CHAPTER 76 Injuries of the Upper Cervical Spine
Demographics
Traumatic injuries to the upper cervical spine are most often encountered in children and in people older than age 60 years. Fortunately, they are still rare occurrences in children. Motor vehicle accidents are the cause of pediatric cervical spine trauma in about 38% of cases.1–3 Cervical spine injuries in children account for 1% to 9% of all reported pediatric spinal trauma.4,5 Upper cervical spine injuries are responsible for the majority of these, accounting for 56% to 73% of all cervical spine injuries in children.2,6 Head injury occurs in conjunction with cervical spine injuries in up to 53% of these cases. When these two entities are concurrent, the overall mortality is very high (41%).7
Upper cervical spine injuries constitute a large proportion of cervical spine trauma in people older than age 60 years. Injuries to the C1-C2 complex account for up to 69.8% of all cervical spine trauma in those older than the age of 60.8 Odontoid fractures alone can constitute up to 57% of all cervical spine injuries in this age group. Unlike the pediatric and young adult populations, upper cervical spine trauma in the elderly often occurs from minor trauma. Falls are often the predominant cause of injury. Many explanations for this phenomenon include the presence of weakened bone at the CCJ owing to osteoporosis/osteopenia, higher stresses on the upper cervical spine owing to spondylosis of the lower cervical spine, and an increased propensity to fall from deterioration in locomotor response and balance control due to age and cervical spinal stenosis. The lower energy of the trauma is directly correlated to the lower rate of neurologic injury seen in this age group after injury as compared with the pediatric and young adult populations. However, when neurologic deficits occur, they can have devastating consequences. A 26% to 28% mortality for upper cervical level spinal cord injury has been reported for this population, with a dismal 59% survival at 2 years.9–11
In the young adult population, the majority of patients with spinal column injury are young males (up to 30% are males in their 30s).12 The most common mechanism in this age group is from vehicular accidents, followed by falls, gunshot injuries, and sports injuries. Most of the cervical spine trauma in this age group occurs in the subaxial spine and is often associated with a high-energy mechanism, severe head injury, or a focal neurologic deficit.13 Trauma to the CCJ in this population is often associated with severe neurologic injury and head trauma with relatively high rates of mortality and morbidity.
Anatomy of the Upper Cervical Spine (Craniocervical Junction)
The upper cervical spine is a complex three-unit joint that includes the bones of the occiput, atlas, and axis, their synovial articulations, and the associated ligamentous structures. The six synovial joints in this complex include the paired occipitoatlantal joints, the anterior and posterior median atlantoodontoid joints, and the paired atlantoaxial joints. These joints allow for a significant amount of motion at the CCJ. The occiput-C1 articulation supplies approximately 50% of total cervical flexion and extension, and the C1-C2 articulations supply 50% of total cervical rotation.14 Corresponding to this, the majority of the mechanical stability at the CCJ is provided by the investing ligamentous structures. An understanding of the anatomy of the CCJ is necessary to appreciate the spectrum of injuries that occur in the upper cervical spine and the strategies that have been devised to treat them. The specific articulations, ligamentous restraints, and neurovascular structures at risk for injury are addressed in a systematic fashion.
The occiput articulates with the atlas through paired occipitoatlantal joints. The occipital condyle is oval and sloped inferiorly from lateral to medial in the coronal plane, making a 25- to 28-degree angle with the midsagittal plane. The convex occipital condyles articulate with the concave superior articular facets of C1 in a “cup-and-saucer”–type fashion. In the coronal plane, the joint slopes medially toward the foramen magnum. The shape of the occipitoatlantal joint allows significant flexion and extension and some lateral bending but minimal axial rotation. Flexion is limited by the bony impingement of the anterior portion of the foramen magnum on the odontoid process, and extension is limited by the posterior arch of the atlas impinging on the posterior aspect of the skull. The anterior atlanto-odontoid joint lies between the anterior arch of the atlas and the anterior aspect of the dens. The posterior atlanto-odontoid articulation lies between the posterior aspect of the dens and the anterior portion of the transverse ligament. The paired atlantoaxial joints are situated between the inferior articular facets of the atlas and the superior articular facets of the axis. These joints are fairly shallow to allow for a significant amount of motion at the CCJ. The ligamentous restraints provide the necessary stability to prevent injury to the enclosed brainstem and spinal cord (Fig. 76–1).
The anterior longitudinal ligament attaches to the anterior body of the axis, anterior arch of the atlas, and anteroinferior edge of the foramen magnum. The cruciform ligament is composed of vertical and transverse portions. The vertical portion attaches to the anterior edge of the foramen magnum and the posterior aspect of the body of the axis. The transverse component of the cruciform ligament is commonly referred to as the transverse ligament. This important structure is made entirely of relatively nonelastic collagen fibers and extends between the osseous tubercles on the medial aspects of the lateral masses of the atlas. The tectorial membrane is the broad cephalic extension of the posterior longitudinal ligament and runs from the posterior surface of the body and dens of the axis to the anterolateral edge of the foramen magnum. This structure is a primary stabilizer of the occipitoatlantal articulation15 and helps to limit extension at this joint. The nuchal ligament extends from the posterior border of the occiput to the spinous processes of the cervical vertebrae to C7 and the intervening interspinous ligaments. The anterior occipitoatlantal membrane, part of the anterior longitudinal ligament, extends from the cephalad portion of the anterior arch of the atlas to the anterior edge of the foramen magnum. The atlanto-odontoid ligament runs from the anterior surface of the odontoid process and the caudal portion of the anterior arch of the atlas. The apical ligament of the dens lies between the vertical band of the cruciform ligament and the anterior occipitoatlantal ligament. This structure connects the apex of the dens with the anterior edge of the foramen magnum. The alar ligaments are paired structures that arise from the dorsolateral aspect of the dens and run obliquely to connect with the inferomedial aspect of the occipital condyles and the lateral masses of the atlas. These ligaments are important stabilizers of the occipitoatlantal joint and limit axial rotation and lateral bending.15 Like the transverse ligament, the alar ligaments are also made entirely of collagen fibers, and failure occurs at 10% stretch.16 These ligaments are most vulnerable in whiplash-type injuries.17 The posterior occipitoatlantal membrane attaches to the posterior margin of the foramen magnum and the posterior arch of the atlas. The posterior atlantoaxial membrane runs between the posterior arches of the atlas and the axis.
The vertebral artery and the internal carotid artery lie in close proximity to the osseous structures of the CCJ. Within the atlas and the axis the paired vertebral arteries typically lie in the foramen transversarium. At this level the paired vertebral arteries are susceptible to injury by shearing forces with rotation and flexion or extension. At the upper atlantal surface, the artery curves posteriorly into a transverse groove in the atlas behind the superior atlantal articular facet. In patients with congenital bony anomalies of the upper cervical spine, there is a higher incidence of vertebral artery anomalies.18 The artery then enters the subarachnoid space by piercing the posterior occipitoatlantal membrane and dura mater just medial to the occipital condyle. The internal carotid artery is adjacent to the anterior surface of the lateral mass of C1. This position renders it susceptible to injury by a forward thrust of the lateral mass as the head is forcefully rotated to the contralateral side.19
Clinical Evaluation
The treatment of a patient with a cervical spine injury regardless of location is initiated at the scene of the injury. Without exception, all victims of trauma are suspected to have a cervical injury until proven otherwise. Cervical spine injury has been closely linked to the presence of severe head injury (odds ratio 8.5), a high-energy mechanism (odds ratio 11.6), or a focal neurologic deficit (odds ratio 58).13 In suspected injuries of the upper cervical spine, an adequate airway and ventilation must be established because upper spinal cord injury can lead to diaphragmatic and intercostal paralysis with respiratory failure. In addition, large retropharyngeal hematomas can cause upper airway obstruction. Nasotracheal intubation or cricothyroidotomy is safest in the acute setting because it causes less cervical spine motion than direct oral intubation techniques.20,21 However, improvements in fiberoptic imaging have resulted in newer technologies such as the Glidescope. This videolaryngoscope allows oral-tracheal intubation without the need for significant neck manipulation and is technically easier than the traditional method of fiberoptic-assisted intubation. Once the patient’s airway, breathing, and circulation are stabilized, initial stabilization of the cervical spine begins with the application of a rigid cervical collar, a spine board, and sandbags.
In general, noncontiguous spinal injuries can occur in 6% of patients and these fractures can be easily missed in the presence of head injury, upper cervical injury, or cervicothoracic injury. Atlas fractures, specifically, are associated with up to a 50% incidence of concurrent cervical spine fractures.22 Facial and head injuries are also commonly seen in conjunction with fractures and ligamentous disruptions of the CCJ. Specifically, upper cervical injuries are also more frequently seen in patients with trauma to the lower third of the face.23 In addition, up to 50% of patients with cervical spine injuries, spinal cord injuries, or both have associated head trauma. Brain damage is more associated with upper cervical injuries than with injuries to the subaxial spine.24 Subarachnoid hemorrhages, subdural hemorrhages, and cerebral contusions must be diagnosed and treated expeditiously because they are the most common cause of mortality in these patients.
In addition to the spinal trauma, other injuries should be assessed because they may influence the treatment of the spinal lesion and also significantly affect the outcome of the patient. In cervical spine trauma, much attention has been paid to the evaluation of these patients for vertebral artery injury. Friedman and colleagues25 reported a 24% overall incidence of vertebral artery injury in 37 cases of nonpenetrating cervical spine trauma. Vaccaro and colleagues26 noted a 19.7% incidence of vertebral artery injury found by magnetic resonance angiography in 61 patients. In Cothren and colleagues’27 series, 18% of 69 patients with vertebral artery injury and cervical spine trauma sustained injuries to the CCJ. The incidence of vertebral artery injury increases if the fracture extends into the foramen transversarium.28 Bilateral or dominant vertebral artery injury can cause fatal ischemic damage to the brainstem and cerebellum.29 Delayed cortical blindness and recurrent quadriparesis can also occur from occult vertebral artery injury after cervical trauma.30 Despite the high incidence of vertebral artery injury with cervical trauma, as well as the potential morbidity and mortality associated with vertebral artery injury, the great majority of these injuries are clinically silent.
Neurologic evaluation of injuries to the CCJ can be difficult because there is no specific myotomal or dermatomal distribution of motor and sensory loss, and it is further confounded by the frequent coexistence of facial and head trauma. Injuries to the C1 and C2 roots generally result in sensory deficits to the occiput and posterior scalp. A complete spinal cord injury at this level can result in ventilator-dependent quadriplegia. Incomplete spinal cord injury syndromes can also occur. At the occipitocervical junction, a peculiar syndrome of incomplete paralysis can develop as a result of compression/injury of the pyramidal decussation on the anterior aspect of the brainstem where the corticospinal tracts cross from one side to the other. The tracts to the arms cross cephalad to the tracts to the legs. If the primary injury is to the upper decussation, the arms can be more affected and give the appearance of a central cord syndrome. Caudal injury will affect the legs more than the arms. It is even possible to affect crossed arm fibers and uncrossed leg fibers, the so-called cruciate paralysis as described by Bell.31 These patients can also have large variations in heart rate, blood pressure, and respiratory rate owing to injuries to the cardiovascular and respiratory centers in the brainstem.32 Dysfunction of the lower cranial nerves (CN IX, X, XI, XII) is often seen with severe injuries to the occipitoatlantal joint and the skull base.
Imaging
Plain radiography is used as the first imaging modality for the upper cervical spine. The standard series includes anteroposterior, lateral, and open-mouth views. In general, about 85% of all significant injuries to the cervical spine will be detected on the lateral view of the cervical spine. In the upper cervical spine, the lateral view and the open-mouth view are the most useful. Flexion and extension views will be inadequate to assess for ligamentous injury owing to voluntary guarding in 33% of cases.33
Computed tomography (CT) remains the most sensitive imaging modality to evaluate fractures of the upper cervical spine, subaxial spine, and cervicothoracic junction. In a prospective study of polytrauma patients, CT used as a primary screening tool had a sensitivity of 84% in detecting upper cervical injury.13 CT is also cost effective as a primary screening tool, especially in high- and moderate-risk patients.34 With the added benefit of sagittal and coronal reconstructed images, CT has immense power to demonstrate complex fracture patterns not easily seen on standard radiography and on the axial images, especially at the occipitocervical junction.35
Magnetic resonance imaging (MRI) is not as good as CT or plain radiographs in the identification and evaluation of cervical fractures. Klein and colleagues36 showed that MRI had only 11.5% sensitivity for posterior fractures and 36.7% sensitivity for anterior fractures. Katzberg and colleagues12 reported that for acute fractures MRI had a weighted average sensitivity of 43%, compared with 48% for conventional radiography. Vaccaro and colleagues37 also noted that MRI is not cost effective as a screening device in patients without a neurologic deficit.
Despite its inadequacies in evaluating bony detail, MRI is unsurpassed for the assessment of the soft tissue elements in the cervical spine. These structures include the intervertebral disc, ligamentous structures, and the spinal cord itself.12 MRI is much more sensitive and specific than plain radiographs for the evaluation of a prevertebral hematoma. MRI is also useful for the detection of spinal cord hemorrhage, which, if present, carries a poor prognosis for neurologic recovery.38 Acute hemorrhage has a low signal intensity on T2-weighted images (secondary to intracellular deoxyhemoglobin) and becomes hyperintense over the next several days after it becomes converted to extracellular methemoglobin. MRI diffusion studies allow a more accurate assessment of the degree of spinal stenosis. These diffusion studies assess the flow of cerebral spinal fluid (CSF) around the spinal cord at an area of constriction. If the degree of spinal stenosis is severe, the CSF flow is significantly compromised.39
MRI neurography provides detailed visualization of individual nerve roots as they exit the brainstem, CCJ, and subaxial cervical spine. This MRI modality can help distinguish root level injuries from more peripheral injuries and double-crush–type nerve damage.40
Bedside fluoroscopic flexion and extension views have shown some diagnostic value in clearing the cervical spine in obtunded patients. In one report it was noted that 30% of these patients could not be adequately evaluated by this technique.41 Other researchers, however, have found this helpful, using a combination of initial in-line traction followed by flexion and extension views only if the traction views are normal.42 Due to the space constraints of most intensive care units and the lack of appropriate built-in shielding for the extensive use of fluoroscopic imaging, bedside fluoroscopy is relatively impractical in most hospitals. In addition, the use of both CT and MRI may provide sufficient information to allow removal of the cervical collar in an obtunded patient without the need for manipulation of the neck.
Specific Injuries to the Upper Cervical Spine
Occipital Condyle Fractures
Most injuries to the occipital condyles are caused by high-energy trauma to the head and neck. Bell reported the first case of occipital condylar fracture in 1817. The incidence of occipital condyle fractures is reported to range from 3% to 16%.43,44
Although radiographs may show some abnormal soft tissue swelling in the presence of an occipital condyle fracture, these injuries are often extremely difficult to detect with conventional radiography (Fig. 76–2).45
The most used classification system for occipital condyle injuries was described by Anderson and Montesano (Fig. 76–3).46 The injuries are categorized into three types according to morphology and mechanism of injury. Type I injuries are impaction fractures of the condyle from axial loading. These fractures tend to be comminuted. The tectorial membrane and the alar ligaments are usually intact. Unilateral type I lesions are stable, but bilateral lesions may be unstable. Type II injuries are part of a more extensive basioccipital fracture that involves one or both occipital condyles. The common mechanism of injury is a direct blow to the skull. The tectorial and alar ligaments are intact, and the fracture is usually stable. Type III injuries are avulsion fractures near the alar ligament insertion that result in medial displacement of the condylar fracture fragment from the inferomedial aspect of the occipital condyle into the foramen magnum. The mechanism of injury is a forced rotation of the head combined with lateral bending. Type III fractures are potentially unstable injuries owing to avulsion of the alar ligaments.
In contrast to the majority of publications, Maserati and colleagues47 reviewed 106 patients with occipital condyle fractures seen at a level one trauma center and found that only 3 required occipitocervical fusion. They suggested that each patient be evaluated looking for occipitocervical misalignment and that only those patients should be treated in a halo-vest or with a posterior fusion. The other 103 patients were successfully treated in a rigid cervical collar.47
Atlanto-Occipital Injuries
The incidence of injuries to the atlanto-occipital joint is estimated to be between 5% and 8% of fatal traffic injuries.48 These injuries account for 19% to 35% of all deaths from cervical spine trauma. More than 80% of cases of occiput-C1 dislocations were reported after 1975. Improvements in on-site resuscitation and emergency transportation have increased the number of patients who survive this catastrophic injury, which is typically the result of a motor vehicle accident. In a review of 146 traffic fatalities, Alker and colleagues49 found a 5% incidence of occipitoatlantal dislocations. Children younger than 12 years of age are uniquely predisposed to this injury because their occipitoatlantal joints are flatter and because their head weight–to–body weight ratio is significantly greater than in adults.
Radiographically, significant retropharyngeal soft tissue swelling at C3 will be seen (Fig. 76–4). Multiple anatomic lines mark the normal relationship of occiput to C1. A line drawn down the cranial aspect of the clivus should be tangential to the dens (Wackenheim line). Distance greater than 10 mm between the basion and the dens is considered abnormal.50 The sensitivity of this method is about 50%. An interval greater than 13 mm between the posterior mandible and the anterior atlas or 20 mm between the posterior mandible and the dens is abnormal (Fig. 76–5).51 The sensitivity of this method is 25%. Failure of a line drawn from the basion to the axis spinolaminar junction to intersect C2 or failure of a line from the opisthion to the posterior inferior corner of the body of the axis to intersect C1 is abnormal.52 The sensitivity of this method ranges from 20% to 75%. Powers’ ratio, the ratio of the distance from the basion to the posterior arch of the atlas divided by the distance from the opisthion to the anterior arch of the atlas, should be 1.0 or less in the absence of anterior occipitoatlantal dislocation (Fig. 76–6).53 Another method to diagnose occipitoatlantal subluxation or dislocation on plain radiographs was described by Harris and colleagues.54,55 They described a posterior axial line as the cranial extent of the posterior cortex of the axis body. If the distance between the basion and the posterior axial line (the basion-axial interval) is greater than 12 mm, or if the basion-dental interval is greater than 12 mm, then occipitocervical instability is present.54,55 The sensitivity of this method varies from 76% to 100%.
Because this is an unstable injury, flexion-extension views are not recommended. However, if they are available, there should be less than 1 mm of translation seen at the occipitoatlantal articulation. Instability is also present when there is distraction or marked asymmetry in the occipito-atlantal joints or when there are O-C1 level neurologic deficits present (Fig. 76−7). In children, more than 5-mm widening of the occipitoatlantal joints should raise the suspicion of this injury.
The most commonly employed classification system for occiput-C1 dislocations was described by Traynelis and colleagus56 (Fig. 76–8), who categorized these injuries into three types. In type I injuries there is anterior displacement of the occiput on the atlas. Type II injuries are the result of longitudinal distraction. Any traction applied to a type II injury can result in progression of the existing neurologic deficit. Type III injuries involve a posterior subluxation or dislocation. Very light traction of about 5 lb applied to type I and type III injuries will help to reduce the dislocation and may improve the neurologic deficit. Radiographs should be taken immediately to ensure that there is no overdistraction.
As noted earlier, the mortality from head-on-neck dislocation is extremely high. Only 20% of patients presenting to the trauma center with acute traumatic atlanto-occipital dislocation will have a normal neurologic examination.57 The remainder will suffer deficits of the cranial nerves, brainstem, and upper cervical spinal cord. Vertebral artery injury may accompany the dislocation. The most common mechanism is from an extension-rotation force. Patients with vertebral artery insufficiency at this level may exhibit Wallenberg syndrome, consisting of ipsilateral defects of cranial nerves V, IX, X, and XI; ipsilateral Horner syndrome; dysphagia; and cerebellar dysfunction.
All occipitocervical dislocations should be treated initially by immediate application of a halo vest. Because the majority of these injuries are unstable, posterior occipitocervical fusion is the procedure of choice (Fig. 76–9).32,58 This can be done using a variety of techniques including posterior wiring and structural grafting (Fig. 76–10), Ransford loop fixation with wiring (i.e., plate/rod and screw fixation with structural grafting) (Fig. 76–11). The first technique will require the use of postoperative halo immobilization, whereas the latter two techniques will usually only need collar immobilization as external support. Many instrumentation systems are currently available for occipitocervical fixation. Some use the thicker midline occipital bone and others rely on bilateral parasagittal occipital fixation.59–61 Also described is the use of polyaxial screws into the occipital condyles as the sole point of fixation to the skull and then connecting to standard C-1 and C-2 screws bilaterally.62,63
Fractures of the Atlas
Fractures of the atlas were first described by Jefferson in 1921. They usually occur in the anterior and posterior arches, which are the weakest points on the C1 ring. These injuries comprise 2% to 13% of all cervical spine fractures and approximately 25% of all injuries to the atlantoaxial complex.22 They are often seen in the younger age groups (mean age, 30 years) and are most commonly the result of vehicular accidents or a fall onto the top of the head.
These fractures are caused by axial loading. Because of this mechanism, these fractures commonly accompany head injuries in the polytrauma patient. In addition, there is an extremely high association (up to 50%) of atlas fractures with other cervical spine fractures. These include dens fractures, hangman’s fractures, teardrop fractures of C2, cervical burst fractures, and lateral mass fractures.22
Patients will often complain of severe suboccipital discomfort and a sense of instability. Neurologic injury is uncommon in the case of isolated fractures of the atlas, but, when it occurs, the greater occipital nerve is most frequently injured, followed by the lower cranial nerves.64
Retropharyngeal soft tissue swelling greater than 5 mm at C3 in combination with a fracture of the posterior arch of C1 is highly suggestive of a bursting-type injury. A combined lateral mass displacement on the open-mouth anteroposterior view exceeding 6.9 mm is indicative of transverse ligament insufficiency (Fig. 76–12),65 but this measurement may not be sensitive enough to detect all unstable injuries. MRI can be used to help assess the continuity of the transverse ligament in those cases in which the ligament status is unclear.66
Fine-cut CT in the plane of the axis will clearly delineate the fracture pattern. Common fracture patterns include isolated posterior arch fractures, lateral mass fractures, and burst fractures with combined anterior and posterior arch fractures. Isolated posterior arch fractures (Fig. 76–13) most commonly occur at the vertebral artery groove at the junction of the lateral mass and the posterior arch. These are stable fractures that can be treated in a cervical collar for comfort. Lateral mass fractures can be either displaced or nondisplaced. Nondisplaced injuries can be treated with a cervical collar. Fractures that are displaced more than 5 mm can be treated with immediate halo vest application. Burst fractures of the C1 ring are often referred to as Jefferson fractures, and these injuries have one or two fractures in the posterior arch and one or two fractures in the anterior arch. Minimally displaced fractures (<7 mm) can be treated in a cervical collar.67 Treatment of fractures with a combined diastasis of more than 6.9 mm consists of immobilization in a halo vest for 3 months (Fig. 76–14). After the halo vest is removed, flexion and extension radiographs are taken to test for C1-C2 instability, which, if significant, can be treated by C1-C2 posterior fusion.
Treatment of Jefferson fractures with greater than 6.9 mm of separation is controversial. Levine and Edwards advocate initial reduction of the fracture with skeletal traction for up to 6 to 8 weeks, followed by another 6 weeks of halo vest treatment.68 Other series have found that immediate treatment with a halo vest can result in an acceptable outcome and avoids the morbidity associated with prolonged bed rest.69,70 Traction reduction followed by early C1-C2 fusion using C1-C2 transarticular screws has also been described,71 but intraoperative difficulty may be found owing to gross instability of the C1 lateral masses and the loose C1 posterior arch.
Most surgeons would agree that operative treatment is indicated if more than 5 mm of C1-C2 instability exists on flexion and extension radiographs once the halo is removed. If the posterior arch of C1 is healed, a posterior C1-C2 fusion is indicated. In the case of nonunion of the posterior arch of C1 or significant injury to the occipital condyles, occiput to C2 fusion can be performed. Another indication for surgery is neck and/or occipital nerve pain in a patient with a nonunion of the atlas ring fractures. The recommended treatment would then be a posterior occiput-C2 fusion.72
Atlantoaxial Subluxation and Dislocation
Conservative care of atlantoaxial rotatory subluxation in children, also known as Grisel syndrome, is most effective when treatment is instituted early. Most children who have had symptoms for less than 1 week will improve with a soft cervical collar and close observation. When symptoms have been present for 2 to 4 weeks, hospital admission and head-halter traction is usually successful in achieving reduction. For patients who present 1 month after the subluxation occurs, skull traction can be instituted and continued for up to 3 weeks. Initial traction should be 7 lb and gradually increased to 15 lb. If traction fails to reduce the deformity, open reduction and C1-C2 fusion are recommended.73
Fielding and Hawkins74 presented the most commonly used classification scheme for these injuries (Fig. 76–15). Type I dislocations are pure rotational injuries. Type II injuries have both rotatory malalignment with anterior displacement of the atlas less than 3 to 5 mm, suggesting only a mild deficiency of the transverse ligament. Type III injuries combine rotatory subluxation with greater than 5 mm of displacement, suggesting complete deficiency of the transverse ligament. Type IV injuries have both rotational malalignment and posterior displacement.
Rupture of the Transverse Ligament
The transverse ligament is the major ligamentous stabilizing structure for the atlantoaxial articulation. It is composed primarily of collagen fibers, which render it stiff and inelastic.75 When torn or disrupted, it is incapable of repair and its original strength and function cannot be restored.
Insufficiency of the transverse ligament and subsequent C1-C2 instability is suspected if the atlantodens interval is greater than 3.5 mm in adults and greater than 5 mm in children on lateral radiographs and in atlas fractures when the combined overhang of the C1 lateral masses on C2 is greater than 6.9 mm on an anteroposterior open-mouth view of the upper cervical spine.65,66 Plain radiographs, however, are often inadequate to assess suspected transverse ligament injuries; and a combination of MRI, CT, and dynamic radiographs is often necessary to fully assess the type and extent of injury.66
Dickman and colleagues66 classified transverse ligament injuries into two types. Type I injuries encompass intrasubstance ruptures. Type Ia injuries occur in the midportion of the ligament. Type Ib injuries occur at the periosteal insertion of the ligament onto the atlas. Type II injuries occur when there is an avulsion of the tubercular insertion of the transverse ligament from the C1 lateral mass. Type IIa injuries occur if the lateral mass is comminuted, and type IIb injuries occur if the lateral mass is intact.
Type I injuries should be treated surgically with C1-C2 fusion. The most common way to achieve this is posteriorly. Wiring techniques such as Brooks and Gallie fusions are effective more than 90% of the time76 but are the least biomechanically stable,77 and a rigid cervical collar is usually used for 3 months postoperatively. Transarticular screw fixation (Fig. 76–16) provides sufficient additional stability to allow the immobilization time to be reduced to 6 weeks in a rigid collar or even to allow the patient to be immobilized in a soft cervical collar. There is, however, risk to the vertebral artery when instrumentation is done across the pars interarticularis of C2. C1 lateral mass screw and C2 pedicle screw constructs provide comparable biomechanical rigidity to transarticular screws with less risk to the vertebral artery but with more risk to the internal carotid artery. If necessary, sacrificing the C2 nerve root provides direct visualization of the C1 lateral mass and the C1-C2 joint. The structures can then be instrumented, reduced, and fused under direct visualization. The combination of C1 lateral mass screws connected to C2 translaminar screws is another alternative fixation option that provides the same degree of stability of the C1-C2 complex. C1-C2 fusion can also be achieved by an anterior approach with anteriorly placed C1-C2 screws.78 Proponents of this technique note that there is less soft tissue dissection involved as compared with posterior approaches.79 We, however, recommend the posterior approach in order to provide better supplemental bone grafting and to minimize postoperative swallowing and airway problems.
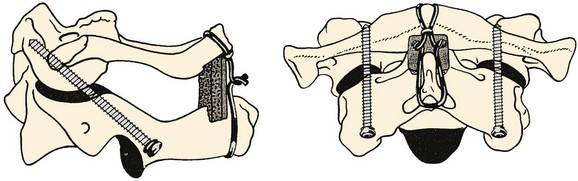
FIGURE 76–16 These lateral and anteroposterior views show the technique described by Grob and colleagues. They advise having the start point 3 to 4 mm cephalad to the C2-C3 facet joint and have it as medial as possible without penetrating the medial wall of the C2 pedicle. They emphasize seeing the medial and superior aspects of the C2 pedicle and also the C1-C2 joint. The anterior aspect of the screw should be either to the anterior cortex of C1 or just through it. As mentioned in the legend to Figure 76–11, the internal carotid artery lies just anterior to the usual exit point for the C1-C2 transarticular screw and, hence, it is extremely important that the screw tip either not protrude anterior at all or only a minimal distance. The illustration also demonstrates placement of an autogenous bone graft between the arches of C1 and C2 and adequately tightening the wires or cables, which, together with the C1-C2 transarticular screws, makes this an extremely strong construct. Packing bone graft within the C1-C2 joint may be done, but it adds considerably greater risk to the procedure and does not seem to be necessary under usual circumstances. It is necessary to perform fine-cut CT sagittal reconstruction views of C1 and C2 from side to side to be certain that there is adequate room for placement of a C1-C2 transarticular screw without compromising the vertebral artery. In approximately 15% of cases local anatomy of the vertebral artery precludes placement of two screws. Placement of one screw in combination with the posterior bone graft seems to be adequate.
(Reprinted with permission from Grob D, Jeanneret B, Aebi M, Markwalder T: Atlanto-axial fusion with transarticular screw fixation. J Bone Joint Surg Br 73:972-981, 1991.)
Type II fractures can be treated with external immobilization for 3 months. Up to 74% of these type II injuries will heal with nonoperative care.66
Fractures of the Odontoid
Fractures of the dens constitute 7% to 13% of all cervical spine injuries.80 They are frequently missed because of the paucity of clinical symptoms other than for neck pain. In addition, if the patient suffers from head trauma and is intoxicated or obtunded, the injury can be easily missed. Both flexion and extension mechanisms can cause fractures of the dens. Hyperflexion results in anterior displacement of the dens fracture, and hyperextension results in posterior displacement of the dens fracture.
The fracture can usually be seen on open-mouth and lateral radiographs of the cervical spine, although nondisplaced fractures can easily be missed. Classification of these fractures is based on their location in the odontoid. The most commonly used classification scheme was described by Anderson and D’Alonzo (Fig. 76–17).81 Type I fractures consist of avulsion injuries at the tip of the dens. Type II fractures occur through the base of the dens at the junction of the dens and the central body of the axis. Type III fractures extend into the body of the axis.
Type II odontoid fracture is the most problematic type of odontoid fracture. In a prospective study of 144 odontoid fractures reported by the Cervical Spine Research Society,82 there were 96 type II and 48 type III fractures. The incidence of spinal cord level neurologic deficits was 14 of 96 (14%) of type II fractures and 4 of 48 (8%) of type III fractures. Two of the 14 patients with type II fractures and cord deficits presented with upper extremity monoplegia, which probably represents the cruciate paralysis described earlier in this chapter.
The overall nonunion rate for type II odontoid fractures is reported to be about 32%. There is an increased nonunion rate associated with fractures with greater than 5 mm of displacement, angulation greater than 10 degrees, age older than 40 years, and posterior displacement.83 Hadley showed a 78% nonunion rate for type II fractures displaced more than 6 mm in comparison with a 10% nonunion rate when the displacement was less than 6 mm.84
As the risk factors for nonunion increase, surgical treatment with a C1-C2 fusion or anterior odontoid screw fixation becomes a better option. If primary C1-C2 fusion is selected, the patient should understand that he or she will lose 50% of neck rotation. Many options exist for fixation and fusion of C1-C2. C1-C2 transarticular screws (Fig. 76–18),85 C1 lateral mass/C2 pedicle screw constructs (Fig. 76–19), and C1 lateral mass/C2 translaminar screw constructs (Fig. 76–20) offer a biomechanical advantage over traditional wiring techniques and should minimize the chance of losing the fracture reduction and maximize the chance of having a solid fusion.86 A fusion rate and fracture union rate of 98% should be expected. If wiring techniques are used, a fusion rate of 94% to 96% can be expected, but there will be a higher chance of losing reduction. Gallie-type constructs are biomechanically advantageous for anteriorly displaced fractures, whereas Brooks constructs are advantageous for posteriorly displaced fractures.
Primary osteosynthesis of the dens (Fig. 76–21) has the theoretical advantage of preserving rotation at the atlantoaxial joint, but the amount of motion that is preserved over a C1-C2 fusion may not be significant.87 To achieve successful anterior fracture fixation, the fracture needs to be transverse, noncomminuted, and reducible. The patient’s chest anatomy and odontoid anatomy must also be amenable to this technique. In the elderly, the use of odontoid screws is associated with a high complication rate.88 Two large series of anterior odontoid screw fixation show failure rates of 13% to 17%.89,90
Biplanar fluoroscopy or intraoperative CT with image guidance is essential for this technique.
Traumatic Spondylolisthesis of the Axis
Traumatic spondylolisthesis of the axis, also known as a hangman’s fracture, can be caused by a variety of mechanisms including combinations of extension, flexion, distraction, and axial loading of the cervical spine. The fracture line passes through the neural arch of the axis. These fractures are best classified using the modification of the Effendi classification system (Fig. 76–22).91 Type I fractures occur through the pars interarticularis bilaterally with less than 3 mm translation and no angulation. This fracture usually results from hyperextension and axial load. The neural arch fractures, but the intervertebral disc and anterior longitudinal ligament are still intact (Fig. 76–23). This fracture is associated with other extension-type injuries including C1 posterior arch fractures and dens fractures.
Type II fractures are bipedicular fractures with greater than 3 mm of displacement and angulation of C2 on C3. This fracture results from an initial axial load with hyperextension of the neck causing a fracture of the neural arch, followed by a flexion moment that results in disruption of the C2-C3 intervertebral disc. This is often accompanied by a compression fracture of the anterosuperior corner of C3 or the posteroinferior body of C2.91 Two variants of type II fractures have been described. The type IIA fracture shows significant angulation but has minimal (rarely exceeding 2 to 3 mm) translation and includes significant disruption of the disc and posterior longitudinal ligament. Gross disc space distraction occurs after the application of minimal amounts of traction (Fig. 76–24). This injury usually results from a flexion-distraction injury. In a type II variant described by Starr and Eismont, the fracture line propagates through the posterior aspect of the vertebral body with unilateral or bilateral continuity of the posterior cortex and is associated with a 33% incidence of neurologic deficit (Fig. 76–25).92
Nonunions of type II or IIa injuries are uncommon,93 but if they occur and are symptomatic, they can be treated with either a posterior C1 to C3 fusion or an anterior C2-C3 fusion.
Summary
Pearls
Pitfalls
Key Points
1 Anderson LD, D’Alonzo RT. Fractures of the odontoid process of the axis. J Bone Joint Surg Am. 1974;56:16. 674
2 Anderson PA, Montesano PX. Morphology and treatment of occipital condyle fractures. Spine. 1988;13:7. 6
3 Clark CR, White AA. Fractures of the dens: A multicenter study. J Bone Joint Surg Am. 1985;67:13. 348
4 Dickman CA, Greene KA, Sonntag VK. Injuries involving the transverse atlantal ligament: Classification and treatment guidelines based upon experience with 39 injuries. Neurosurgery. 1996;38:44-50.
5 Harris JHJr, Carson GC, Waganer LK, Kerr N. Radiologic diagnosis of traumatic occipitovertebral dissociation: II. Comparison of three methods of detecting occipitovertebral relationships on lateral radiographs of supine subjects. AJR Am J Roentgenol. 1994;162:887-892.
6 Levine AM, Edwards CC. The management of traumatic spondylolisthesis of the axis. J Bone Joint Surg Am. 1985;67:217-226.
1 McGrory BJ, Klassen RA, Chao EY, et al. Acute fractures and dislocations of the cervical spine in children and adolescents. J Bone Joint Surg Am. 1993;75:988-995.
2 Sherk HH, Nicholson JT, Chung SM. Fractures of the odontoid process in young children. J Bone Joint Surg Am. 1978;60:921-924.
3 Alker GJJr, Oh YS, Leslie EV. High cervical spine and craniocervical junction injuries in fatal traffic accidents: A radiological study. Orthop Clin North Am. 1978;9:1003-1010.
4 Hachen HJ. Spinal cord injury in children and adolescents: Diagnostic pitfalls and therapeutic considerations in the acute stage [Proceedings]. Paraplegia. 1977;15:55-64.
5 Bohlman HH. Acute fractures and dislocations of the cervical spine: An analysis of three hundred hospitalized patients and review of the literature. J Bone Joint Surg Am. 1979;61:1119-1142.
6 Birney TJ, Hanley ENJr. Traumatic cervical spine injuries in childhood and adolescence. Spine. 1989;14:1277-1282.
7 Givens TG, Polley KA, Smith GF, Hardin WDJr. Pediatric cervical spine injury: A three-year experience. J Trauma. 1996;41:310-314.
8 Spivak JM, Weiss MA, Cotler JM, Call M. Cervical spine injuries in patients 65 and older. Spine. 1994;19:2302-2306.
9 Kiwerski JE. Injuries to the spinal cord in elderly patients. Injury. 1992;23:397-400.
10 Weingarden SI, Graham PM. Falls resulting in spinal cord injury: Patterns and outcomes in an older population. Paraplegia. 1989;27:423-427.
11 DeVivo MJ, Kartus PL, Rutt RD, et al. The influence of age at time of spinal cord injury on rehabilitation outcome. Arch Neurol. 1990;47:687-691.
12 Katzberg RW, Benedetti PF, Drake CM, et al. Acute cervical spine injuries: Prospective MR imaging assessment at a level 1 trauma center. Radiology. 1999;213:203-212.
13 Blackmore CC, Emerson EE, Mann FA, Koepsell TD. Cervical spine imaging in patients with trauma: Determination of fracture risk to optimize use. Radiology. 1999;211:759-765.
14 White AAIII, Panjabi MM. Clinical Biomechanics of the Spine. Philadelphia: JB Lippincott; 1978.
15 Werne S. Studies in spontaneous atlas dislocation. Acta Orthop Scand. 1957;Suppl 23:1-150.
16 Dvorak J, Schneider E, Saldinger P, Rahn B. Biomechanics of the craniocervical region: The alar and transverse ligaments. J Orthop Res. 1988;6:452-461.
17 Saldinger P, Dvorak J, Rahn BA, Perren SM. Histology of the alar and transverse ligaments. Spine. 1990;15:257-261.
18 Wang S, Wang C, Liu Y, et al. Anomalous vertebral artery in craniovertebral junction with occipitalization of the atlas. Spine. 2009;34:2838-2842.
19 Lyness SS, Simeone FA. Vascular complications of upper cervical spine injuries. Orthop Clin North Am. 1978;9:1029-1038.
20 Fitzgerald RD, Krafft P, Skrbensky G, et al. Excursions of the cervical spine during tracheal intubation: Blind oral intubation compared with direct laryngoscopy. Anaesthesia. 1994;49:111-115.
21 Donaldson WF3rd, Towers JD, Doctor A, et al. A methodology to evaluate motion of the unstable spine during intubation techniques. Spine. 1993;18:2020-2023.
22 Levine AM, Edwards CC. Fractures of the atlas. J Bone Joint Surg Am. 1991;73:680-691.
23 Lalani Z, Bonanthaya KM. Cervical spine injury in maxillofacial trauma. Br J Oral Maxillofac Surg. 1997;35:243-245.
24 Iida H, Tachibana S, Kitahara T, et al. Association of head trauma with cervical spine injury, spinal cord injury, or both. J Trauma. 1999;46:450-452.
25 Friedman DP, Tartaglino LM, Fisher AR, Flanders AE. MR imaging in the diagnosis of intramedullary spinal cord diseases that involve specific neural pathways or vascular territories. AJR Am J Roentgenol. 1995;165:515-523.
26 Vaccaro AR, Klein GR, Flanders AE, et al. Long-term evaluation of vertebral artery injuries following cervical spine trauma using magnetic resonance angiography. Spine. 1998;23:789-794. discussion 795
27 Cothren CC, Moore EE, Biffl WL, et al. Cervical spine fracture patterns predictive of blunt vertebral artery injury. J Trauma. 2003;55:811-813.
28 Weller SJ, Rossitch EJr, et al. Detection of vertebral artery injury after cervical spine trauma using magnetic resonance angiography. J Trauma. 1999;46:660-666.
29 Wirbel R, Pistorius G, Braun C, et al. Bilateral vertebral artery lesion after dislocating cervical spine trauma: A case report. Spine. 1996;21:1375-1379. discussion 1380
30 Vaccaro AR, Urban WC, Aiken RD. Delayed cortical blindness and recurrent quadriplegia after cervical trauma. J Spinal Disord. 1998;11:535-539.
31 Bell HS. Paralysis of both arms from injury of the upper portion of the pyramidal decussation: “cruciate paralysis.”. J Neurosurg. 1970;33:376-380.
32 Eismont FJ, Bohlman HH. Posterior atlanto-occipital dislocation with fractures of the atlas and odontoid process. J Bone Joint Surg Am. 1978;60:397-399.
33 Wang JC, Hatch JD, Sandhu HS, Delamarter RB. Cervical flexion and extension radiographs in acutely injured patients. Clin Orthop. 1999;365:111-116.
34 Blackmore CC, Ramsey SD, Mann FA, Deyo RA. Cervical spine screening with CT in trauma patients: A cost-effectiveness analysis. Radiology. 1999;212:117-125.
35 Katz MA, Beredjiklian PK, Vresilovic EJ, et al. Computed tomographic scanning of cervical spine fractures: Does it influence treatment? J Orthop Trauma. 1999;13:338-343.
36 Klein GR, Vaccaro AR, Albert TJ, et al. Efficacy of magnetic resonance imaging in the evaluation of posterior cervical spine fractures. Spine. 1999;24:771-774.
37 Vaccaro AR, Kreidl KO, Pan W, et al. Usefulness of MRI in isolated upper cervical spine fractures in adults. J Spinal Disord. 1998;11:289-293. discussion 294
38 Cotler HB, Kulkarni MV, Bondurant FJ. Magnetic resonance imaging of acute spinal cord trauma: Preliminary report. J Orthop Trauma. 1988;2:1-4.
39 Lee KH, Chung TS, Jeon TJ, et al. Application of spatial modulation of magnetization to cervical spinal stenosis for evaluation of the hydrodynamic changes occurring in cerebrospinal fluid. Korean J Radiol. Jan-Mar 2000;1:11-18.
40 Du R, Auguste KI, Chin CT, et al. Magnetic resonance neurography for the evaluation of peripheral nerve, brachial plexus, and nerve root disorders. J Neurosurg. 2010;112:362-371.
41 Sees DW, Rodriguez Cruz LR, Flaherty SF, Ciceri DP. The use of bedside fluoroscopy to evaluate the cervical spine in obtunded trauma patients. J Trauma. 1998;45:768-771.
42 Harris MB, Kronlage SC, Carboni PA, et al. Evaluation of the cervical spine in the polytrauma patient. Spine. 2000;25:2884-2891.
43 Blacksin MF, Lee HJ. Frequency and significance of fractures of the upper cervical spine detected by CT in patients with severe neck trauma. AJR Am J Roentgenol. 1995;165:1201-1204.
44 Bloom AI, Neeman Z, Slasky BS, et al. Fracture of the occipital condyles and associated craniocervical ligament injury: Incidence, CT imaging and implications. Clin Radiol. 1997;52:198-202.
45 Mariani PJ. Occipital condyle fracture presenting as retropharyngeal hematoma. Ann Emerg Med. 1990;19:1447-1449.
46 Anderson PA, Montesano PX. Morphology and treatment of occipital condyle fractures. Spine. 1998;13:731-736.
47 Maserati MB, Stephens B, Zohny Z, et al. Occipital condyle fractures: clinical decision rule and surgical management. J Neurosurg Spine. 2009;11:388-395.
48 Bucholz RW, Burkhead WZ, Graham W, Petty C. Occult cervical spine injuries in fatal traffic accidents. J Trauma. 1979;19:768-771.
49 Alker GJ, Oh YS, Leslie EV, et al. Postmortem radiology of head and neck injuries in fatal traffic accidents. Radiology. 1975;114:611-617.
50 Wholey MH, Bruwer AJ, Baker HLJr. The lateral roentgenogram of the neck; with comments on the atlanto-odontoid-basion relationship. Radiology. 1958;71:350-356.
51 Dublin AB, Marks WM, Weinstock D, Newton TH. Traumatic dislocation of the atlanto-occipital articulation (AOA) with short-term survival: With a radiographic method of measuring the AOA. J Neurosurg. 1980;52:541-546.
52 Lee C, Woodring JH, Goldstein SJ, et al. Evaluation of traumatic atlantooccipital dislocations. AJNR Am J Neuroradiol. 1987;8:19-26.
53 Powers B, Miller MD, Kramer RS, et al. Traumatic anterior atlanto-occipital dislocation. Neurosurgery. 1979;4:12-17.
54 Harris JH, Carson GC, Wagner LK. Radiologic diagnosis of traumatic occipitovertebral dissociation: I. Normal occipitovertebral relationships on lateral radiographs of supine subjects. AJR Am J Roentgenol. 1994;162:881-886.
55 Harris JHJr, Carson GC, Wagner LK, Kerr N. Radiologic diagnosis of traumatic occipitovertebral dissociation: II. Comparison of three methods of detecting occipitovertebral relationships on lateral radiographs of supine subjects. AJR Am J Roentgenol. 1994;162:887-892.
56 Traynelis VC, Marano GD, Dunker RP, Kaufman HH. Traumatic atlanto-occipital dislocation: Case report. J Neurosurg. 1986;65:863-870.
57 Hadley MN. Management of pediatric cervical spine and spinal cord injuries. Neurosurgery. 2002;50(3 Suppl):S85-S99.
58 Montane I, Eismont FJ, Green BA. Traumatic occipitoatlantal dislocation. Spine. 1991;16:112-116.
59 Finn MA, Bishop FS, Dailey AT. Surgical treatment of occipitocervical instability. Neurosurgery. 2008;63(5):961-968. discussion 968-969
60 Frush TJ, Fisher TJ, Ensminger SC, et al. Biomechanical evaluation of parasagittal occipital plating: screw load sharing analysis. Spine. Apr 20, 2009;34:877-884.
61 Garrido BJ, Puschak TJ, Anderson PA, et al. Occipitocervical fusion using contoured rods and medial offset connectors: description of a new technique. Orthopedics. 32(10), 2009.
62 Uribe JS, Ramos E, Vale F. Feasibility of occipital condyle screw placement for occipitocervical fixation: a cadaveric study and description of a novel technique. J Spinal Disord Tech. 2008;21:540-546.
63 Uribe JS, Ramos E, Baaj A, et al. Occipital cervical stabilization using occipital condyles for cranial fixation: technical case report. Neurosurgery. 2009;65:E1216-E1217. discussion E1217
64 Connolly B, Turner C, DeVine J, Gerlinger T. Jefferson fracture resulting in Collet-Sicard syndrome. Spine. 2000;25:395-398.
65 Spence KFJr, Decker S, Sell KW. Bursting atlantal fracture associated with rupture of the transverse ligament. J Bone Joint Surg Am. 1970;52:543-549.
66 Dickman CA, Greene KA, Sonntag VK. Injuries involving the transverse atlantal ligament: Classification and treatment guidelines based upon experience with 39 injuries. Neurosurgery. 1996;38:44-50.
67 Lee TT, Green BA, Petrin DR. Treatment of stable burst fractures of the atlas (Jefferson fracture) with rigid cervical collar. Spine. 1998;23:1963-1967.
68 Levine AM, Edwards CC. Treatment of injuries in the C1-C2 complex. Orthop Clin North Am. 1986;17:31-44.
69 Fowler JL, Sandhu A, Fraser RD. A review of fractures of the atlas vertebra. J Spinal Disord. 1990;3:19-24.
70 Hadley MN, Dickman CA, Browner CM, Sonntag VK. Acute traumatic atlas fractures: Management and long term outcome. Neurosurgery. 1988;23:31-35.
71 McGuire RAJr, Harkey HL. Primary treatment of unstable Jefferson’s fractures. J Spinal Disord. 1995;8:233-236.
72 Segal LS, Grimm JO, Stauffer ES. Bursting atlantal fracture associated with rupture of the transverse ligament. J Bone Joint Surg Am. 1987;69:1423-1434.
73 Phillips WA, Hensinger RN. The management of rotatory atlanto-axial subluxations in children. J Bone Joint Surg Am. 1989;71:664-668.
74 Fielding JW, Hawkins RJ. Atlanto-axial rotatory fixation. (Fixed rotatory subluxation of the atlanto-axial joint). J Bone Joint Surg Am. 1977;59:37-44.
75 Fielding JW, Cochran GB, Lawsing JF3rd, Hohl M. Tears of the transverse ligament of the atlas: A clinical and biomechanical study. J Bone Joint Surg Am. 1974;56:1683-1691.
76 Brooks AL, Jenkins EB. Atlanto-axial arthrodesis by the wedge compression method. J Bone Joint Surg Am. 1978;60:279-284.
77 Crawford NR, Hurlbert RJ, Choi WG, Dickman CH. Differential biomechanical effects of injury and wiring at C1-C2. Spine. 1999;24:1894-1902.
78 Lesoin F, Autricque A, Franz K, et al. Transcervical approach and screw fixation for upper cervical spine pathology. Surg Neurol. 1987;27:459-465.
79 Reindl R, Sen M, Aebi M. Anterior instrumentation for traumatic C1-C2 instability. Spine. 1993;28:E329-E333.
80 Amyes EW, Anderson FM. Fracture of the odontoid process; report of sixty-three cases. AMA Arch Surg. 1956;72:377-393.
81 Anderson LD, D’Alonzo RT. Fractures of the odontoid process of the axis. J Bone Joint Surg Am. 1974;56:1663-1674.
82 Clark CR, White AA. Fractures of the dens: a multicenter study. J Bone Joint Surg Am. 1985;67:1340-1348.
83 Roberts A, Wickstrom J. Prognosis of odontoid fractures. Acta Orthop Scand. 1973;44:21-30.
84 Hadley MN, Dickman CA, Browner CM, Sonntag VK. Acute axis fractures: A review of 229 cases. J Neurosurg. 1989;71(5 Pt 1):642-647.
85 Grob D, Jeanneret B, Aebi M, Markwalder TM. Atlanto-axial fusion with transarticular screw fixation. J Bone Joint Surg Br. 1991;73:972-976.
86 Parker SL, McGirt MJ, Garces-Embrossi GL, et al. Translaminar versus pedicle screw fixation of C2: comparison of surgical morbidity and accuracy of 313 consecutive screws. Neurosurgery. 2009;64(5 Suppl 2):343-348. discussion 348-349
87 Jeanneret B, Vernet O, Frei S, Magerl F. Atlantoaxial mobility after screw fixation of the odontoid: A computed tomographic study. J Spinal Disord. 1991;4:203-211.
88 Andersson S, Rodrigues M, Olerud C. Odontoid fractures: High complication rate associated with anterior screw fixation in the elderly. Eur Spine J. 2000;9:56-59.
89 Chiba K, Fujimura Y, Toyama Y, et al. Treatment protocol for fractures of the odontoid process. J Spinal Disord. 1996;9:267-276.
90 Vieweg U, Meyer B, Schramm J. Differential treatment in acute upper cervical spine injuries: A critical review of a single-institution series. Surg Neurol. 2000;54:203-210.
91 Levine AM, Edwards CC. The management of traumatic spondylolisthesis of the axis. J Bone Joint Surg Am. 1985;67:217-226.
92 Starr JK, Eismont FJ. Atypical hangman’s fractures. Spine. 1993;18:1954-1957.
93 Francis WR, Fielding JW, Hawkins RJ, et al. Traumatic spondylolisthesis of the axis. J Bone Joint Surg Br. 1981;63:313-318.