible.80 Automated external defibrillators that can deliver a 50-J dose are now available and are appropriate for use in children aged 1 to 8 years.98
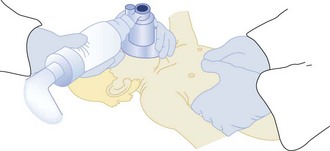
Figure 42-2 Two-person technique for cardiopulmonary resuscitation in infants and young children.
(Reprinted from Pediatric Basic Life Support. Guidelines 2000 for Cardiopulmonary and Emergency Cardiovascular Care: International Consensus on Science. Circulation 2000;102(Suppl):I253–90.)
Intubation of pediatric patients is a difficult task for inexperienced providers. Furthermore, the short length of the trachea combined with patient movement during transport and patient care can easily result in displacement of the endotracheal tube.99 Secondary confirmation of tracheal tube placement is critical. End-tidal CO2 detection is the method most commonly utilized for secondary confirmation of endotracheal tube placement in children. However, a false-negative reading can occur when circulatory collapse is so severe that CO2 is not delivered to the alveolar space. If CO2 is not detected during CPR, tube placement can be confirmed by visualizing the airway with a laryngoscope. Although no single confirmation technique is 100% reliable in all circumstances, some effort of secondary confirmation of tube placement can be helpful.
Patients are initially resuscitated using 100% oxygen. The rationale is that hypoxia often causes or contributes to the development of cardiac arrest, and an oxygen debt accumulates during cardiac arrest. However, there is increasing awareness that oxygen might contribute to reperfusion injury, and thus prolonged delivery of unnecessarily high concentrations of oxygen should be avoided.100,101
Adults resuscitated from cardiac arrest demonstrate intact cerebrovascular reactivity with evidence of hyperventilation-associated ischemia.102 Although there is evidence that injured brain has diminished metabolism, which may offset the decrease in blood flow, it seems prudent to avoid decreasing CBF to injured brain. Therefore, hyperventilation should be reserved for patients with signs of cerebral herniation syndrome or suspected pulmonary hypertension. In addition to avoiding purposeful hyperventilation, it is prudent to guard against inadvertent hyperventilation during patient transport.103 Increased use of quantitative continuous CO2 monitors throughout the health care system would decrease the occurrence of inadvertent hyperventilation.
The most commonly used drugs in pediatric resuscitation are epinephrine, atropine, and sodium bicarbonate (Table 42-1). Magnesium and calcium are reserved for specific indications such as torsades de pointes, hypocalcemia, and calcium channel blockade. Amiodarone has recently been added to the American Heart Association (AHA) pediatric algorithms, based on extrapolation from adult experience.104 Adults with ventricular fibrillation or ventricular tachycardia in the prehospital setting are more likely to be successfully defibrillated after IV administration of amiodarone compared with lidocaine.105 Accordingly, amiodarone (5 mg/kg bolus) is a therapeutic option for children with pulseless arrest. Amiodarone (5 mg/kg infused over 20 to 60 min) is also an option for ventricular tachycardia with a pulse but should be used with extreme caution because of the risk for profound hypotension. Vasopressin has been added to the AHA adult algorithms as an alternative to epinephrine on the basis of its improved myocardial and CBF effects. However, subsequent clinical data in adults have not consistently yielded positive results, and pediatric data are limited to small case series.106,107 The optimal vasopressor for hemodynamic support after return of circulation in children is not known.
Extracorporeal membrane oxygenation (ECMO) has been used to successfully resuscitate children from selected causes of in-hospital cardiac arrest.108–112 ECMO-CPR provides greater cerebral and myocardial blood flow than either closed- or open-chest CPR and facilitates titration of temperature, blood flow, and oxygen-carrying capacity. Good outcomes have been documented with the use of ECMO even when initiated after durations of conventional CPR typically associated with poor outcome. It is best reserved for patients with reversible conditions or as a bridge to cardiac transplantation.
Post-Resuscitative Care
Temperature control is a priority for patients who remain comatose after cardiac arrest. Adults cooled to 32°C to 34°C for 12 to 24 hours after resuscitation from ventricular fibrillation demonstrate improved survival and neurologic outcome.113,114 In contrast, fever worsens outcome in experimental models of brain injury and has been associated with worse clinical outcome in adults with ischemic brain injury. Children resuscitated from cardiac arrest often develop mild hypothermia followed by delayed fever.115 There is a consensus that initial hypothermia, if tolerated, should be permitted to continue and fever should be vigilantly avoided. The practice of inducing hypothermia in normothermic children is more controversial. Experimental models using either pediatric mechanisms of injury (asphyxia, hypovolemic shock) or examining the immature brain suggest a beneficial effect of induced hypothermia. However, clinical data are limited and there is a concern about hypothermia-impaired immune function and risk of pneumonia/sepsis.116,117 Clinical trials of induced hypothermia for neonatal asphyxia have been remarkably positive,118–120 and important data in newborns with asphyxia indicate that even one degree of hyperthermia after the insult is associated with neurologic morbidity.121 This supports the need for targeted temperature management after cardiac arrest.
Sustained elevation of ICP may be more common after asphyxial arrests versus arrests of cardiac origin122 and is a poor prognostic sign in children with drowning. ICP monitoring fell out of favor in the 1980s when it was found to not influence outcome in small case series.123 However, studies using contemporary ICP-directed therapy (perhaps including induced hypothermia) deserve reevaluation.
Miscellaneous
Most pediatric victims of cardiopulmonary arrest will not be successfully resuscitated. The difficulty of accepting this reality often results in prolonged attempts at resuscitation. The AHA guidelines state, “In the absence of recurring or refractory ventricular fibrillation or ventricular tachycardia, history of a toxic drug exposure, or a primary hypothermic insult, resuscitative efforts may be discontinued if there is no return of spontaneous circulation despite advanced life support. In general, this requires no more than 30 minutes.”104 This acknowledges the futility of prolonged resuscitative efforts and empowers clinicians to feel permitted to stop resuscitative efforts. The guideline does not mandate stopping at a specific duration of CPR, but clinicians should recognize that the chance of survival with lifelong severe disabilities correlates with the duration of CPR.
Surveys indicate that most family members would like to be present during resuscitation attempts of a loved one124–127; presence during resuscitation can help family members adjust to the death.128,129 Although allowing family presence during resuscitation requires planning and additional resources, when done properly it is worth the effort. Perhaps one of the most disheartening statistics in resuscitation research is the high divorce rate (up to 90%) of parents after the death of a child. Thus, pastoral and social services can be integral components of care during both the acute resuscitation event and long-term follow-up.
Status Epilepticus
Status epilepticus is a pediatric emergency traditionally defined as either a continuous seizure of at least 30 minutes or more than two discrete seizures without complete recovery of consciousness. Refractory status epilepticus is defined as failure of two first-line antiepileptic medications to treat this condition for greater than 60 minutes. Many children with refractory status epilepticus have new or established CNS lesions.130
Epidemiology and Etiology
The incidence of pediatric status epilepticus from a prospective study is 40 cases/100,000 per year. Infants younger than 1 year of age have the highest incidence at 150 cases/100,000 per year.131 More than 90% of cases are convulsive status epilepticus. The first episode of status epilepticus occurs at a mean age of 4.2 years.132 There is a slight male predominance in status epilepticus.131,133
There are five etiologic categories of status epilepticus that have bearing on treatment and prognosis. A child with idiopathic or cryptogenic status epilepticus has no prior history of seizures and no known risk factors. Atypical febrile status epilepticus occurs during fever in children with no prior history of seizures without fever. Children with acute symptomatic status epilepticus have new CNS lesions such as encephalitis, trauma, tumor, stroke, or anoxia. Children with remote symptomatic status epilepticus have preexisting CNS lesions and therefore a lowered seizure threshold. In these children, status epilepticus can occur without provocation, sometimes even years after the initial insult. Finally some children have status epilepticus resulting from progressive encephalopathy, including neurodegenerative diseases, malignancies, and neurocutaneous syndromes (Box 42-1).131,133,134
In one study, status epilepticus accounted for 1.6% of total pediatric ICU admissions, and etiology varied with age. In children younger than 2 years of age, acute symptomatic status epilepticus from meningitis and encephalitis accounted for 51% of cases, whereas remote symptomatic status epilepticus in children with a prior diagnosis of epilepsy was seen in 16% of children. Older children were more likely than younger children to have a history of epilepsy.133 Mortality rates for status epilepticus in children are between 3% and 6%.131,134 Mortality is dependent on etiology, age, and duration of status epilepticus. Mortality rates of 0% and 12.5% were seen when patients were divided into either unprovoked/febrile status epilepticus or acute CNS insult/progressive encephalopathy groups, respectively.133 Morbidity risk varies from between 11% and 25%. Infants are at great risk for morbidity because the etiology in this group is commonly acute symptomatic status epilepticus. Neurologic sequelae of status epilepticus include epilepsy, recurrence, mental retardation, and motor disorders. However, many of the morbidities can be attributed to the underlying disease and not status epilepticus per se. Risk of recurrence in the category of idiopathic status epilepticus is less than 5%. In contrast, recurrence of status epilepticus in children in the acute symptomatic groups can be as high as 60%.131,135 Systemic complications occur with increasing frequency in proportion to the duration of status epilepticus, the most important being respiratory failure and cardiovascular compromise and autonomic and metabolic disturbances.136
Diagnosis
Status epilepticus can be convulsive or nonconvulsive when comparing clinical events with electrographic information. Convulsive seizures either begin as generalized seizures or progress from partial seizures. Nonconvulsive seizures are characterized as having subtle clinical signs such as nystagmus, irregular clonic twitches along with decreased consciousness, and/or ictal discharges on EEG. Included under the subheading of nonconvulsive seizures are complex and simple partial and absence seizures.137
Treatment
The goals in treating status epilepticus are to provide respiratory and cardiovascular support, terminate clinical and electrical seizure activity, identify and treat precipitating factors, and prevent systemic complications.137 Recognizing that a prolonged duration of seizure increases the risk of morbidity and mortality, the Epilepsy Foundation of America published a consensus view to initiate antiepileptic drugs for treatment 10 minutes after the onset of an episode of status epilepticus.138 A timetable for treatment of status epilepticus in children is provided in Table 42-2.
TABLE42-2 Suggested Timetable for Emergency Diagnosis and Treatment of Status Epilepticus
Time | Exam/Intervention | Testing |
---|---|---|
Initial presentation: 0 min | Airway, breathing, circulation, IV access, monitoring | Glucose, oxygenation via pulse oximetry ± blood gas analysis |
Primary survey: 5 min | Neurologic exam Administer antiepileptic drugs Lorazepam, 0.1 mg/kg IV Phenobarbital, 20 mg/kg IV Normal saline maintenance IV Reduce fever |
Electrolytes, renal and liver function, ammonia, anticonvulsant levels, toxicology, complete blood cell count, urinalysis |
Secondary survey: 15-30 min | Evaluate treatment results Second-line antiepileptic drug if seizure persists Fosphenytoin, 20 mg/kg IV; or phenytoin, 20 mg/kg IV |
Patient-specific: cranial imaging (CT vs MRI), lumbar puncture, EEG, ECG |
Status epilepticus: >30 min | Intubation and mechanical ventilation | |
Refractory status epilepticus: >60 min | Titrate antiepileptic drug to burst suppression Pentobarbital, 10 mg/kg IV given over 30 min, then 5 mg/kg every hour for 3 doses, then 1 mg/kg/h; titrate to effect Midazolam, 0.15 mg/kg IV, then 1-2 µg/kg/min, titrate to effect Phenobarbital, 5-10 mg/kg IV every 20 minutes to achieve burst suppression, then every 12 hours Evaluate need for vasopressors |
Continuous EEG Neurologic consultation Consider anesthesia consultation for treatment with inhaled anesthetic |
CT, computed tomography; ECG, electrocardiogram; EEG, electroencephalogram; IV, intravenous; MRI, magnetic resonance imaging.
Phenytoin/Fosphenytoin
In a study in adults comparing lorazepam, phenytoin, phenobarbital, and diazepam, phenytoin had the highest success rate in stopping status epilepticus.139 Phenytoin is not commonly associated with respiratory depression and has less of an effect on the impairment of consciousness than either benzodiazepines or barbiturates. Fosphenytoin has the advantage of having a faster infusion rate, shorter onset of action, and less cardiovascular side effects than phenytoin but is more expensive.
Lorazepam
In the same study in adults, lorazepam had the second highest success rate in stopping status epilepticus.139 Lorazepam can be administered rapidly, has a long duration of effect, and is effective even when administered rectally. Lorazepam produced less respiratory failure requiring intubation than diazepam in retrospective140 and prospective studies.141 Incidence of respiratory depression in these studies varied widely—between 3% and 76%. Support for selection of Lorazepam over diazepam was also shown in a recent Cochrane review.142
Additional Diagnostic Workup
Lumbar puncture is best performed early after presentation, but not in unstable patients or those who may have increased ICP. The decision to perform lumbar puncture should be guided by head CT. Otherwise, the type of neuroimaging used in infants and children with status epilepticus should be individualized, depending on history and physical findings. Both electrocardiography (ECG) and EEG are useful to investigate cause of status epilepticus (i.e., long QT syndrome or identifiable EEG patterns). EEG is also useful in titrating therapy (see later discussion).137
Drug Treatment for Refractory Status Epilepticus
Initiation of treatment for refractory status epilepticus should occur by 60 minutes, usually with neurologic consultation and with appropriate monitoring in a pediatric ICU or intermediate unit. These patients are mechanically ventilated, and seizures are typically treated with a variety of therapies, generally to induce burst suppression on continuous EEG. Most commonly, pentobarbital is used as a continuous infusion to treat refractory status epilepticus. Pentobarbital is given initially as a slow IV loading dose of 5 to 15 mg/kg, followed by an infusion rate of 1 mg/kg/h titrated to effect. There are differing opinions on when to begin to wean therapy, but it is generally recommended that about 12 hours of seizure cessation be attained before weaning the infusion.143 In children, placement of either a central venous pressure or pulmonary artery catheter is indicated to titrate fluid, inotropic, and/or pressor support. Pentobarbital use often requires the addition of inotropes or pressors. As an alternative to continuous barbiturate infusion, phenobarbital can be administered every 20 minutes (5–10 mg/kg IV) to achieve burst suppression, and then as a chronic therapy every 12 hours. A midazolam infusion has also been shown to be effective in refractory status epilepticus in some children (0.15 mg/kg IV bolus followed by infusion of 1–2 µg/kg/min). The infusion can be increased every 15 minutes if seizures are still present on continuous EEG or if burst suppression is not achieved. With this approach in one series, inotropic support was not required.144
Stroke
Epidemiology
Stroke in children is becoming increasingly recognized and now exceeds an incidence of 8 cases per 100,000 children per year.145 Substantial advances in our knowledge of this condition in children have resulted from the work of the Canadian Pediatric Ischemic Stroke Registry. Neonates account for about 25% of these cases. The increasing incidence is believed to result from improvements in diagnostic tools (MRI, computed tomography [CT], magnetic resonance angiography [MRA]) applied to the pediatric population and to increasing survival rates in infants and children with stroke risk factors (e.g., complex congenital heart disease, malignancies).
Etiology
As discussed, atherosclerosis is a key risk factor for stroke in adults. In pediatric and neonatal stroke, extracerebral risk factors contribute to about 75% of cases; however, the spectrum of risk factors differs from those seen in adults. DeVeber145 grouped the most common risk factors for childhood ischemic stroke into vascular, intravascular, and embolic categories (Box 42-2). The most common vascular risk factor has been reported to be transient cerebral arteriopathy.146 Post-varicella arteriopathy, migraine, traumatic carotid dissection, and vasculitis, such as moyamoya, are also important examples in this category. In the intravascular category, sickle cell anemia, sinus thrombosis, leukemias, and both acquired and congenital prothrombotic states are important examples. Dehydration and intravascular volume depletion increase stroke risk in these settings, which are of special importance in the pediatric ICU. There is an 84% incidence of an acute systemic illness and a 30% incidence of dehydration in cerebral sinovenous thrombosis in infants and children.147 Congenital and acquired heart disease in infants and children are the most important underlying causes of embolic stroke.145 The risk of stroke in children after surgery for congenital heart disease is about 1 in 250 cases.148
Diagnosis
The clinical presentation of stroke in infants and children is age related. Infants present typically with seizures and lethargy, whereas older children may present with acute focal neurologic deficits or diffuse symptoms (headache, lethargy, or seizures).145,149 In some cases, the duration of neurologic deficits in pediatric stroke may be shorter than the 24-hour deficits classically required to differentiate stroke from transient ischemic attack in adults.150 It is often difficult to differentiate migraine, Todd paralysis, and stroke in children. Complicating this problem, CT may be normal within the initial 12 hours.145 MRI is a more sensitive technique for diagnosing stroke, and advanced MRI modalities such as perfusion, diffusion, and MRA are important adjuncts to making the diagnosis. These methods are discussed in Chapter 31. Because of the impact of making specific vascular diagnoses on the management strategy, angiography is often recommended in children with idiopathic stroke.145
In addition to the importance of echocardiography in the diagnostic work of stroke after cardiac surgery or catheterization, endocarditis, cardiomyopathy, and other occult cardiac abnormalities are also important risk factors for embolic stroke, thus recognizing the importance of echocardiography and the general diagnostic workup for stroke in children.151,152 A general diagnostic approach to pediatric stroke is presented in Box 42-3.
Box 42-3
Diagnostic Workup in Pediatric Stroke
CT, Computed tomography; MRA, magnetic resonance angiography; MRI, magnetic resonance imaging.
Adapted from the Children’s Hemiplegia and Stroke Association. Website: http://www.chasa.org/diagnosis.htm
Treatment
In the acute setting, antithrombotic therapy has been used increasingly in the therapy for pediatric stroke. Strater and colleagues153 compared treatment with low-molecular-weight heparin versus aspirin in 135 children across a variety of causes (including idiopathic, cardiac, vascular, and infectious) and suggested safety when used to prevent stroke recurrence. This is a controversial area for which there is a lack of systematic study.154 DeVeber145 recommends that neonates do not require antithrombotic treatment because of negligible recurrence risk, whereas older children require aspirin (2–3 mg/kg/d).155 In dissection, high-grade stenosis, or severe prothrombotic state, low-molecular-weight heparin or warfarin (Coumadin) is recommended for several months. In endocarditis, anticoagulation is not recommended because of the risk of rupture of occult mycotic aneurysms. Thrombolytic therapy has been subjected to very limited study in children. Cases describing the use of tissue plasminogen activator and cerebral balloon angioplasty in acute stroke in children with dramatic results are being reported.156 Table 42-3 compares key management issues across three recent guidelines documents in acute ischemic stroke, as summarized by DeVeber and Kirkham.157
Supportive Care in the Pediatric Intensive Care Unit (ICU)
An evidence-based approach for care in the pediatric ICU of children with stroke is lacking. Nevertheless, intensive care for the child with stroke must be at a level commensurate with that provided for other critical pediatric neurologic disorders such as severe TBI158 and ruptured arteriovenous malformation.159
Arterial blood pressure must be adequate to optimize cerebral perfusion. The management of systemic hypertension in the setting of pediatric stroke can be complicated by the variety of underlying disorders (i.e., status post cardiac surgery, underlying hypertension) and the presence or absence of hemorrhage. In adults with thrombotic or hemorrhagic stroke and systemic hypertension, it is generally recommended that mean arterial blood pressure not be aggressively reduced below 130 mm Hg.160 Age-appropriate guidelines for this question are not available for children. In the pediatric ICU, for acute stroke, it is a reasonable first approach to extrapolate from the adult recommendations.
In infants and children with severe stroke with infarction and cerebral swelling, signs and symptoms of raised ICP can develop. Standard protocols for monitoring ICP and treatment of raised ICP in stroke in infants and children have not been developed. Nevertheless, intracranial hypertension can develop; and even in the absence of controlled trials on the beneficial effects of ICP-directed therapy in severe pediatric stroke, ICP monitoring and ICP-directed therapy should be considered if signs and symptoms of intracranial hypertension develop. Anecdotal reports of successful treatment with a variety of therapies including mild hypothermia and decompressive craniectomy have been reported.161,162 Plasticity in the pediatric brain, particularly in the recovery from focal lesions, should prompt the consideration of an aggressive approach.163–165 However, long-term morbidity remains substantial after stroke in childhood.166
Critical Central Nervous System Infections
Bacterial Meningitis
Epidemiology
The etiology of bacterial meningitis and its treatment differ in neonates (0–28 days of life) versus older infants and children. During the first 2 months of life, the bacteria that cause meningitis in normal infants reflect the maternal flora and the environment to which the infant is exposed. The most common pathogens include groups B and D streptococci, gram-negative enteric bacilli, and Listeria monocytogenes. Occasionally, Haemophilus influenzae (both type B and nonencapsulated strains) and other pathogens—more typically found in older patients—can be the etiologic agent. Bacterial meningitis in children between 2 months and 12 years of age is usually caused by Streptococcus pneumoniae, Neisseria meningitides, or H. influenzae type B. After the implementation of immunization against H. influenzae, the incidence of H. influenzae meningitis decreased rapidly. Subsequent to the universal recommendation for the use of conjugated pneumococcal vaccine at 2 months of age in 2000, the incidence of meningitis caused by this pathogen is also decreasing. Anatomic abnormalities, surgical procedures, neurotrauma, or immune deficiency often underlie meningitis caused by other agents.167
Bacterial meningitis most commonly results from hematogenous dissemination of microorganisms from a distant site of infection; bacteremia usually precedes meningitis or occurs concomitantly. Colonization of the nasopharynx with a pathogenic microorganism is the usual source of bacteremia. Bacteria gain entry to the CSF through the choroid plexus of the lateral ventricles and the meninges and then circulate to the extracerebral CSF and the subarachnoid space. Bacterial cell wall lipopolysaccharide of gram-negative bacteria and pneumococcal cell wall components stimulate a marked inflammatory response, with local production of tumor necrosis factor alpha, interleukin-1β, prostaglandin E, and other mediators, leading to neutrophil infiltration, increased vascular permeability, and thrombosis. Inflammation of spinal nerves and roots produces meningeal signs, and inflammation of the cranial nerves produces optic, oculomotor, facial, and auditory neuropathies. Intracranial hypertension can produce oculomotor and abducens nerve palsy. Intracranial hypertension in meningitis is believed to result from a combination of cell death (cytotoxic cerebral edema), cytokine-induced increased capillary vascular permeability (vasogenic edema), and increased hydrostatic pressure after obstruction of CSF reabsorption and/or flow. Rarely, meningitis may follow bacterial invasion from a contiguous focus of infection such as paranasal sinusitis, otitis media, mastoiditis, orbital cellulites, or cranial or vertebral osteomyelitis or may occur after introduction of bacteria via penetrating head trauma or meningomyelocele.168
Diagnosis
The clinical presentation may be as fulminant as rapidly progressing shock, purpura, disseminated intravascular coagulation, and altered consciousness, frequently resulting in death within 24 hours. More often, however, children present with several days of fever with upper respiratory tract or gastrointestinal symptoms, followed by nonspecific signs of CNS infection such as lethargy and irritability. The presence of headache, emesis, bulging fontanelle, widening of the sutures, oculomotor or abducens nerve paralysis, hypertension with bradycardia, apnea, or hyperventilation suggests intracranial hypertension. Papilledema is uncommon in uncomplicated meningitis and suggests a more chronic process, such as intracranial abscess, sinus thrombosis, or subdural empyema. Seizures can result from cerebritis, infarction, or electrolyte abnormalities and occur in between 20% and 30% of children with meningitis. Seizures that occur at presentation or within first 4 days of onset are usually of no prognostic significance. Seizures that persist beyond the fourth day of illness and those that are difficult to treat are associated with poor prognosis.169
The diagnosis of acute bacterial meningitis is confirmed by analysis of CSF. Contraindications for an immediate lumbar puncture are (1) evidence of increased ICP (other than bulging fontanelle), (2) presence of severe cardiopulmonary compromise or likelihood that positioning for the procedure would significantly compromise cardiopulmonary function, (3) infection of the skin overlying the needle insertion site, and (4) coagulopathy. If lumbar puncture is delayed, empirical antibiotic treatment should be started after a blood culture is obtained. Blood culture reveals the susceptible bacteria in 80% to 90% of cases of meningitis. The need for a cranial CT scan, for signs and symptoms of increased ICP or brain abscess, should not delay therapy. Table 42-4 summarizes the CSF findings in CNS infections. Pleocytosis with lymphocyte predominance may be seen early in bacterial meningitis; conversely, neutrophilic pleocytosis may be present in patients during the early stages of acute viral meningitis. The shift to lymphocytic-monocytic predominance in viral meningitis invariably occurs within 8 to 24 hours. A traumatic lumbar puncture complicates the diagnosis of meningitis. If the CSF is bloody, it should be collected in three or more tubes. If the CSF clears in successive tubes, it suggests a traumatic lumbar puncture. Blood that does not clear is more suggestive of intracranial bleeding. The CSF leukocyte to erythrocyte ratio in CSF from a traumatic lumbar puncture is generally similar to that in a concurrently obtained peripheral blood sample (usually 1 : 500 to 1 : 1000).170
The mortality rate of bacterial meningitis after the neonatal period is less than 10%, owing to appropriate recognition, prompt antibiotic treatment, and supportive care. Severe neurodevelopmental sequelae occur in between 10% and 20% of pediatric patients. The most common sequelae include hearing loss, mental retardation, epilepsy, delay in language acquisition, visual impairment, and behavioral problems. Sensorineural hearing loss occurs in 30%, 10%, and 5% to 10% of patients with pneumococcal, meningococcal, and H. influenzae type B meningitis, respectively.171
Treatment
The initial (empirical) choice of antibiotic treatment in immunocompetent infants and children is primarily determined by the antibiotic susceptibilities of S. pneumoniae. In the United States, between 25% and 50% of strains of S. pneumoniae are currently resistant to penicillin, and up to 25% of isolates are resistant to cefotaxime or ceftriaxone. Based on this, empirical therapy is with vancomycin (60 mg/kg/24 h, divided q 6 h) and cefotaxime (200 mg/kg/24 h, divided q 6 h) or ceftriaxone (100 mg/kg/24 h, given either as a single daily dose or divided q 12 h). Patients allergic to beta-lactam antibiotics can be treated with chloramphenicol (100 mg/kg/24 h, divided q 6 h). If L. monocytogenes infection is suspected, as in infants between 1 and 2 months of age or patients with T-lymphocyte deficiency, ampicillin (200 mg/kg/24 h, divided q 6 h) should be administered with either cefotaxime or ceftriaxone. If a patient is immunocompromised and gram-negative bacterial meningitis is suspected, ceftazidime and an aminoglycoside may be used as initial therapy. The duration of treatment should be either 10 or 14 days, depending on the bacteria; gram-negative bacillary meningitis should be treated for 3 weeks or for at least 2 weeks after sterilization of CSF. Repeat lumbar puncture may be indicated in some neonates and in children with gram-negative or beta-lactam–resistant meningitis caused by S. pneumoniae. Of the adjunctive treatments that might limit CNS inflammation, only corticosteroids have been properly assessed in clinical trials. Adjuvant corticosteroid use was associated with lower case fatality and lower rates of both severe hearing loss and long-term neurologic sequelae in acute bacterial meningitis. Corticosteroids administered either before or with the first dose of antibiotic reduced severe hearing loss in bacterial meningitis caused by H. influenzae as well as in meningitis caused by S. pneumoniae.172 The recommended dose of dexamethasone is 0.6 mg/kg/24 h, divided every 6 hours for 4 days.168 A recent meta-analysis of individual patient data from five randomized, double-blind, placebo-controlled trials (two of them including pediatric patients from 2 months to 16 years of age) of dexamethasone published since 2001 did not show benefit of adjunctive dexamethasone on mortality or neurologic disability.172 Therefore the role of dexamethasone in prevention of death or neurologic sequelae needs reevaluation. There are no data about the role of corticosteroids in newborns or in patients with nosocomial or CSF shunt–associated meningitis.
Peltola et al.173 reported improved outcomes in 654 children with meningitis with oral glycerol therapy (6 mL/kg/d divided in 4 doses; maximum 25 mL/dose). However, a more recent study did not demonstrate benefit from oral glycerol on hearing impairment in pediatric meningitis.174 Nevertheless, glycerol was recommended in pediatric meningitis in two recent reviews, albeit not at a guidelines level.175,176 Patients who (1) manifest signs of poor perfusion, cutaneous manifestations of disseminated intravascular coagulation (purpura, petechiae), irregular respiratory pattern, altered mental status, cranial nerve involvement, and other signs potentially indicative of raised ICP and patients who (2) have rapid clinical presentation, significant metabolic acidosis, hypoxemia, hypercapnia, neutropenia, hyponatremia, anemia, and abnormal liver or renal function should be admitted to the pediatric ICU—at least until the course of illness can be determined, the first several doses of antibiotics are administered, and a tentative bacteriologic diagnosis is made. Early recognition of complications such as shock or raised ICP and initiation of treatments in a timely fashion may improve outcome in cases of fulminate meningitis.
Acute CNS complications during the treatment of meningitis include seizures, intracranial hypertension, cranial nerve palsies, stroke, herniation, and thrombosis of the dural venous sinuses.177 Subdural effusions develop in between 10% and 30% of pediatric patients and are more common in infants. They are asymptomatic in between 85% and 90% of cases. Aspiration of subdural effusions is indicated in the presence of raised ICP; fever alone is not an indication for aspiration. The syndrome of inappropriate secretion of antidiuretic hormone (SIADH) with hyponatremia and reduced serum osmolality occurs in between 30% and 50% of children. Cerebral salt wasting can also be seen. Attention to maintaining a normal serum sodium concentration using either normal saline or judicious titration of hypertonic saline is important to preventing exacerbation of brain edema. Prolonged fever (>10 days) occurs in 10% of the patients. It is usually due to intercurrent viral infection, secondary or nosocomial bacterial infection, thrombophlebitis, drug reaction, pericarditis, or arthritis. Thrombocytosis, eosinophilia, or anemia may also develop during treatment.169
Viral Encephalitis
Epidemiology
Enteroviruses are the most common etiologic agent for encephalitis in children. The severity of the disease ranges from mild illness to severe encephalitis with death or long-term morbidity. Enterovirus infections spread directly from person to person, with an incubation period of between 4 and 6 days. Most cases occur in summer and fall in temperate climates. Arboviruses are responsible for some cases of encephalitis in children. The most common arboviruses responsible for CNS infection in the United States are St. Louis and California encephalitis and the West Nile virus.178
Several members of the herpesvirus family can cause encephalitis. Herpes simplex virus (HSV) type 1 is an important cause of severe encephalitis in children and adults. The cerebral cortex, especially the temporal lobe, is often severely affected by HSV. Neonatal herpes infections are usually caused by HSV type 2 contracted at delivery via vertical transmission. Three forms of the disease develop in neonates: (1) skin, eye, mouth disease (seen in 45% of cases), (2) encephalitis (seen in 35% of cases), and (3) disseminated intravascular coagulation (seen in 20% of cases). The transmission rate from mother to infant is between 30% and 40% when genital infection is primary and 3% for reactivated herpes infection. The mean age at onset of cutaneous or systemic disease is 6 days after birth. In contrast, the mean age at onset of encephalitis is 11 days after birth. The diagnosis of HSV infection in neonates can be difficult to make unless skin lesions are present. Cultures of conjunctiva, nasopharynx, and rectum at between 48 hours and 72 hours of age may identify early infection. In neonates, the mortality rates are approximately 50% and 14% for HSV disseminated disease and encephalitis, respectively.178
A number of other viral causes are important in pediatric encephalitis. Varicella-zoster may cause CNS infection in close proximity to chickenpox. The most common manifestation of CNS infection by varicella-zoster is cerebellar ataxia. Cytomegalovirus infection of the CNS may be either part of congenital infection or disseminated disease in an immunocompromised host. CNS diseases caused by Epstein-Barr virus may present as perceptual distortions of sizes, shapes, and spatial relationships known as “Alice in Wonderland syndrome.” There may be meningitis, seizures, ataxia, facial palsy, transverse myelitis, and encephalitis.178 Influenza A (H1N1) has taken on considerable significance during the pandemic and a recent report by Baltagi et al.179 demonstrated important neurologic sequelae of this infection in children including altered mental status, seizures, and encephalopathy. Notably, these findings were seen in children without significant respiratory symptomatology.
Infectious agents can enter the brain via a hematogenous route or by neuronal tracts. Many hematogenous pathogens cause direct endothelial damage to arteries, arterioles, and capillaries, resulting in vasculitis, hemorrhage, and thrombosis. Postinfectious encephalitis is an autoimmune process characterized by a perivenulitis with demyelination. It is uncommon in children younger than 1 year of age.180 The mortality rate in untreated cases of HSV encephalitis is 70%, and fewer than 3% return to normal function. Early treatment with acyclovir reduces the mortality rate to 20% to 30%, but there is still substantial morbidity.181
Diagnosis
The onset of illness is generally acute and often preceded by a nonspecific febrile illness of few days’ duration. The manifestations of viral encephalitis in older children are headache and hyperesthesia, whereas in infants, irritability and lethargy predominate. Adolescents frequently complain of retrobulbar pain. Fever, nausea, vomiting, photophobia, and pain in the legs, back, and neck are common. Exanthems often precede or accompany the CNS signs. Seizures occur in 60% of the cases during the course of HSV encephalitis. The diagnosis of viral encephalitis is usually made on the basis of clinical presentation of nonspecific prodrome followed by progressive CNS symptoms. The CSF usually shows a mild mononuclear predominance. In the diagnostic workup, the CSF should be cultured for viruses, bacteria, fungi, and mycobacteria.182 Detection of viral DNA or RNA by polymerase chain reaction is useful for diagnosis of HSV, varicella-zoster, cytomegalovirus, Epstein-Barr virus, and enteroviral meningoencephalitis. Polymerase chain reaction of CSF is 100% specific and more than 90% sensitive for HSV.183 About 50% of patients with HSV encephalitis have focal abnormalities on nonenhanced CT. MRI is the imaging modality of choice and should ideally be the first step after initial clinical examination. The EEG is abnormal in almost all cases of HSV encephalitis and may show periodic lateralized epileptiform discharges (Figure 42-3).184
Treatment
Antiviral therapy with acyclovir is indicated for HSV encephalitis. Acyclovir has a relatively short half-life in plasma, and more than 80% is excreted unchanged in the urine, so renal impairment can exacerbate toxicity. The standard dose of acyclovir for HSV encephalitis is 30 mg/kg/24 h, divided every 8 hours for 14 days. The dose in neonates is 60 mg/kg/d. The duration of treatment is 21 days for immunocompromised patients. Acyclovir is effective in encephalitis due to HSV types 1 and 2 and varicella-zoster. The dose of acyclovir for varicella-zoster encephalitis is similar to that for herpes simplex encephalitis.182 Antiviral therapy with oseltamivir is indicated in H1N1 encephalopathy.179
Supportive Care in the Pediatric Intensive Care Unit
A substantial body of data supporting an evidence-based approach to care in the pediatric ICU of children with meningitis and encephalitis is lacking. Careful attention to the ABCs with a neurointensive care approach is essential. If the GCS score is less than 8 and/or the airway or ventilation is compromised, intubation is indicated and should be performed using a neuroprotective rapid-sequence approach. Normal values for both PaCO2 and PaO2 should be ensured. Bacterial meningitis and encephalitis can be associated with severe septic shock that should be approached and treated according to published guidelines.185 Arterial blood pressure must be adequate to optimize cerebral perfusion.
In infants and children with meningitis and encephalitis, increased ICP may develop. The most important morbidity and mortality of CNS infections is herniation of brain tissue secondary to intracranial hypertension. No randomized controlled trial has been conducted to evaluate the effect ICP monitoring has on outcome in meningitis or encephalitis in children or adults. However, evidence supports the association of intracranial hypertension and poor neurologic outcome in infants and children.186–188 In addition, ICP monitoring and aggressive treatment of intracranial hypertension showed reductions in the expected mortality rate in pediatric and adult patients with meningitis and encephalitis.189–192 ICP monitoring and ICP-directed therapy should be considered if signs and symptoms of intracranial hypertension develop in children with meningitis and encephalitis. ICP monitoring in patients with known or suspected CNS infection with a GCS score less than 8 may be considered at the discretion of the physician. An external ventricular drain is the preferred route of ICP monitoring if there is hydrocephalus or CSF is required for therapeutic or diagnostic drainage. Likely benefit derived from oral glycerol, as previously discussed, is mediated via an osmolar effect.
Other aspects of contemporary pediatric neurointensive care should be included in the treatment regimen, including maintenance of euglycemia and careful fluid management to maintain both a euvolemic state and avoid hyponatremia. This is particularly important because SIADH is common in these conditions. Appropriate nutritional support as outlined in Chapter 95 should also be instituted as soon as possible.
Brain Abscess
Epidemiology and Diagnosis
Brain abscesses are most common in children between the ages of 4 and 8 years. The underlying causes of brain abscess include chronic otitis media and sinusitis, orbital cellulitis, dental infections, penetrating head injury, infection of ventriculoperitoneal shunts, immunodeficiency states, embolization due to congenital heart disease with left-to-right shunts, and meningitis. About 80% of brain abscesses in children occur in frontotemporal and parietal lobes, and 30% have multiple sites of involvement. Table 42-5 summarizes the relationships between predisposing conditions and site of brain abscess, likely pathogens, and suggested initial empirical treatment. In the early stages, the clinical presentation of brain abscess includes low-grade fever, headache, and lethargy. Vomiting, papilledema, focal neurologic signs, and seizures may develop as the inflammation proceeds. Nystagmus, ipsilateral ataxia and dysmetria, headache, and vomiting are characteristic signs of cerebellar brain abscess. If the abscess ruptures into the ventricular cavity, severe shock may rapidly develop and death may result.193
Treatment
Treatment is initiated with an antibiotic regimen that is based on the probable pathogenesis and most likely organism. An encapsulated abscess should be treated by antibiotics and aspiration, which is also the most likely diagnostic approach. Surgery is indicated when the abscess (1) is larger than 2.5 cm in diameter, (2) contains gas, (3) is multiloculated, (4) is located in the posterior fossa, or (5) when fungus is identified. The duration of treatment depends on the organism and response but usually ranges between 4 and 6 weeks. Other aspects of neurointensive care in the pediatric ICU for infants and children with brain abscess should mirror those presented previously for meningitis and encephalitis.194
Postoperative Neurosurgical Cases
Treatment
Hyponatremia is of particular concern in neurosurgical patients, because the osmotic effects can result in increasing cerebral edema. The incidence is as high as 31% at 48 hours in pediatric surgical patients,195 and the use of isotonic fluid in the pediatric ICU can reduce iatrogenic hyponatremia.196 Thus, normal saline is the preferred IV fluid to avoid this complication. When hyponatremia occurs in conjunction with a decreasing urine output, a high specific gravity, and a high sodium concentration in the urine, it is likely a result of SIADH. In this case, fluid restriction is indicated. Neurosurgical patients also have two unique possible sources for excessive sodium loss: CSF losses from extraventricular drainage and urine losses from cerebral salt wasting. Both require correction of sodium losses.
A few medications should be considered for every neurosurgical patient. First, antiemetics are important to prevent postanesthesia nausea and vomiting, because vomiting can cause a dramatic increase in intracranial pressure. Ondansetron and droperidol are good choices for antiemetic therapy because they are minimally sedating.197 Postoperative seizures can have serious consequences. Antiepileptics should be considered in all patients at risk for postoperative seizures. Typically, phenytoin is the least sedating drug for seizure prophylaxis. Patients on chronic anticonvulsants should have their usual regimen started as soon as possible after the surgery. Dexamethasone is used to reduce edema formation around brain tumors and reduce tumor size.198 The use of corticosteroids is controversial in most other settings. However, patients who received corticosteroids preoperatively may require stress-dose corticosteroids during the postoperative period. Prophylaxis with H2 blockers may reduce gastrointestinal hemorrhage in critically ill patients199 but may also increase the risk of nosocomial infections.200 Gastrointestinal bleeding is more common after resection of a posterior fossa tumor, and use of prophylaxis has been advocated in these patients.201
Other Critical Central Nervous System Disorders in Infants and Children
There are other critical CNS disorders in infants and children, including hepatic encephalopathy, hypertensive encephalopathy, and Reye syndrome. Discussion of these less common disorders is beyond the scope of this chapter, and the reader is referred to the appropriate primary references or other textbooks focused on pediatric critical care medicine. Reye syndrome was once a key disorder in the field of pediatric neurointensive care—reaching a peak of 555 cases in the United States in 1980. In the past decade, fewer than 2 cases per year have been reported.202
Key Points
Chiron C, Raynaud C, Maziere B, et al. Changes in regional cerebral blood flow during brain maturation in children and adolescents. J Nucl Med. 1992;33:696-703.
The most comprehensive study of normal CBF in infants and children.
DeVeber G, Kirkham F. Guidelines for the treatment and prevention of stroke in children. Lancet. 2008;7:983-985.
Kitamura T, Iwami T, Kawamura T, Nagao K, Tanaka H, Nadkarni VM, et al. implementation working group for All-Japan Utstein Registry of the Fire and Disaster Management Agency. Conventional and chest-compression-only cardiopulmonary resuscitation by bystanders for children who have out-of-hospital cardiac arrests: a prospective, nationwide, population-based cohort study. Lancet. 2010;375:1347-1354.
Kochanek PM, Tasker RC. Pediatric neurointensive care: 2008 update for the Rogers’ textbook of pediatric intensive care. Pediatr Crit Care Med. 2009;10:517-523.
Contemporary review of key new developments in the emerging field of pediatric neurocritical care.
Lacroix J, Deal C, Gauthier M, et al. Admissions to a pediatric intensive care unit for status epilepticus: a 10-year experience. Crit Care Med. 1994;22:827-832.
Laptook A, Tyson J, Shankaran S, McDonald S, Ehrenkranz R, Fanaroff A, et al. National Institute of Child Health and Human Development Neonatal Research Network. Elevated temperature after hypoxic-ischemic encephalopathy: risk factor for adverse outcomes. Pediatrics. 2008;122:491-499.
Peltola H, Roine I, Fernández J, Zavala I, Ayala SG, Mata AG, et al. Adjuvant glycerol and/or dexamethasone to improve the outcomes of childhood bacterial meningitis: a prospective, randomized, double-blind, placebo-controlled trial. Clin Infect Dis. 2007;45:1277-1286.
Shankaran S, Laptook AR, Ehrenkranz RA, Tyson JE, McDonald SA, Donovan EF, et al. National Institute of Child Health and Human Development Neonatal Research Network. Whole-body hypothermia for neonates with hypoxic-ischemic encephalopathy. N Engl J Med. 2005;353:1574-1584.
Topjian AA, Berg RA, Nadkarni VM. Pediatric cardiopulmonary resuscitation: advances in science, techniques, and outcomes. Pediatrics. 2008;122:1086-1098.
Comprehensive review of the pathobiology and treatment of pediatric cardiac arrest.
Vavilala MS, Lee LA, Lam AM. The lower limit of autoregulation in children during sevoflurane anesthesia. J Neurosurg Anesthesiol. 2003;15:307-312.
1 Kochanek PM, Bell MJ, Forbes ML, et al. Pathophysiology. In: Marion DW, editor. Traumatic Brain Injury. New York: Thieme Medical; 1999:233-256.
2 Kochanek PM, Clark RS, Ruppel RA, et al. Biochemical, cellular, and molecular mechanisms in the evolution of secondary damage after severe traumatic brain injury in infants and children: Lessons learned from the bedside. Pediatr Crit Care Med. 2000;1:4-19.
3 Donaldson HH, Hatai S. On the weight of the parts of the brain and on the percentage of water in them according to brain weight and to age in albino and in wild Norway rats. J Comp Neurol. 1931;53:263-307.
4 Agrawal HC, Davis JM, Himwich WA. Water content of dog brain parts in relation to maturation of the brain. Am J Physiol. 1968;215:846-848.
5 Selzer ME, Myers RE, Holstein SB. Maturational changes in brain water and electrolytes in Rhesus monkey with some implications for electrogenesis. Brain Res. 1972;45:193-204.
6 Van Lookeren Campagne M, Verheul JB, Nicolay K, et al. Early evolution and recovery from excitotoxic injury in the neonatal rat brain: A study combining magnetic resonance imaging, electrical impedance, and histology. J Cereb Blood Flow Metab. 1994;14:1011-1023.
7 Caley DW, Maxwell DS. Development of the blood vessels and extracellular space during postnatal maturation of rat cerebral cortex. J Comp Neurol. 1970;138:31-48.
8 Evans AN, Reynolds JM, Reynolds ML, et al. Development of a blood-brain barrier mechanism in foetal sheep. J Physiol. 1974;238:371-386.
9 Schleien CL, Koehler RC, Shaffner H, et al. Blood-brain barrier disruption after cardiopulmonary resuscitation in immature swine. Stroke. 1991;22:477-483.
10 Schleien CL, Koehler RC, Shaffner DH, et al. Blood-brain barrier integrity during cardiopulmonary resuscitation in dogs. Stroke. 1990;21:185-191.
11 Lou HC, Lassen NA, Tweed WA, et al. Pressure passive cerebral blood flow and breakdown of the blood-brain barrier in experimental fetal asphyxia. Acta Paediatr Scand. 1979;68:57-63.
12 Nehlig A, Vasconcelos A, Boyet S. Postnatal changes in local cerebral blood flow measured by the quantitative autoradiographic [14C] iodoantipyrine technique in freely moving rats. J Cereb Blood Flow Metab. 1989;9:579-588.
13 Moore TJ, Lione AP, Regen DM, et al. Brain glucose metabolism in the newborn rat. Am J Physiol. 1971;221:1746-1753.
14 Himwich HE, Fazekas JF. Comparative studies of the metabolism of the brain of infant and adult dogs. Am J Physiol. 1941;132:454-459.
15 Kennedy C, Grave JD, Jehle JW, et al. Changes in blood flow in component structures of the dog brain during postnatal maturation. J Neurochem. 1972;19:2423-2433.
16 Chugani HT, Phelps ME. Maturational changes in cerebral function in infants determined by 18FDG positron emission tomography. Science. 1986;231:840-843.
17 Chugani HT, Phelps ME, Mazziotta JC. Positron emission tomography study of human brain functional development. Ann Neurol. 1987;22:487-497.
18 Volpe JJ, Herscovitch P, Perlman JM, et al. Positron emission tomography in the newborn: Extensive impairment of regional cerebral blood flow with intraventricular hemorrhage and hemorrhagic intracerebral involvement. Pediatrics. 1983;72:589-601.
19 Chiron C, Raynaud C, Maziere B, et al. Changes in regional cerebral blood flow during brain maturation in children and adolescents. J Nucl Med. 1992;33:696-703.
20 Muizelaar JP, Marmarou A, DeSalles AAF, et al. Cerebral blood flow and metabolism in severely head-injured children: I. Relationship with GCS score, outcome, ICP, and PVI. J Neurosurg. 1989;71:63-71.
21 Muizelaar JP, Ward JD, Marmarou A, et al. Cerebral blood flow and metabolism in severely head-injured children: II. Autoregulation. J Neurosurg. 1989;71:72-76.
22 Dobbing J, Sands J. Quantitative growth development of human brain. Arch Dis Child. 1973;48:757-767.
23 Brody BA, Kinney HC, Kloman AS, et al. Sequence of central nervous system myelination in human infancy: I. An autopsy study of myelination. J Neuropathol Exp Neurol. 1987;46:283-301.
24 Zwienenberg M, Muixrlsst JP. Severe pediatric head injury: The role of hyperemia revisited. J Neurotrauma. 1999;16:37-43.
25 Obrist WD, Langfitt TW, Jaggi JL, et al. Cerebral blood flow and metabolism in comatose patients with acute head injury. J Neurosurg. 1984;61:241-253.
26 Keyt SS, King BD, Horvath SM, et al. The effect of an acute reduction in blood pressure by means of differential spinal sympathetic block on the cerebral circulation of hypertensive patients. J Clin Invest. 1950;29:402-407.
27 Olesen J. Quantitative evaluation of normal and pathologic cerebral blood flow regulation to perfusion pressure. Arch Neurol. 1973;28:143-149.
28 Pasternak JF, Groothuis DR. Autoregulation of cerebral blood flow in the newborn beagle puppy. Biol Neonate. 1985;48:100-109.
29 Laptook AR, Stonestreet BS. Oh W Brain blood flow and O2 delivery during hemorrhagic hypotension in the piglet. Pediatr Res. 1983;17:77-80.
30 Szymonowicz W, Walker AM, Yu VYH, et al. Regional cerebral blood flow after hemorrhagic hypotension in the preterm, near-term, and newborn lamb. Pediatr Res. 1990;28:361-366.
31 Raju TNK, Doshi UV, Vidyasagar D. Cerebral perfusion pressure studies in healthy preterm and term newborn infants. J Pediatr. 1982;100:139-142.
32 Goitein KJ, Tamir I. Cerebral perfusion pressure in central nervous system infections of infancy and childhood. J Pediatr. 1983;103:40-43.
33 Sharples PM, Stuart AG, Matthews DSF, et al. Cerebral blood flow and metabolism in children with severe head injury: I. Relation to age, Glasgow Coma Score, outcome, intracranial pressure, and time after injury. J Neurol Neurosurg Psychiatry. 1995;58:145-152.
34 Vavilala MS, Lee LA, Lam AM. The lower limit of autoregulation in children during sevoflurane anesthesia. J Neurosurg Anesthesiol. 2003;15:307-312.
35 Hutchison JS, Ward RE, Lacroix J, Hébert PC, Barnes MA, Bohn DJ, et al. Hypothermia Pediatric Head Injury Trial Investigators and the Canadian Critical Care Trials Group. Hypothermia after traumatic brain injury in children. N Engl J Med. 2008;358:2447-2456.
36 White JR, Farukhi Z, Bull C, et al. Predictors of outcome in severely head-injured children. Crit Care Med. 2001;29:534-540.
37 Vavilala MS, Bowen A, Lam AM, et al. Blood pressure and outcome after severe pediatric traumatic brain injury. J Trauma. 2003;55:1039-1044.
38 Faden AI, Demediuk P, Panter SS, et al. The role of excitatory amino acids and NMDA receptors in traumatic brain injury. Science. 1989;244:798-800.
39 Ruppel RA, Kochanek PM, Adelson PD, et al. Excitatory amino acid concentrations in ventricular cerebrospinal fluid after severe traumatic brain injury in infants and children: The role of child abuse. J Pediatr. 2001;138:18-25.
40 McDonald JW, Johnston MV. Physiological pathophysiological roles of excitatory amino acids during central nervous system development. Brain Res Rev. 1990;15:41-70.
41 McDonald JW, Silverstein FS, Johnston MV. Neurotoxicity of N-methyl-D-aspartate is markedly enhanced in developing rat central nervous system. Brain Res. 1988;459:200-203.
42 Tremblay E, Roisin MP, Repressa A, et al. Transient increased density of MDA binding sites in developing rat hippocampus. Brain Res. 1988;461:393-396.
43 Foster AC, Gill R, Kemp JA, et al. Systemic administration of MK-801 prevents N-methyl-D-aspartate induced neuronal degeneration in rat brain. Neurosci Lett. 1987;76:307-311.
44 Oin YO, Ikonomidou C, Labruyere J, et al. Effect of glutamate receptor antagonists on traumatic brain damage in neonatal rats. Soc Neurosci Abstr. 1995;21:762.
45 Richards DA, Tolias CM, Sgouros S, Bowery NG. Extracellular glutamine to glutamate ratio may predict outcome in the injured brain: A clinical microdialysis study in children. Pharmacol Res. 2003;48:101-109.
46 Tolias CM, Richards DA, Bowery NG, Sgouros S. Extracellular glutamate in the brains of children with severe head injuries: A pilot microdialysis study. Childs Nerv Syst. 2002;18:368-374.
47 Robertson CL, Bell MJ, Kochanek PM, et al. Increased adenosine in cerebrospinal fluid after severe traumatic brain injury in infants and children: Association with severity of injury and excitotoxicity. Crit Care Med. 2001;29:2287-2390.
48 Pohl D, Bittigau P, Ishimaru MJ, et al. N-Methyl-D-aspartate antagonists and apoptotic cell death triggered by head trauma in developing rat brain. Proc Natl Acad Sci U S A. 1999;96:2508-2513.
49 Rink A, Fung K-M, Trojanowski JQ, et al. Evidence of apoptotic cell death after experimental traumatic brain injury in the rat. Am J Pathol. 1995;147:1575-1583.
50 Clark RS, Kochanek PM, Chen M, et al. Increases in Bcl-2 and cleavage of caspase-1 and caspase-3 in human brain after head injury. FASEB J. 1999;13:813-821.
51 Ikonomidou C, Bosch F, Miksa M, et al. Blockade of NMDA receptors and apoptotic neurodegeneration in the developing brain. Science. 1999;283:70-74.
52 Jevtovic-Todorovic V, Hartman RE, Izumi Y, et al. Early exposure to common anesthetic agents causes widespread neurodegeneration in the developing rat brain and persistent learning deficits. J Neurosci. 2003;23:876-882.
53 Ikonomidou C, Bittigau P, Ishimaru MJ, et al. Ethanol-induced apoptotic neurodegeneration and fetal alcohol syndrome. Science. 2000;287:1056-1060.
54 Clark RS, Chen J, Watkins SC, et al. Apoptosis-suppressor gene BCL-2 expression after traumatic brain injury in rats. J Neurosci. 1997;17:9172-9182.
55 Seidberg NA, Clark RSB, Kochanek PM, et al. Soluble fat is increased in CSF from infants and children after head injury. Crit Care Med. 2000;27:A38.
56 Satchell MA, Kochanek PM, Graham SH, et al. IL-1 converting enzyme, pro IL-1 and cytochrome C in cerebrospinal fluid after head injury in pediatric patients. Crit Care Med. 2000;28:A52.
57 Kochanek PM. Biochemical, metabolic and molecular response in the brain after inflicted childhood neurotrauma. Presented before the American Academy of Pediatrics, Proceedings of the Inflicted Childhood Neurotrauma Conference. Sponsored by the Department of Health and Human Services (HHS), National Institutes of Health (NIH), National Institute of Child Health and Human Development (NICHD), Office of Rare Diseases (ORD), and the National Center for Medical Rehabilitation Research (NCMRR). Reece RM, Nicholson CE, editors. Inflicted Childhood Neurotrauma. October 10-11, 2002;191-201. Bethesda, MD
58 Chesnut RM, Marshall LF, Klauber MR, et al. The role of secondary brain injury in determining outcome from severe head injury. J Trauma. 1993;34:216-222.
59 Levin HS, Aldrich EF, Saydjari C, et al. Severe head injury in children: Experience of the Traumatic Coma Data Bank. Neurosurgery. 1992;31:435-444.
60 Kirsch JR, Helfaer MA, Blizzard K, et al. Age-related cerebrovascular response to global ischemia in pigs. Am J Physiol. 1990;259:H1-H7.
61 Cockburn F, Daniel SS, Dawes GS, et al. The effect of pentobarbital anesthesia on resuscitation and brain damage in fetus rhesus monkeys asphyxiated on delivery. J Pediatr. 1969;75:281.
62 Daniel SS, Dawes GS, James LS, et al. Hypothermia and the resuscitation of asphyxiated fetal rhesus monkeys. J Pediatr. 1966;68:45.
63 Ment LR, Stewart WB, Duncan CC, et al. Beagle pup model of perinatal asphyxia: Nimodipine studies. Stroke. 1987;18:599.
64 Miller JR, Myers RE. Neuropathology of systematic circulatory arrest in adult monkeys. Neurology. 1972;22:888.
65 Kochanek PM, Uhl MW, Schoettle RJ. Hypoxic ischemic encephalopathy: Pathobiology and therapy of the post-resuscitation syndrome in children. In: Fuhrman B, Zimmerman J, editors. Pediatric Critical Care Medicine. St. Louis: CV Mosby; 1992:637-657.
66 Duhaime AC, Genneralli TA, Thibault LE, et al. The shaken baby syndrome: A clinical pathological and biochemical study. J Neurosurg. 1987;66:409-415.
67 Ichord RN, Naim M, Pollock AN, Nance ML, Margulies SS, Christian CW. Hypoxic-ischemic injury complicates inflicted and accidental traumatic brain injury in young children: the role of diffusion-weighted imaging. J Neurotrauma. 2007;24:106-118.
68 Berger RP, Adelson PD, Richichi R, Kochanek PM. Serum biomarkers after traumatic and hypoxemic brain injuries: insight into the biochemical response of the pediatric brain to inflicted brain injury. Dev Neurosci. 2006;28:327-335.
69 Yamamoto M, Meyer JS, Sakai F, Yamaguchi F. Aging and cerebral vasodilator responses to hypercarbia: Responses in normal aging and in persons with risk factors for stroke. Arch Neurol. 1980;37:489-496.
70 Webster MW, Makaroun MS, Steed DL, et al. Compromised cerebral blood flow reactivity is a predictor of stroke in patients with symptomatic carotid artery occlusive disease. J Vasc Surg. 1995;21:338-344.
71 Raol YH, Lynch DR, Brooks-Kayal AR. Role of excitatory amino acids in development epilepsies. Ment Retard Dev Disabil Res Rev. 2001;7:254-260.
72 Naritomi H, Meyer JS, Sakai F, et al. Effects of advancing age on regional cerebral blood flow: Studies in normal subjects and subjects with risk factors for atherothrombotic stroke. Arch Neurol. 1979;36:410-416.
73 Rogers RL, Meyer JS, Shaw TG, et al. The effects of chronic cigarette smoking on cerebrovascular responsiveness to 5 per cent CO2 and 100 per cent O2 inhalation. J Am Geriatr Soc. 1984;32:415-420.
74 Rimel RW, Giordani B, Barth JT, et al. Disability caused by minor head injury. Neurosurgery. 1981;9:221-228.
75 Rimel RW, Giodani B, Barth JT, Jane JA. Moderate head injury: Completing the clinical spectrum of brain trauma. Neurosurgery. 1982;11:344-351.
76 Ruff RM, Marshall LF, Klauber MR, et al. Alcohol abuse and neurological outcome of the severely head injured. J Head Trauma Rehabil. 1990;5:21-31.
77 Kelly DF, Kozlowski DA, Haddad E, et al. Ethanol reduces metabolic uncoupling following experimental head injury. J Neurotrauma. 2000;17:261-272.
78 Sirbaugh PE, Pepe PE, Shook JE, et al. A prospective, population-based study of the demographics, epidemiology, management, and outcome of out-of-hospital pediatric cardiopulmonary arrest [see comments] [published erratum appears in Ann Emerg Med 1999 Mar;33(3):358]. Ann Emerg Med. 1999;33:174-184.
79 Kuisma M, Suominen P, Korpela R. Paediatric out-of-hospital cardiac arrests—epidemiology and outcome. Resuscitation. 1995;30:141-150.
80 Young KD, Seidel JS. Pediatric cardiopulmonary resuscitation: A collective review [see comments]. Ann Emerg Med. 1999;33:195-205.
81 Hickey RW, Cohen DM, Strausbaugh S, Dietrich A. Pediatric patients requiring CPR in the prehospital setting. Ann Emerg Med. 1995;25:495-501.
82 Appleton GO, Cummins RO, Larson MP, Graves JR. CPR and the single rescuer: At what age should you “call first” rather than “call fast?”. Ann Emerg Med. 1995;25:492-494.
83 Mogayzel C, Quan L, Graves JR, et al. Out-of-hospital ventricular fibrillation in children and adolescents: Causes and outcomes. Ann Emerg Med. 1995;25:484-491.
84 Reis AG, Nadkarni V, Perondi MB, et al. A prospective investigation into the epidemiology of in-hospital pediatric cardiopulmonary resuscitation using the international Utstein reporting style. Pediatrics. 2002;109:200-209.
85 Friesen RM, Duncan P, Tweed WA. Appraisal of pediatric cardiopulmonary resuscitation. Can Med Assoc J. 1982;16:1055.
86 Zaritsky A, Nadkarni V, Getson P. CPR in children. Ann Emerg Med. 1987;16:1107.
87 Kyriacou DN, Arcinue EL, Peek C, Kraus JF. Effect of immediate resuscitation on children with submersion injury. Pediatrics. 1994;94:137.
88 Schindler MB, Bohn D, Cox PN, et al. Outcome of out-of-hospital cardiac or respiratory arrest in children. N Engl J Med. 1996;335:1473-1479.
89 De Meirleir LJ, Taylor MJ. Prognostic utility of SEPs in comatose children. Pediatr Neurol. 1987;3:78-82.
90 Taylor MJ, Farrell EJ. Comparison of the prognostic utility of VEPs and SEPs in comatose children. Pediatr Neurol. 1989;5:145-150.
91 Goodwin SR, Friedman WA, Bellefleur M. Is it time to use evoked potentials to predict outcome in comatose children and adults? Crit Care Med. 1991;19:518-524.
92 Beca J, Cox PN, Taylor MJ, et al. Somatosensory evoked potentials for prediction of outcome in acute severe brain injury. J Pediatr. 1995;126:44-49.
93 Carter BG, Taylor A, Butt W. Severe brain injury in children: Long-term outcome and its prediction using somatosensory evoked potentials (SEPs). Intensive Care Med. 1999;25:722-728.
94 Heindl UT, Laub MC. Outcome of persistent vegetative state following hypoxic or traumatic brain injury in children and adolescents. Neuropediatrics. 1996;27:94-100.
95 Mandel R, Martinot A, Delepoulle F, et al. Prediction of outcome after hypoxic-ischemic encephalopathy: A prospective clinical and electro-physiologic study. J Pediatr. 2002;141:45-50.
96 Jacinto SJ, Gieron-Korthals M, Ferreira JA. Predicting outcome in hypoxic-ischemic brain injury. Pediatr Clin North Am. 2001;48:647-660.
97 Kitamura T, Iwami T, Kawamura T, Nagao K, Tanaka H, Nadkarni VM, et al. implementation working group for All-Japan Utstein Registry of the Fire and Disaster Management Agency. Conventional and chest-compression-only cardiopulmonary resuscitation by bystanders for children who have out-of-hospital cardiac arrests: a prospective, nationwide, population-based cohort study. Lancet. 2010;375:1347-1354.
98 Samson RA, Berg RA, Bingham R. Use of automated external defibrillators for children: An update—an advisory statement from the Pediatric Advanced Life Support Task Force, International Liaison Committee on Resuscitation. Pediatrics. 2003;112:163-168.
99 Gausche M, Lewis RJ, Stratton SJ, et al. Effect of out-of-hospital pediatric endotracheal intubation on survival and neurological outcome: A controlled clinical trial. JAMA. 2000;283:783-790.
100 Lefkowitz W. Oxygen and resuscitation: Beyond the myth. Pediatrics. 2002;109:517-519.
101 Kilgannon JH, Jones AE, Shapiro NI, Angelos MG, Milcarek B, Hunter K, et al. Emergency Medicine Shock Research Network (EMShockNet) Investigators. Association between arterial hyperoxia following resuscitation from cardiac arrest and in-hospital mortality. JAMA. 2010;303:2165-2171.
102 Buunk G, van der Hoeven JG, Meinders AE. Cerebrovascular reactivity in comatose patients resuscitated from a cardiac arrest. Stroke. 1997;28:1569-1573.
103 Tobias JD, Lynch A, Garrett J. Alterations of end-tidal carbon dioxide during the intrahospital transport of children. Pediatr Emerg Care. 1996;12:249-251.
104 American Heart Association. Guidelines 2000 for Cardiopulmonary Resuscitation and Emergency Cardiovascular Care, 8th ed. Dallas, TX: American Heart Association; 2000.
105 Dorian P, Cass D, Schwartz B, et al. Amiodarone as compared with lidocaine for shock-resistant ventricular fibrillation. N Engl J Med. 2002;346:884-890.
106 Mann K, Berg RA, Nadkarni V. Beneficial effects of vasopressin in prolonged pediatric cardiac arrest: A case series. Resuscitation. 2002;52:149-156.
107 Liedel JL, Meadow W, Nachman J, et al. Use of vasopressin in refractory hypotension in children with vasodilatory shock: Five cases and a review of the literature. Pediatr Crit Care Med. 2002;3:15-18.
108 Dalton HJ, Siewers RD, Fuhrman BP, et al. Extracorporeal membrane oxygenation for cardiac rescue in children with severe myocardial dysfunction. Crit Care Med. 1993;21:1020-1028.
109 del Nido PJ. Extracorporeal membrane oxygenation for cardiac support in children. Ann Thorac Surg. 1996;61:336-339.
110 Duncan BW, Ibrahim AE, Hraska V, et al. Use of rapid-deployment extracorporeal membrane oxygenation for the resuscitation of pediatric patients with heart disease after cardiac arrest. J Thorac Cardiovasc Surg. 1998;116:305-311.
111 Posner JC, Osterhoudt KC, Mollen CJ, et al. Extracorporeal membrane oxygenation as a resuscitative measure in the pediatric emergency department. Pediatr Emerg Care. 2000;16:413-415.
112 Parra DA, Totapally BR, Zahn E, et al. Outcome of cardiopulmonary resuscitation in a pediatric cardiac intensive care unit. Crit Care Med. 2000;28:3296-3300.
113 Hypothermia after Cardiac Arrest Study Group. Mild therapeutic hypothermia to improve the neurologic outcome after cardiac arrest. N Engl J Med. 2002;346:549-556.
114 Bernard SA, Gray TW, Buist MD, et al. Treatment of comatose survivors of out-of-hospital cardiac arrest with induced hypothermia. N Engl J Med. 2002;346:557-563.
115 Hickey RW, Kochanek PM, Ferimer H, et al. Hypothermia and hyperthermia in children after resuscitation from cardiac arrest. Pediatrics. 2000;106:118-122.
116 Doherty DR, Parshuram CS, Gaboury I, Hoskote A, Lacroix J, Tucci M, et al. Canadian Critical Care Trials Group. Hypothermia therapy after pediatric cardiac arrest. Circulation. 2009;119:1492-1500.
117 Fink EL, Clark RS, Kochanek PM, Bell MJ, Watson RS. A tertiary care center’s experience with therapeutic hypothermia after pediatric cardiac arrest. Pediatr Crit Care Med. 2010;11:66-74.
118 Shankaran S, Laptook AR, Ehrenkranz RA, Tyson JE, McDonald SA, Donovan EF, et al. National Institute of Child Health and Human Development Neonatal Research Network. Whole-body hypothermia for neonates with hypoxic-ischemic encephalopathy. N Engl J Med. 2005;353:1574-1584.
119 Azzopardi DV, Strohm B, Edwards AD, Dyet L, Halliday HL, Juszczak E, et al. Moderate hypothermia to treat perinatal asphyxial encephalopathy. TOBY Study Group. N Engl J Med. 2009;361:1349-1358.
120 Gluckman PD, Wyatt JS, Azzopardi D, Ballard R, Edwards AD, Ferriero DM, et al. Selective head cooling with mild systemic hypothermia after neonatal encephalopathy: multicentre randomised trial. Lancet. 2005;365:663-670.
121 Laptook A, Tyson J, Shankaran S, McDonald S, Ehrenkranz R, Fanaroff A, et al. National Institute of Child Health and Human Development Neonatal Research Network. Elevated temperature after hypoxic-ischemic encephalopathy: risk factor for adverse outcomes. Pediatrics. 2008;122:491-499.
122 Morimoto Y, Kemmotsu O, Kitami K, et al. Acute brain swelling after out-of-hospital cardiac arrest: Pathogenesis and outcome. Crit Care Med. 1993;21:104-110.
123 Bohn DJ, Biggar WD, Smith CR, et al. Influence of hypothermia, barbiturate therapy, and intracranial pressure monitoring on morbidity and mortality after near-drowning. Crit Care Med. 1986;14:529-534.
124 Boie ET, Moore GP, Brummett C, Nelson DR. Do parents want to be present during invasive procedures performed on their children in the emergency department? A survey of 400 parents. Ann Emerg Med. 1999;34:70-74.
125 Doyle CJ, Post H, Burney RE, et al. Family participation during resuscitation: An option. Ann Emerg Med. 1987;16:673-675.
126 Hanson C, Strawser D. Family presence during cardiopulmonary resuscitation: Foote Hospital emergency department’s nine-year perspective. J Emerg Nurs. 1992;18:104-106.
127 Meyers TA, Eichhorn DJ, Guzzetta CE. Do families want to be present during CPR? A retrospective survey. J Emerg Nurs. 1998;24:400-405.
128 Tsai E. Should family members be present during cardiopulmonary resuscitation. N Engl J Med. 2002;346:1019-1021.
129 Robinson SM, Mackenzie-Ross S, Campbell Hewson GL, et al. Psychological effect of witnessed resuscitation on bereaved relatives. Lancet. 1998;352:614-617.
130 Mitchell WG. Status epilepticus and acute repetitive seizures in children, adolescents, and young adults: Etiology, outcome, and treatment. Epilepsia. 1996;37:S74-S80.
131 DeLorenzo RJ, Hauser WA, Towne AR. A prospective, population-based epidemiologic study of status epilepticus in Richmond, Virginia. Neurology. 1996;46:1029-1035.
132 Shinnar S, Pellock JM, Moshe SL, et al. In whom does status epilepticus occur: Age-related differences in children. Epilepsia. 1997;38:907-914.
133 Lacroix J, Deal C, Gauthier M, et al. Admissions to a pediatric intensive care unit for status epilepticus: A 10-year experience. Crit Care Med. 1994;22:827-832.
134 Maytal J, Shinnar S, Moshe SL, Alvarez LA. Low morbidity and mortality of status epilepticus in children. Pediatrics. 1989;83:323-331.
135 Shinnar S, Maytal J, Krasnoff L, Moshe SL. Recurrent status epilepticus in children. Ann Neurol. 1992;31:598-604.
136 Tasker RC. Neurological critical care. Curr Opin Pediatr. 2000;12:222-226.
137 Pellock JM. Status epilepticus. 3rd ed. Swaiman KF, Ashwal S, editors. Pediatric Neurology, Principles and Practice. vol 1. St. Louis: Mosby; 1999.
138 Treatment of Convulsive Status Epilepticus. Recommendations of the Epilepsy Foundation of America’s Working Group on Status Epilepticus. JAMA. 1993;270:854-859.
139 Treiman DM, Meyers PD, Walton NY, et al. A comparison of four treatments for generalized convulsive status epilepticus. N Engl J Med. 1998;339:792-798.
140 Chiulli DA, Terndrup TE, Kanter RK. The influence of diazepam or lorazepam on the frequency of endotracheal intubation in childhood status epilepticus. J Emerg Med. 1991;9:13-17.
141 Tasker RC. Emergency treatment of acute seizures and status epilepticus. Arch Dis Child. 1998;79:78-83.
142 Appleton R, Macleod S, Martland T. Drug management for acute tonic-clonic convulsions including convulsive status epilepticus in children. Cochrane Database Syst Rev 2008 Jul 16;(3):CD001905.
143 Bleck T. Management approaches to prolonged seizures and status epilepticus. Epilepsia. 1999;40:S59-S63.
144 Igartua J, Silver P, Maytal J, Sagy M. Midazolam coma for refractory status epilepticus in children. Crit Care Med. 1999;27:1982-1985.
145 deVeber G. Arterial ischemic strokes in infants and children: An overview of current approaches. Semin Thromb Hemost. 2003;29:567-573.
146 Chabrier S, Rodesch G, Lasjaunias P, et al. Transient cerebral arteriopathy: A disorder recognized by serial angiograms in children with stroke. J Child Neurol. 1998;13:27-32.
147 deVeber G, Andrew M, Adams C, et al. Cerebral sinovenous thrombosis in children. (for the Canadian Pediatric Ischemic Stroke Study Group). N Engl J Med. 2001;345:417-423.
148 Domi T, Edgell D, McCrindle BW, et al. Frequency and predictors of vaso-occlusive strokes associated with congenital heart disease [abstract]. Ann Neurol. 2002;52(Suppl 3):S129-S133.
149 Levy SR, Abroms IF, Marshall PC, Rosquete EE. Seizures and cerebral infarction in the full-term newborn. Ann Neurol. 1985;17:366-370.
150 deVeber G, and The Canadian Pediatric Ischemic Stroke Study Group. Canadian Paediatric Ischemic Stroke Registry: Analysis of children with arterial ischemic stroke [abstract]. Ann Neurol. 2000;48:526.
151 Alehan D, Ozkutu S, Ayabakan C, et al. Complications and outcome in left-sided endocarditis in children. Turk J Pediatr. 2002;44:5-12.
152 Karande SC, Kulthe SG, Lahiri KR, Jain MK. Embolic stroke in a child with idiopathic dilated cardiomyopathy. J Postgrad Med. 1996;42:84-86.
153 Strater R, Kurnik K, Heller C, et al. Aspirin versus low-dose low-molecular-weight heparin: Antithrombotic therapy in pediatric ischemic stroke patients: A prospective follow-up study. Stroke. 2001;32:2554-2558.
154 Carvalho KS, Garg BP. Arterial strokes in children. Neurol Clin. 2002;20:1079-1100.
155 The Child Neurological Society ad hoc Committee on Stroke in Children. Available at. http//www.stroke-site.org/guidelines/childneuro_stmt.html.
156 Kirton A, Wong JH, Mah J, et al. Successful endovascular therapy for acute basilar thrombosis in an adolescent. Pediatrics. 2003;112:e248-e251.
157 DeVeber G, Kirkham F. Guidelines for the treatment and prevention of stroke in children. Lancet. 2008;7:983-985.
158 Adelson PD, Bratton SL, Carney NA, et al. Guidelines for the Acute Medical Management of Severe Traumatic Brain Injury in Infants, Children, and Adolescents. Pediatr Crit Care Med. 2003;4:S1-S75.
159 Meyer PG, Orliaguet GA, Zerah M, et al. Emergency management of deeply comatose children with acute rupture of cerebral arteriovenous malformations. Can J Anaesth. 2000;47:758-766.
160 Wijdicks EFM. Management of Specific Disorders. In The Clinical Practice of Critical Care Neurology, 2nd ed. New York: Oxford University Press; 2003. p 228
161 Inamasu J, Ichikizaki K, Matsumoto S, et al. Mild hypothermia for hemispheric cerebral infarction after evacuation of an acute subdural hematoma in an infant. Childs Nerv Syst. 2002;18:175-178.
162 Lee MC, Frank JI, Kahana M, et al. Decompressive hemicraniectomy in a 6-year-old male after unilateral hemispheric stroke: Case report and review. Pediatr Neurosurg. 2003;38:181-185.
163 Golomb MR, deVeber GA, MacGregor DL, et al. Independent walking after neonatal arterial ischemic stroke and sinovenous thrombosis. J Child Neurol. 2003;18:530-536.
164 Willis JK, Morello A, Davie A, et al. Forced use treatment of childhood hemiparesis. Pediatrics. 2002;110(1 Pt 1):94-96.
165 Rutten GJ, Ramsey NF, van Rijen PC, et al. Interhemispheric reorganization of motor hand function to the primary motor cortex predicted with functional magnetic resonance imaging and transcranial magnetic stimulation. J Child Neurol. 2002;17:292-297.
166 Lynch JK. Cerebrovascular disorders in children. Curr Neurol Neurosci Rep. 2004;4:129-138.
167 Dawson KG, Emerson JC, Burns JL. Fifteen years of experience with bacterial meningitis. Pediatr Infect Dis J. 1999;18:816-822.
168 Chaudhuri A. Adjunctive dexamethasone treatment in acute bacterial meningitis. Lancet Neurol. 2004;3:54-62.
169 Pomeroy SL, Holmes SJ, Dodge PR, Feigin RD. Seizures and other neurologic sequelae of bacterial meningitis in children. N Engl J Med. 1990;323:1651-1657.
170 Bonsu BK, Harper MB. Fever interval before diagnosis, prior antibiotic treatment, clinical outcome for young children with bacterial meningitis. Clin Infect Dis. 2001;32:566-572.
171 Grimwood K, Anderson P, Anderson V, et al. Twelve year outcome following bacterial meningitis: Further evidence for persistent effects. Arch Dis Child. 2000;83:111-116.
172 van de Beek D, de Gans J, McIntyre P, Prasad K. Corticosteroids in acute bacterial meningitis. Cochrane Database Syst Rev 2003;3:CD004305.
173 Peltola H, Roine I, Fernández J, Zavala I, Ayala SG, Mata AG, et al. Adjuvant glycerol and/or dexamethasone to improve the outcomes of childhood bacterial meningitis: a prospective, randomized, double-blind, placebo-controlled trial. Clin Infect Dis. 2007;45:1277-1286.
174 Peltola H, Roine I, Fernández J, González Mata A, Zavala I, Gonzalez Ayala S, et al. Hearing impairment in childhood bacterial meningitis is little relieved by dexamethasone or glycerol. Pediatrics. 2010 Jan;125(1):e1-e8. Epub 2009 Dec 14
175 Ackerman AD, Singhi S. Pediatric infectious diseases: 2009 update for the Rogers’ Textbook of Pediatric Intensive Care. Pediatr Crit Care Med. 2010 Jan;11(1):117-123.
176 Gwer S, Gatakaa H, Mwai L, Idro R, Newton CR. The role for osmotic agents in children with acute encephalopathies: a systematic review. BMC Pediatr. 2010 Apr 17;10:23.
177 Connor SEJ, Jarosz JM. Magnetic resonance imaging of the cerebral venous sinus thrombosis. Clin Radiol. 2002;57:449-461.
178 Bonthius D, Karacay B. Meningitis and encephalitis in children: An update. Neurol Clin. 20, 2002.
179 Baltagi SA, Shoykhet M, Felmet K, Kochanek PM, Bell MJ. Neurological sequelae of 2009 influenza A (H1N1) in children: a case series observed during a pandemic. Pediatr Crit Care Med. 2010;11:179-184.
180 Redington JJ, Tyler KL. Viral infections of the nervous system: Update on diagnosis and treatment. Arch Neurol. 2002;59:712-718.
181 Kleinschmidt-DeMasters BK, Gilden DH. The expanding spectrum of herpes virus infections of the nervous system. Brain Pathol. 2001;11:440-451.
182 Chaudhuri A, Kennedy PGE. Diagnosis and treatment of viral encephalitis. Postgrad Med J. 2002;78:575-583.
183 Cinque P, Bossolasco S, Lundkvist A. Molecular analysis of cerebrospinal fluid in viral diseases of central nervous system. J Clin Virol. 2003;26:1-28.
184 Watemberg N, Morton L. Periodic lateralized epileptiform discharges. N Engl J Med. 1996;334:634.
185 Carcillo J, Field A. American College of Critical Care Medicine Task Force Committee Members: Clinical practice parameters for hemodynamic support of pediatric and neonatal patients in septic shock. Crit Care Med. 2002;30:1365-1378.
186 Goitein KJ, Amit Y, Mussaffi H. Intracranial pressure in central nervous system infections and cerebral ischaemia of infancy. Arch Dis Child. 1983;58:184-186.
187 Rebaud P, Berthier JC, Hartemann E, Floret D. Intracranial pressure in childhood central nervous system infections. Intensive Care Med. 1988;14:522-525.
188 Rennick G, Shann F, de Campo J. Cerebral herniation during bacterial meningitis in children. BMJ. 1993;306:953-955.
189 Lindvall P, Ahlm C, Ericsson M, et al. Reducing intracranial pressure may increase survival among patients with bacterial meningitis. Clin Infect Dis. 2004;38:384-390.
190 Grände PO, Myhre E, Nordstrom CH, Schliamser S. Treatment of intracranial hypertension and aspects on lumbar dural puncture in severe bacterial meningitis. Acta Anaesthesiol Scand. 2002;46:264-270.
191 Barnett GH, Ropper AH, Romeo J. Intracranial pressure and outcome in adult encephalitis. J Neurosurg. 1988;68:585-588.
192 Schwab S, Junger E, Spranger M, et al. Craniectomy: An aggressive treatment approach in severe encephalitis. Neurology. 1997;48:412-417.
193 Saez-Llorens A. Brain abscess in children. Semin Pediatr Infect Dis. 2003;14:108-114.
194 Brook I. Brain abscesses in children: Microbiology and management. J Child Neurol. 1995;10:283-288.
195 Eulmesekian PG, Pérez A, Minces PG, Bohn D. Hospital-acquired hyponatremia in postoperative pediatric patients: prospective observational study. Pediatr Crit Care Med. 2010;11:479-483.
196 Montañana PA, Modesto I, Alapont V, Ocón AP, López PO, López Prats JL, Toledo Parreño JD. The use of isotonic fluid as maintenance therapy prevents iatrogenic hyponatremia in pediatrics: a randomized, controlled open study. Pediatr Crit Care Med. 2008;9:589-597.
197 Fabling JM, Gan TJ, El-Moalem HE, et al. A randomized, double-blinded comparison of ondansetron, droperidol, and placebo for prevention of postoperative nausea and vomiting after supratentorial craniotomy. Anesth Analg. 2000;91:358-361.
198 Gutin PH. Corticosteroid therapy in patients with brain tumors. Natl Cancer Inst Monogr. 1977;46:151-156.
199 Levine B, Sirinek K, Mcleod C. The role of cimetidine in the prevention of stress-induced gastric mucosal injury. Surg Gynecol Obstet. 1979;148:399-402.
200 MacLean L. Prophylactic treatment of stress ulcers: First do no harm. Can J Surg. 1988;31:76-77.
201 Ross AJ, Siegel KR, Bell W, et al. Massive gastrointestinal hemorrhage in children with posterior fossa tumors. J Pediatr Surg. 1987;22:633-636.
202 Chow EL, Cherry JD, Harrison R, et al. Reassessing Reye syndrome. Arch Pediatr Adolesc Med. 2003;157:1241.