6 Intracardiac Echocardiography
Equipment and Handling Procedures
Guiding Device Closure of Interatrial Communications
Monitoring of Percutaneous Left Atrial Appendage Closure
Guiding Radiofrequency Pulmonary Vein Ablation
Monitoring Transcatheter Aortic Valve Implantation
Perioperative and Periinterventional Imaging of the Ascending Aorta
Periinterventional Imaging of the Descending Thoracic Aorta
During the past decade, intracardiac echocardiography (ICE) has become a standard guiding approach in interventional treatment of structural heart disease,1 such as in device closure of interatrial communications, percutaneous transluminal septal myocardial ablation, pulmonary vein ablation, percutaneous left atrial appendage closure, and recently in transcatheter aortic valve implantation (TAVI). With the introduction of the 8F AcuNav catheter (Acuson Siemens, Mountain View, Calif.), which is also used for ICE,2 intraaortic phased-array imaging (IPAI) became feasible. Guidance by fluoroscopy alone is limited because it cannot distinguish soft tissues and does not allow cross-sectional imaging. Consequently accurate positioning of devices can be difficult without echocardiographic guidance.2a On the other hand, distant posterior areas are difficult to depict with transthoracic echocardiography, especially when the patient is supine. Although transesophageal echocardiography (TEE) including real-time three-dimensional (3D) imaging is a well established diagnostic tool and provides exceptional high-resolution images, TEE’s usefulness for interventional procedures is not always ideal (see Chapter 5). However, evidence that ICE guidance can improve the safety of these procedures is still lacking.
Miniaturized ultrasound-tipped catheter devices were primarily introduced for intravascular use. The first attempts to percutaneously introduce intravenous probes with built-in echo transducers for in vivo intracardiac imaging were reported in the late 1960s. During the following two decades, several intracardiac echocardiographic catheters were developed. Later, intravascular ultrasound (IVUS) was employed to image coronary arteries as well as the aorta and peripheral vessels (see Chapter 7). IVUS-based intracardiac imaging was also used for guiding electrophysiologic procedures. Nevertheless, intracardiac IVUS lacks Doppler capabilities and is further limited by inadequate ultrasound penetration. For current noncoronary percutaneous interventions in structural heart disease, high-quality near-field images as well as Doppler flow analysis are a prerequisite for optimal results and also aid in avoiding and detecting complications. Thus, technical advances led from IVUS to the development of ICE and IPAI. Using these methods as a guiding tool in noncoronary percutaneous interventions is justifiable on the basis of improved procedural success and reduced complications, although costs of the catheter and reimbursement are problematic in many countries. In particular, progress in electrophysiologic ablation is clearly linked to the advances made with ICE.3 ICE and IPAI are also exciting research tools. Transfer of the methodology from the realm of research to routine clinical use is ongoing, such as in TAVI.4
Equipment and Handling Procedures
Current devices (Acuson, Mountain View, Calif.) are multimodal, phased-array transducer-tipped intracardiac echocardiographic catheters. Nowadays, the 8 F AcuNav catheter has become the tool of choice for ICE. Other devices have been introduced5 but have gained only limited acceptance (Table 6-1).
TABLE 6-1 Presently Available Intracardiac Echocardiographic Devices
Catheters | Company | Features |
---|---|---|
UltraICE | Boston Scientific | Rotational, nonsteerable |
EP Med View Flex | St. Jude Medical | Side-looking, 10 Fr, 2-8 MHz |
ClearICE | St. Jude Medical | Side-looking, steerable, 3D localization |
AcuNav | Siemens/Biosense-Webster | Side looking, steerable, 8 Fr and 10 Fr |
SoundStar | Siemens/Biosense-Webster | Side-looking, steerable, 10 Fr, 3D localization |
In comparison to the 10-Fr version, the 8-Fr AcuNav catheter has facilitated ICE and increased patient comfort and probably safety as well. After its introduction in pediatric cardiology,2 the 8-Fr ICE catheter now is also used in adult interventional cardiology. The catheter possesses the unlimited echocardiographic capabilities of its predecessor but is available with more insertable length and in a shorter version. Besides two-dimensional (2D) and M-mode imaging, the ICE catheter also permits functional analysis. It possesses complete Doppler capabilities, including pulsed wave, continuous wave, color flow, and tissue Doppler modalities (Table 6-2).
TABLE 6-2 Doppler Imaging Modes and Frequencies of the Intracardiac Echocardiographic Catheter Family
Catheter versions | 10 F | 8 F |
Insertable length | 90 cm | 90 cm (formerly 110 cm) |
Steering | Four-way | Four-way |
2D imaging frequency | 5.0-10.0 MHz | 5.0-10.0 MHz |
Color Doppler frequency | 4.0-7.0 MHz | 4.0-7.0 MHz |
CW Doppler | 5.0 MHz | 5.0-5.2 MHz |
PW Doppler | 4.0-5.0 MHz | 4.0-5.0 MHz |
Recommended access sheaths | 11 Fr | 8 or 9 Fr |
Penetration | 15 cm | 15 cm |
PW, Pulsed wave.
Practical Tips
• Use long access sheaths to avoid injuring the pelvic veins during navigation.
• Use fluoroscopy as a precaution to safely advance the bidirectionally steerable (but guidewireless) catheter into the heart.
• Infuse 500 mL saline solution to dilate the veins and to facilitate catheter passage. Added benefit: reduced tendency for vagal responses when both the left and the right femoral veins need to be punctured.
To permit adequate imaging of the interatrial septum and its neighboring structures, two standardized views are used: (1) a transatrial longitudinal view showing the extent of the atrial septum from cranial to its distal margins—this view is seen with the catheter retroflexed inside the RA; and (2) a perpendicular transatrial short-axis view for visualizing the anterior part of the atrial septum and the transition to the aortic valve and the ascending aorta (Fig. 6-1).
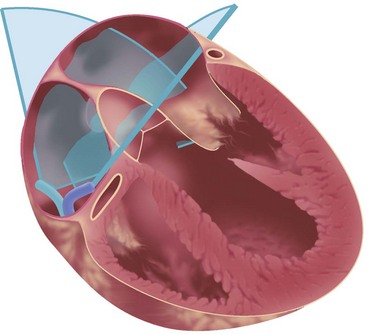
Figure 6-1 Standard transatrial views for intracardiac imaging are obtained with the catheter tip in the RA.
Clockwise rotation of the straightened ICE catheter permits visualization of the smallest anatomic details in the near field as well as to a depth of up to 12 cm. In order to enter the right ventricle, the tip of the probe is positioned in the mid to upper RA with the piezoelectric crystal facing the free wall of the RA, before the probe is gradually deflected anteriorly. From the right ventricle, the transventricular long-axis view of the left ventricle (LV) is obtained, which shows the interventricular septum proximally, the LV outflow tract, and the mitral valve apparatus. When the catheter tip is tilted to the right, the transventricular short-axis view of the LV comes into view (Figs. 6-2 and 6-3).
Interpretation of LV wall motion from transventricular views requires care because the catheter is moving inside the RV. By tilting the catheter tip away from the transventricular long-axis view, the RV outflow tract and pulmonary valve can be visualized as well (Fig. 6-4).
To avoid ventricular arrhythmias, the catheter has to be carefully navigated inside the RV cavity. The catheter should not be advanced beyond the pulmonary valve. When using the SVC approach, inadvertent catheter passage into the coronary sinus has to be avoided by all means. A certain expertise in intracardiac catheter manipulation is essential to safely advance the catheter into the right heart, to orient oneself inside the heart, to obtain standardized views, and to adequately visualize the cardiac anatomy6 (Table 6-3; also see Fig. 6-1).
Window | Catheter Position | Standardized Cut Plane |
---|---|---|
Transatrial | RA | Longitudinal, craniocaudal view of IAS, LA, LAA |
RA | Short-axis view of the anterior IAS, aortic valve, and ascending aorta | |
RA | Longitudinal view of the RV, showing TV, RV | |
Transventricular | RV | Long-axis view of the LV, IVS, LVOT, LA including LAA |
RV | Short-axis view of the LV | |
Transvenous | IVC | Aortic view of the abdominal aorta and its side branches |
SVC/RA | Aortic view of the ascending aorta and aortic valve | |
Intraaortic | Aorta | Imaging from inside the whole aorta including aortic arch |
Aorta | Long-axis view of the aortic valve seen from the aortic arch |
IAS, Interatrial septum; IVC, inferior vena cava; IVS, interventricular septum; LAA, left atrial appendage; LVOT, left ventricular outflow tract; TV, tricuspid valve.
Guiding Device Closure of Interatrial Communications
Device closure of interatrial communications is performed for treatment of severe left-to-right shunts associated with atrial septal defects (ASDs) (see Chapter 44) and for prevention of recurrent paradoxical embolism in patients with a patent foramen ovale (see Chapter 41). Some complications with closure of interatrial communications are due to suboptimal device performance.7 Others, however, may be related to discontinuous echocardiographic monitoring, because supine patients do not tolerate continuous monitoring with TEE well unless they are sedated or under general anesthesia. More than 10 years of experience make us believe that some specific complications of transcatheter closure can potentially be avoided with improved echocardiographic monitoring. In that respect, ICE can be recommended as the method of choice for guiding percutaneous device closure, especially of ASDs.
Before passing instrumentation through the interatrial communication, the ICE catheter is advanced through the inferior vena cava into the RA. The transducer is aimed at the left atrium (LA) to obtain the transatrial longitudinal view. As a first step, adequate position of a long guidewire in the left superior pulmonary vein is demonstrated. The left superior pulmonary veins are depicted by angulating the probe from the longitudinal view inferiorly. The stretched size of ASDs can be adequately measured by ICE; however, sizing balloons must still be used—a mandatory step for estimating the size of the communication before ASD device closure.8
Practical Tips
• ICE displays release of the left-sided counteroccluder opening in the LA.
• The whole system including the long access sheath with the delivery cable inside and the opened left-sided counteroccluder is slightly pulled back until the open counteroccluder applies some traction on the atrial septum. ICE depicts the moment when the left-sided counteroccluder is closely aligned with the atrial septum.
• With the delivery cable still under traction, the long access sheath is further withdrawn to release the right-sided counteroccluder. ICE demonstrates release of the right-sided counteroccluder inside the RA and the left-sided counteroccluder remaining on the left side of the septum.
• While monitoring the opening of the right-sided counteroccluder, the delivery cable is carefully pushed forward until the counteroccluder also fits tightly into the atrial septum, but from the right side. Viewing two perpendicular cut planes (transatrial longitudinal and short-axis views) is mandatory to confirm the result and to rule out jamming of one of the counteroccluders in the interatrial communication (Fig. 6-5).