Chapter 64 Intraoperative Neurophysiologic Monitoring
MONITORING FACIAL NERVE
History
Krause1 first described facial nerve monitoring in 1912 using a faradic stimulation during cochlear nerve section for tinnitus. Twitching of the ipsilateral facial muscles during stimulation helped him preserve the facial nerve, and the patient had transient facial weakness postoperatively. In the 1960s, dedicated facial nerve monitoring systems were developed. The Hilger stimulator2 was used principally in the assessment of facial paralysis, but was also used during surgery. Further developments in facial nerve monitoring occurred in the 1970s and 1980s. Delgado and colleagues3 described the use of electromyography (EMG) monitoring in cerebellopontine angle (CPA) surgery. Moller and Jannetta4 combined the specificity of EMG recording with the advantage of acoustic feedback to the surgeon.
Electromyography
Subdermal needle electrodes are most commonly used for facial nerve monitoring. They have the advantages of ease of use, low impedance, and stability (less likely to be displaced than surface discs or cups). Monitoring more than one muscle provides additional sensitivity and redundancy.5 For two-channel bipolar recording, a typical sensing montage includes a pair of electrodes in the orbicularis oculi approximately 1 cm apart and another pair in the orbicularis oris (Fig. 64-1). The ground electrode is placed in the forehead, and the anode for the monopolar nerve stimulator is inserted at the ipsilateral shoulder. The operating room is a noisy place with abundant electric interference. The connections are checked by tapping the electrodes and observing an audible and oscilloscopic response. This mechanical compound muscle action potential results in a characteristic sound from the monitor. A second check, when available, involves testing the impedance of the inserted electrodes.
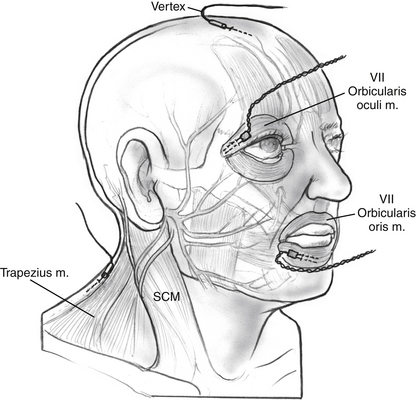
FIGURE 64-1 Electrode placement for facial nerve intraoperative monitoring. SCM, sternocleidomastoid.
Many facial nerve monitoring systems are available commercially, including the Nerve Integrity Monitor (Xomed, Inc., Jacksonville, FL) (Fig. 64-2), Neurosign 100 (Smith & Nephew Richards, Inc., Memphis, TN), Brackmann II (WR Medical Electronics Co., Stillwater, MN), and NEI (Grass Instrument Co., Quincy, MA). All of these devices use EMG. The Silverstein Facial Nerve Monitor (WR Medical Electronics Co.,) is an example of a motion detector device. Some systems, such as the Silverstein Monitor, include the ability to electrify instruments to aid with monitoring.
Stimulation
Pulsed stimulation may be safer and more efficacious.6 The parameters of safe nerve stimulation are 100 to 250 μs pulses with a range of 0.05 to 0.5 mA.5 The upper limit of safe nerve stimulation varies along the course of the nerve, but animal studies have shown myelin and axonal injury using 2 mA stimulus for 3 seconds.7 A 0.5 mA stimulus applied briefly 50 times was found to manifest no functional or histologic evidence of injury in a mouse model.8 Most normal facial nerves should be stimulated with direct contact of the probe using a 100 μs pulse of 0.05 mA. Settings of 0.05 to 0.1 mA are recommended when working close to the nerve. Farther from the nerve, currents of 0.2 to 0.3 mA may be used. Higher settings may be required when the nerve is covered by bone, connective tissue, or granulation tissue. Cerebrospinal fluid or blood may shunt current from a stimulator probe. In these cases, stimulation with a constant voltage may be used.
In an attempt to determine the threshold necessary to detect a surgical dehiscence of the facial nerve electrically, Choung and associates9 prospectively estimated the minimal threshold of electric current needed to change the EMG of facial muscles using the Nerve Integrity Monitor (NIM)-2 in 100 patients. They found that 43% of ears with surgical dehiscence responded to electric stimulation of 0.7 mA or less. The mean threshold of minimal electric stimulation was 0.29 mA for tympanic segments and 0.41 mA for mastoid segments.
Burst Activity
During the course of surgery, many burst potentials may be observed and are usually not associated with significant trauma to the nerve. Bursts are brief discharges in which the stimulus and response are simultaneous (see Fig. 64-2). Burst activity occurring with gentle manipulation suggests a healthy nerve. Very similar responses may be obtained, however, when drilling close to or after complete transection of the nerve. Lack of burst activity during dissection may be associated with minor manipulation of a healthy nerve, significant manipulation of an already injured nerve, or a problem with the monitoring connections and instruments. Electrically stimulating the nerve at this point verifies the integrity of the nerve.
Trains
Episodes of repetitive EMG activity may occur several seconds to minutes after the stimulus, making it difficult to identify the initiating factor or to modify dissection technique. As seen in Figure 64-2, trains are caused by prolonged depolarization of the nerve beyond its threshold for developing an action potential. Subsequent repetitive firing continues until the nerve repolarizes, or can no longer sustain the repetitive activation.5 The most common initiating factor is traction on the nerve. Trains may indicate significant trauma has occurred, although this is not always the case. Changes in temperature around the nerve may also precipitate train activity. When caused by cool irrigating fluid, the spontaneous activity usually subsides with warming. When a train pattern develops after laser application or cautery, however, thermal damage should be suspected. Elevated stimulation thresholds after repetitive nerve activity suggest significant injury. Higher frequency train activity (>50 Hz) with an amplitude greater than 250 μV is associated with a more ominous outcome; in a series of 51 patients, Nakao and coworkers10 found that 86% of patients with train activity having an amplitude greater than 250 μV had severe facial nerve dysfunction. More than 10 seconds of cumulative train time is associated with postoperative facial paresis.11
Anesthetic Issues
It is common practice that when facial nerve monitoring is employed, neuromuscular blocking agents are avoided after induction of anesthesia. In 2003, Kizilay and associates12 examined the effects of different levels of neuromuscular blockade (NMB) on electric stimulation thresholds of the facial nerve during otologic surgery. Minimal facial nerve stimulation causing EMG responses in the facial musculature was measured during recovery from the effects of muscular relaxants and with 25%, 50%, 75%, and 100% levels of NMB. All of the patients had detectable EMG responses of the facial musculature at the 50% and 75% levels of NMB in response to the electric stimulation (mean 0.1 mA) of the facial nerve. No responses were measured in 31% of the patients when the level of peripheral NMB was 100%. The investigators concluded that a regulated 50% level of peripheral NMB provides reliable intraoperative EMG monitoring of the facial musculature in response to electric stimulation and adequate anesthesia, with full immobilization of the patient. Chronic injury resulting from compression from a tumor may make the facial nerve more sensitive to the effects of NMB, however.13
Practical Application in Surgery
Locating the Facial Nerve
Monopolar stimulation is especially useful for mapping the nerve throughout its course.4 Monitoring becomes more important in cases of large tumors.5 A stronger stimulus may need to be used to confirm that the nerve is not in close proximity to an area of tumor to be removed. False-positive responses may be obtained from stimulation of the superior vestibular and cochlear nerves owing to electric current dispersion, especially within the internal auditory canal where the nerves lie in close proximity to one another. Stimulation of the trigeminal nerve may elicit motor activity that can be interpreted as facial nerve stimulation. False-negative responses are usually related to technical errors such as failure to connect the stimulus probe, anesthetic-induced muscle paralysis, or impedance imbalances.
Postoperative Prognosis and Prognosis of Acute Injury
The threshold for stimulation has been shown to correlate with postoperative facial nerve function. When using constant current stimulation with a 50 μs pulse, if the threshold for facial nerve stimulation at the brainstem was 0.1 mA or lower, 90% of patients exhibited House-Brackmann grade I or II function at 1 year after CPA surgery.14 Thresholds of 0.1 to 0.2 mA were associated with grade I or II function in 77% of cases.15 The amount of energy delivered to the nerve using a 50 μs pulse of 0.1 mA is equal in magnitude to a 100 μs pulse of 0.05 mA as delivered by many commercial facial nerve monitors. When testing the nerve at the root entry zone of the brainstem to determine threshold at the conclusion of the procedure, it is important to contact the nerve long enough to confirm the precisely timed pulses of electric stimulation. A single noise could be a mechanically evoked burst, which may give the surgeon a falsely optimistic estimate of nerve function.
In 2005, Grayeli and colleagues16 looked at the short-term facial prognostic value of a four-channel facial EMG device in vestibular schwannoma surgery. In 89 patients, EMG detection was performed in frontal, orbicularis oculi, orbicularis oris, and platysma muscles. Postoperative facial function at 6 months was assessed as House-Brackmann grade I or II in 80%, as grade III or IV in 16%, and as grade V or VI in 4% (n = 80). A proximal threshold between 0.01 and 0.04 mA had a positive predictive value of 94% for good facial function (grade I or II). The proximal threshold was lower in patients with improving or stable facial function compared with patients with a delayed deterioration between days 8 and 30. The maximal EMG response was detected in the frontal muscle or the platysma in 27% of cases and in orbicularis oris and oculi in 73% of cases.
Also in 2005, Neff and coworkers17 sought to evaluate prospectively whether the intraoperative stimulus threshold and response amplitude measurements from facial EMG can predict facial nerve function at 1 year after vestibular schwannoma resection. In 74 consecutive patients, the minimal stimulus intensity and EMG response amplitude were recorded during stimulation applied to the proximal facial nerve after vestibular schwannoma removal. Of the 74 patients, 66 of 74 (89%) had House-Brackmann grade I or II facial nerve function, and 8 of 74 (11%) had House-Brackmann grade III-VI function at 1 year after surgery. With intraoperative minimal stimulus intensity of 0.05 mA or less and response amplitude of 240 μV or greater, the authors were able to predict a House-Brackmann grade I or II outcome in 56 of 66 (85%) patients at 1 year after surgery. With these same electrophysiologic parameters, only 1 of 8 (12%) patients with House-Brackmann grade III-VI also met this standard and gave a false-positive result. Logistic regression analysis of the data showed that a stimulus threshold of 0.05 mA or less and a response amplitude of 240 μV or greater predicted a House-Brackmann grade I or II outcome with a 98% probability. Stimulus threshold or response amplitude alone had a much lower probability of the same result, however.
A similar study by Isaacson and colleagues18 looked at two independent intraoperative monitoring parameters in predicting long-term facial nerve function in 60 patients undergoing resection of vestibular schwannomas. They found that 5 of 60 (8.3%) patients showed significant long-term weakness (i.e., House-Brackmann grade III or worse). Intraoperative monitoring parameters (proximal stimulation threshold, proximal-to-distal response amplitude ratio) were accurate in predicting increased risk of long-term facial nerve dysfunction when used in a logistic regression model. Table 64-1 summarizes a selection of studies using facial nerve monitoring to evaluate postoperative prognosis in acoustic neuroma surgery38–47.
TABLE 64-1 Studies Using Facial Nerve Monitoring to Predict Facial Nerve Function after Acoustic Neuroma Surgery
Study | N | Result |
---|---|---|
Lin et al, 2006 | 38 | With 0.3 mA stimulus, proximal-distal amplitude >50% had PPV 93% for HB grade II or better immediately postoperative |
Grayeli et al, 200516 | 89 | Stimulation threshold of 0.01-0.04 mA had PPV 94% for HB grade II or better 180 days postoperative using four-channel EMG |
Neff et al, 200517 | 74 | For <0.05 mA stimulus and response amplitude of >0.240 V, authors could predict 85% of patients with HB grade II or better 1 yr postoperative |
Isaacson et al, 2003 | 229 | Using proximal-distal amplitude and stimulation threshold, authors developed regression function with sensitivity 89%, specificity 83%, PPV 94% in predicting HB grade III or worse in immediate postoperative period |
Fenton et al, 2002 | 67 | Using stimulation current and tumor size, authors developed regression function correctly describing 93% of patients at 2 yr follow-up |
Goldbrunner et al, 2000 | 137 | With proximal-distal amplitude >80%, 98.4% had HB grade I at 6 mo |
Nissen et al, 1997 | 116 | Median threshold for HB grade II or better group was 0.100 V versus 0.725 V for HB grade III or worse group |
Zeitouni et al, 1997 | 109 | HB grade III or worse long-term was related to higher minimum stimulation current |
Selesnick et al, 199615 | 49 | At 1 yr after surgery, 90% of patients stimulating at 0.1 mA had HB grade II or better |
Taha et al, 1995 | 20 | 100% HB grade III or better initial and grade I long-term (≤28 mo) function occurred if proximal-distal amplitude >67% |
Lalwani et al, 1994 | 129 | Of patients with stimulus of <0.2 V, 98% had HB grade II or better 1 year postoperative versus 50% if stimulus was >0.2 V |
Prasad et al, 1993 | 77 | When threshold after tumor removal was ≤0.2 V, 93% patients had early, and 85% had late postoperative HB grade I or II |
Niparko et al, 1989 | 29 | 83% HB grade I 1 wk postoperative if proximal-distal amplitude = 1 with 88% of these patients HB grade I 1 yr after surgery |
HB, House-Brackmann; PPV, positive predictive value.
Artifactual Responses
Artifacts (i.e., monitor activity that is not due to facial nerve stimulation) are common and may cause confusion. Electrocautery obliterates facial nerve responses by saturating the monitor with electric noise. Consequently, monitors have a muting function while electrocautery is in use. During muting, monitoring is disengaged. Ultrasonic aspirators also cause a large electric artifact, and may trigger a muting circuit.14 When accurate monitoring cannot be performed, many surgeons engage a member of the team to observe visually or palpate the patient’s face.
Troubleshooting the Facial Nerve Monitor
Direct communication with the anesthesiologist to confirm complete neuromuscular reversal is warranted. Checking along the electric circuit should be done, including electrode placement in the skin, electrode connections to the monitor, and probe connections. The volume of the monitor might have been turned down. A typical event threshold setting is 100 μV. Event threshold settings set too high may lead to a lack of a response. Conversely, false-positive results can be reduced by increasing the event threshold. The stimulus intensity should be confirmed and can be incrementally increased by 0.05 to 0.1 mA. In the middle ear, high sensitivity can be achieved with stimulus up to 0.7 mA.9 If a continuous current is used, stimulus should be changed to pulsed mode. Selesnick19 calculated the optimal stimulus duration of 50 μs, although many commercial monitors use a default pulse of 100 μs.
Antidromic Facial Nerve Monitoring
When a nerve is stimulated, in addition to conduction distally toward the neuromuscular junction (orthodromic), an action potential is conducted proximally (antidromically). The orthodromic wave produces the M wave on muscle contraction. The antidromic electric activity can be measured and used to assess neural function as the signal is reflected back toward the muscle resulting in a more subtle F wave. Wedekind and Klug20 prospectively evaluated F wave monitoring comparing it with intraoperative EMG, and found it useful monitoring facial nerve injury. They found that transient loss of F waves portends imminent severe facial dysfunction, and reported a 100% positive predictive value for unfavorable facial outcome with a permanent loss of F waves. A major advantage of antidromic monitoring is that it may be performed under NMB. Arriaga and associates21 described another use for antidromic potentials to locate the geniculate ganglion during middle fossa craniotomy.
MONITORING HEARING
Pathophysiology
Operating in the internal auditory canal and CPA poses two potential mechanisms for hearing loss: interruption of blood flow to the cochlea and the cochlear nerve, and injury to neural structures of the auditory pathway.22 In some cases, preoperative audiometric tests suggest a site of the lesion for the hearing loss. An inappropriately large reduction in speech discrimination score with good pure tone average suggests tumor effect on CN VIII or brainstem, rather than on the cochlea. Intact otoacoustic emissions (OAE) with poor auditory brainstem response (ABR) suggest the same.
Indications
Selecting appropriate cases for monitoring involves analysis of preoperative hearing, disease prognosis, and surgical approach. The role of monitoring hearing is to decrease the risk to the auditory nerve in CPA operations. Hearing can be monitored directly from the auditory nerve, and auditory evoked potentials (electrocochleography [ECoG] potentials) can be used to check the endolymphatic system in endolymphatic sac decompression operations. Operations in which monitoring of auditory function has been reported include microvascular decompression of cranial nerves, vestibular nerve section,21 and removal of vestibular schwannomas and other CPA masses. The most common application for monitoring of auditory function is the removal of vestibular schwannomas.
The generally accepted limits of serviceable hearing have been 50 dB HL pure tone average threshold and 50% speech discrimination score. Tumors in only hearing ears and bilateral tumors warrant special consideration. Worse hearing may indicate a more severe insult of auditory structures by disease and poor prognosis for hearing conservation. Exceptions exist, however, for hearing improvement when tumor removal alleviates a CN VIII conduction block.
In 2006, Samii and coworkers23 published their outcomes in a retrospective review of 200 consecutive vestibular schwannoma resections. They found that anatomic preservation of the facial nerve was possible in 98.5% of patients. By the last follow-up examination, excellent or good facial nerve function had been achieved in 81% of the cases. In patients with preserved hearing, the rate of anatomic preservation of the cochlear nerve was 84%. The overall rate of functional hearing preservation was 51%.
General Considerations
None of the methods for measuring auditory function during surgery should be affected by the use of routine anesthetics, including NMB. Patient factors, such as core temperature, muscle activity, blood pressure, and oxygenation, may alter electrophysiologic responses, however. Small decreases in core body temperature to 34.5° C result in delays of ABR waves.23 Similarly, increased muscle activity (e.g., fasciculations in a nonparalyzed patient) or hypotension and hypoxia may interfere with accurate measurements.
Auditory Evoked Brainstem Response
ABR is considered a far-field response because it represents activity measured between scalp electrodes that are placed at relatively large distances from CN VIII and brainstem generator sites. Brainstem auditory evoked potentials consist of five to seven peaks representing electric activity of auditory nerve, nuclei, and fiber tracts of the ascending auditory pathways. Peak I refers to the distal portion of the auditory nerve. Peak II represents the central portion of the auditory nerve. Peak III represents the cochlear nucleus. Peak V represents the termination of the lateral lemniscus in the inferior colliculus. Waves III, IV, and V are created by multiple generators. Intraoperative ABR monitoring setup is similar to the setup for routine office measurement (Fig. 64-3). Subdermal needle electrodes are used for recording brainstem auditory evoked potentials. Insert ear plugs are suitable for delivering sound for the recording of auditory evoked potentials. Recorded potentials should be compared with baseline recording before the beginning of the operation. To reduce the time to obtain interpretable evoked potentials, the following actions are recommended:
Interpretation of Results
Much depends on obtaining an optimal preoperative baseline response before entering the operating room and again after induction of anesthesia before initiation of the procedure. Criteria for judging that a change has occurred include increases in absolute latency of wave V from 0.5 to 2 ms.23 The strongest change is the loss of a repeatable wave V after all potential sources of interference have been ruled out. Harper and Slavit24 used criteria of greater than a 0.1 ms increase in latency or greater than a 50% decrease in amplitude to identify a change in the response.
Electrocochleography
Interpretation of Results
As with ABR, an adequate preoperative baseline response must be obtained against which to judge changes observed during the procedure. ECoG has been used to ascertain whether the goal of decompression operation of the endolymphatic shunt has been achieved. It has been thought that summating potential potentials normalize when pressure imbalances of the cochlea have been eliminated. Zappia and colleagues25 suggested that latency changes of greater than 1 ms and an amplitude decrease of greater than 50% should be considered significant changes in the response, although any measurable deviation should be reported immediately to the surgeon. Significant changes in the action potential indicate either direct or ischemic cochlear injury. After interruption of cochlear blood flow, 20 seconds or more may elapse before changes in ECoG response can be detected. This delay presumably occurs as a result of metabolic reserves that sustain cochlear function until their depletion from prolonged ischemia causes failure of electrophysiologic activity.
Direct Eighth Cranial Nerve Recording
The response waveforms obtained from direct recordings of the cochlear nerve typically exhibit triphasic patterns.26 The initial positive deflection (downward) represents nerve activity approaching the recording site. The generally larger negative (upward) deflection occurs as the impulse passes under the electrode. As the depolarization moves away (more centrally) from the recording electrode, another positive wave results and completes the triphasic complex. In the case of CN VIII monitoring, baseline recordings are obtained after craniotomy, but before tumor dissection.
Otoacoustic Emissions
Distortion-product OAE have been shown to be very sensitive to hypoxia and interruption of inner ear blood flow. Being sound waves, OAE are described by amplitude, frequency, and phase measures. Amplitude and phase are affected by manipulations that alter cochlear (and presumably outer hair cell) function. Subtle early changes in amplitude were measurable in some subjects within the first 10 seconds after internal auditory artery (IAA) occlusion. More recently, phase changes have been noted within 2 to 3 seconds of IAA compression, suggesting that phase measures may be the most sensitive OAE parameter for monitoring cochlear function during surgery.27
Emissions were stable during various procedures over many hours. Inhalation and intravenous (narcotic) anesthetics did not seem to affect the recording of emissions. Acoustic noise has the potential to undermine the recording of OAE. This effect was particularly prominent when measuring emissions at frequencies less than 2 kHz.28 Based on numerous recordings from various operating rooms, most background acoustic noise energy seemed consistently concentrated at less than 2 kHz. The authors recommended choosing patients for surgical OAE monitoring with preoperatively intact hearing and emissions greater than 2 kHz.28
Some of the noise can be eliminated from the ear canal by sealing the probe and the meatus. A surgical scrub sponge has been used with some success.29 Silicone, wax, and other substances also have been employed in an attempt to solve this problem. Distortion-product OAE have been monitored during loud suctioning of cerebrospinal fluid (relatively high frequency) from the operative field. Emissions could not be monitored during drilling (large-amplitude noise of low and high frequency). Mechanical interference with the conductive hearing mechanism would affect measurement of OAE. Fluid or debris such as bone dust in the external auditory canal or middle ear would interfere with emissions. For this reason, OAE monitoring is unsuitable for many transmastoid surgical approaches.
Transient-evoked OAE result in an amplitude-weighted frequency spectrum that may be repeated throughout the critical portion of the operation.28 Generally, reliable transient-evoked OAE can be obtained in 30 to 40 seconds in ideal conditions and longer intervals in noisy situations.
Because OAE reflect only cochlear function. ABR or direct CN VIII techniques would be appropriate to combine with emissions monitoring. Distortion-product OAE and ABR have been measured during vestibular schwannoma surgery using the same acoustic probe (ER 10-B, Etymotic Research Corp., Elk Grove Village, IL), which contained two speakers and a microphone.29 Rapid switching between the two recording instruments allowed comparisons of the responses. The probe should be secured in the ear canal at the level of the meatus. The pinna, probe, and tubing may be prepared out of the field by folding and securing (with tape or suture) the pinna anteriorly. The tubing should be secured to the patient or operating table in a location to prevent pulling or other disturbance. Baseline measurements obtained after probe insertion and after sterile draping of the operative field help ensure successful monitoring.
Laser-Doppler Cochlear Blood Flow
Cochlear blood flow can be measured using laser Doppler flowmetry.30 The technique involves positioning the end of a needle probe, housing emitter and collector optical fibers, against the cochlear promontory in the middle ear. The angle of the probe is adjusted to obtain maximal flow readings. Although the potential application of this technology is exciting, transferring laser Doppler flow measurement techniques developed in the animal model to human surgery is being done in research trials and is to be studied further before it can be practically applied during tumor removal operations.
Monitoring Other Cranial Nerves
Nerves are localized using the same technique as used for the facial nerve electrode, employing subdermal needle electrodes placed with great care. Subdermal needle electrodes can be placed percutaneously in extraocular muscles that are innervated by a respective cranial nerve. Great care is needed to prevent injury to the globe. It is important to secure the electrodes well so that the location of the needle does not change when the patient is repositioned. The opposite forehead serves as a good location for reference electrode to avoid contamination with EMG potentials from ipsilateral facial muscles.31
EMG from soft palate (CN IX),32 false vocal cords or laryngeal muscles (CN X),33 sternocleidomastoid or trapezius muscle (CN XI), or lateral tongue (CN XII) can be used for the lower cranial nerves. CN XII monitoring can be done by placing recording electrodes spaced 1 cm apart in the lateral tongue.34 Each EMG potential is monitored on separate channels and clearly labeled so that the oscilloscope loudspeaker can be set to monitor the channel of importance at a particular time in a case.
CONCLUSION
Intraoperative monitoring of CN VIII has become the standard of care for skull base and CPA surgery. Cost-effectiveness analysis supports facial nerve monitoring in mastoid and middle ear surgery.35 Innovative methods of cranial nerve monitoring using techniques such as intraoperative F wave20 and transcranial electric motor evoked potential measurement36,37 are being investigated, but have yet to be widely adopted in otolaryngology. The latter technique is used in neurosurgery and vascular surgery, and provides the advantage of not relying on irritating stimulus of the nerve, but rather stimulation of the cerebral cortex. Their use may be limited by the requirement for only intravenous anesthetic agents because of suppression of the signal when inhalational agents are used.
1. Krause F. Surgery of the Brain and Spinal Cord. New York: Rebman; 1912.
2. Hilger J.A. Facial nerve stimulator. Trans Am Acad Ophthalmol Otolaryngol. 1964;68:74-76.
3. Delgado T.E., Buchheit W.A., Rosenholtz H.R., Chrissian S. Intraoperative monitoring of facial muscle evoked responses obtained by intracranial stimulation of the facial nerve: A more accurate technique for facial nerve dissection. Neurosurgery. 1979;4:418-421.
4. Moller AR, Jannetta PJ: Preservation of facial function during removal of acoustic neuromas: Use of monopolar constant-voltage stimulation and EMG. J Neurosurg 61:757-760, 2984.
5. Pratt R.L. Iatrogenic facial nerve injury: The role of facial nerve monitoring. Otolaryngol Clin North Am. 1996;29:265-275.
6. Hughes G., Bottomy M., Dickins J., et al. A comparative study of neuropathologic changes following pulsed and direct current stimulation of the mouse sciatic nerve. Am J Otolaryngol. 1980;1:378-384.
7. Hughes G.B., Bottomy M.B., Jackson C.G., et al. Myelin and axon degeneration following direct current peripheral nerve stimulation: A prospective controlled experimental study. Otolaryngol Head Neck Surg. 1981;89:767-775.
8. Chase S.G., Hughes G.B., Dudley A.W.Jr. Neuropathologic changes following direct-current stimulation of the rat sciatic nerve. Otolaryngol Head Neck Surg. 1984;92:615-617.
9. Choung Y.H., Park K., Cho M.J., et al. Systematic facial nerve monitoring in middle ear and mastoid surgeries: “Surgical dehiscence” and “electrical dehiscence. Otolaryngol Head Neck Surg. 2006;135:872-876.
10. Nakao Y., Piccirillo E., Falcioni M., et al. Electromyographic evaluation of facial nerve damage in acoustic neuroma surgery. Otol Neurotol. 2001;22:554-557.
11. Prell J., Rampp S., Romstock J., et al. Train time as a quantitative electromyographic parameter for facial nerve function in patients undergoing surgery for vestibular schwannoma. J Neurosurg. 2007;106:826-832.
12. Kizilay A., Aladag I., Cokkeser Y., et al. Effects of partial neuromuscular blockade on facial nerve monitorization in otologic surgery. Acta Otolaryngol. 2003;123:321-324.
13. Blair E.A., Teeple E.Jr., Sutherland R.M., et al. Effect of neuromuscular blockade on facial nerve monitoring. Am J Otol. 1994;15:161-167.
14. Lacombe H., Keravel Y., Eshraghi A.A. Interest of monitoring of facial nerve on facial function in translabyrinthine surgery of acoustic neuroma. Ann Otolaryngol. Chir Cervicofac. 1994;111:89-93.
15. Selesnick S.H., Carew J.F., Victor J.D., et al. Predictive value of facial nerve electrophysiologic stimulation thresholds in cerebellopontine-angle surgery. Laryngoscope. 1996;106:633-638.
16. Grayeli A.B., Guindi S., Kalamarides M., et al. Four-channel electromyography of the facial nerve in vestibular schwannoma surgery: Sensitivity and prognostic value for short-term facial function outcome. Otol Neurotol. 2005;26:114-120.
17. Neff B.A., Ting J., Dickinson S.L., Welling D.B. Facial nerve monitoring parameters as a predictor of postoperative facial nerve outcomes after vestibular schwannoma resection. Otol Neurotol. 2005;26:728-732.
18. Isaacson B., Kileny P.R., El-Kashlan H.K. Prediction of long-term facial nerve outcomes with intraoperative nerve monitoring. Otol Neurotol. 2005;26:270-273.
19. Selesnick S.H. Optimal stimulus duration for intraoperative facial nerve monitoring. Laryngoscope. 1999;109:1376-1385.
20. Wedekind C., Klug N. Facial F wave recording: A novel and effective technique for extra- and intraoperative diagnosis of facial nerve function in acoustic tumor disease. Otolaryngol Head Neck Surg. 2003;129:114-120.
21. Arriaga M., Haid R., Masel D. Antidromic stimulation of the greater superficial petrosal nerve in middle fossa surgery. Laryngoscope. 1995;105:102-105.
22. Levine R.A., Ojemano R.G., Montgomery W.V., McGaffigan P.M. Monitoring auditory evoked potentials during acoustic neuroma surgery: Insights into the mechanism of the hearing loss. Ann Otol Rhinol Laryngol. 1984;93:116-123.
23. Samii M., Gerganov V., Samii A. Improved preservation of hearing and facial nerve function in vestibular schwannoma surgery via the retrosigmoid approach in a series of 200 patients. J Neurosurg. 2006;105:527-535.
24. Harper C.M., Harner S.G., Slavit D.H. Effect of BAEP monitoring on hearing preservation during acoustic neuroma resection. Neurotology. 1992;42(8):1551-1553.
25. Zappia J.J., Wiet R.J., O’Connor C.A. Intraoperative monitoring in acoustic neuroma surgery. Otolaryngol Head Neck Surg. 1996;115:99-106.
26. Schwartz D.M., Bloom M.I., Dennis I.M. Perioperative monitoring of auditory brainstem responses. Hear J. 1985;38:9-13.
27. Telischi F.F., Stagner B.B., Widick M.P., et al. Distortion-product otoacoustic emission monitoring of cochlear blood flow. Laryngoscope. 1998;108:837-842.
28. Telischi F.F., Widick M.P., Lonsbury-Martin B.L., McCoy M.I. Monitoring cochlear function intraoperatively using distortion product otoacoustic emissions. Am J Otol. 1995;16:597-607.
29. Cane M.A., O’Donoghue U.M., Lutman M.E. The feasibility of using oto-acoustic emissions to monitor cochlear function during acoustic neuroma surgery. Scand Audiol. 1992;21:173-176.
30. Nakashima T., Naganawa S., Sone M., et al. Disorders of cochlear blood flow. Brain Res Brain Res Rev. 2003;43:17-28.
31. Sekiya T., Hatayama T., Iwabuchi T., Maeda S.H. A ring electrode to record extraocular muscle activities during skull base surgery. Acta Neurochir (Wien). 1992;117:66-69.
32. Yingling C.D. Intraoperative monitoring in skull base surgery. In: Jackler R.K., Brackmann D.E., editors. Neurotology. St Louis: Mosby–Year Book; 1994:967-1002.
33. Stechison M.T. Vagus nerve monitoring: A comparison of percutaneous versus vocal fold electrode recording. Am J Otol. 1995;16:703-706.
34. Moller A.R. Intraoperative monitoring of evoked potentials: An update. In: Wilkins R.H., Rengachary S.S., editors. Operative Technique, and Neuro-Oncology. New York: McGraw-Hill; 1990:169-176. Neurosurgery Update I: Diagnosis
35. Wilson L., Lin E., Lalwani A. Cost-effectiveness of intraoperative facial nerve monitoring in middle ear or mastoid surgery. Laryngoscope. 2003;113:1736-1745.
36. Akagami R., Dong C.C., Westerberg B.D. Localized transcranial electrical motor evoked potentials for monitoring cranial nerves in cranial base surgery. Neurosurgery. 2005;57(1 Suppl):78-85.
37. Liu B.Y., Tian Y.J., Liu W., et al. Intraoperative facial motor evoked potentials monitoring with transcranial electrical stimulation for preservation of facial nerve function in patients with large acoustic neuroma. Chin Med J. 2007;120:323-325.
38. Lin V.Y., Houlden D., Bethune A., et al. A novel method in predicting immediate postoperative facial nerve function post acoustic neuroma excision. Otol Neurotol. 2006;27:1017-1022.
39. Isaacson B., Kileny P.R., El-Kashlan H., Gadre A.K. Intraoperative monitoring and facial nerve outcomes after vestibular schwannoma resection. Otol Neurotol. 2003;24:812-817.
40. Fenton J.E., Chin R.Y., Fagan P.A., et al. Predictive factors of long-term facial nerve function after vestibular schwannoma surgery. Otol Neurotol. 2002;23:388-392.
41. Goldbrunner R.H., Schlake H.P., Milewski C., et al. Quantitative parameters of intraoperative electromyography predict facial nerve outcomes for vestibular schwannoma surgery. Neurosurgery. 2000;46:1140-1146.
42. Nissen A.J., Sikand A., Curto F.S., et al. Value of intraoperative threshold stimulus in predicting postoperative facial nerve function after acoustic tumor resection. Am J Otol. 1997;18:249-251.
43. Zeitouni A.G., Hammerschlag P.E., Cohen N.L. Prognostic significance of intraoperative facial nerve stimulus thresholds. Am J Otol. 1997;18:494-497.
44. Taha J.M., Tew J.M.Jr., Keith R.W. Proximal-to-distal facial amplitude ratios as predictors of facial nerve function after acoustic neuroma excision. J Neurosurg. 1995;83:994-998.
45. Lalwani A.K., Butt F.Y., Jackler R.K., et al. Facial nerve outcome after acoustic neuroma surgery: A study from the era of cranial nerve monitoring. Otolaryngol Head Neck Surg. 1994;111:561-570.
46. Prasad S., Hirsch B.E., Kamerer D.B., et al. Facial nerve function following cerebellopontine angle surgery: Prognostic value of intraoperative thresholds. Am J Otol. 1993;14:330-333.
47. Niparko J.K., Kileny P.R., Kemink J.L., et al. Neurophysiologic intraoperative monitoring, II: Facial nerve function. Am J Otol. 1989;10:55-61.