20 Inhalation anesthesia
Adjustable Positive-Pressure Relief (APR) Valve: Used for release of excessive gas on the circle system of an anesthesia machine.
Amnesia: A component of anesthesia in which the patient is unable to recall the events that occurred during the administration of the inhalational anesthetic.
Analgesia: A component of anesthesia in which the patient is unable to experience pain.
CO2 Absorption Canisters: Located in a circle system on an anesthesia machine that clears rebreathed gas that contains carbon dioxide by passing through a canister containing a chemical carbon dioxide absorbent.
Delirium: A portion of a stage of anesthesia in which the patient has a transient disturbance during a loss of consciousness accompanied by a change in cognition that has a fluctuating course.
Diffusion Hypoxia: Sometimes referred to as the Fink phenomenon; refers to the rapid exit of nitrous oxide and thus partial reduction of the percentage of oxygen that can be inhaled during the immediate (first 4 to 5 minutes) emergence phase of recovery from anesthesia. Supplemental oxygen and the use of the modified stir-up regime negate this effect of nitrous oxide on emergence.
Effective Dose (ED): The dose of a drug necessary to produce a certain effect in a certain percentage of patients. For example, the ED50 is the term for when a drug produces a particular effect in 50% of patients.
Hypnosis: A component of anesthesia in which the patient becomes unconscious.
Inhalation Anesthesia: Anesthetic substances, in either volatile or gaseous form, that are inhaled via an anesthesia machine.
Lacrimation: Tears from the lacrimal glands on the medial side of the tissue surrounding the eyes.
Minimal Alveolar Concentration (MAC): A measure of potency of inhalation anesthetic agents; occurs when the equilibrium end-tidal anesthetic concentrations, expressed as a fraction of 1 atm, prevent movement in response to surgical skin incision in 50% of human subjects.
Muscle Relaxation: A component of anesthesia in which the patient has reduced tension of the skeletal muscle.
Nociception: A component of anesthesia in which the patient is unable to sense pain.
Scavenger System: Used to reduce exposure to escaping gases from the anesthesia machine; a waste gas suction tube (scavenger) is connected to the adjustable positive-pressure relief valve and anesthesia ventilator relief valve, and the gases are then vented to the outside atmosphere via an operating room suction system.
Solubility Coefficient: The ratio of the concentration of an anesthetic in blood or other tissue to that in a gas phase when the two are in equilibrium.
Sympatholysis: A component of anesthesia in which the patient is blocked from having an autonomic response to nociceptive (painful) stimuli.
Vaporizer: A device on the anesthesia machine that converts liquid anesthetics into metered amounts of vapor that are added to the fresh gas mixture to produce a known concentration of the vaporized form of the inhalational anesthetic agent.
The inhalation anesthetic agents in use today have survived the many examinations of research, and clinically they have been shown to add significant safety factors and improved outcomes for perianesthesia patients. Research continues in this area; for example, xenon is under evaluation as an inhalation anesthetic agent.1 Other volatile agents are undergoing evaluation, all in an effort to find the inhalational agent that best represents all the facets of anesthesia, such as muscle relaxation, sedation, analgesia, amnesia, with minimal effects on the major organ systems of the body.
Basic concepts
Evolution of the signs and stages of anesthesia
The five components of anesthesia are hypnosis, analgesia, muscle relaxation, sympatholysis, and amnesia. In the past, when diethyl ether was the primary general anesthetic, assessment of anesthetic depth with the signs and stages of anesthesia was simple; the patient could be monitored by assessing the pupils, respiratory activity, muscle tone, and various reflexes. The ether signs and stages were devised to provide a means of assessing the depth of anesthesia. The first three stages were described by Plomley in 1847; 1 year later, John Snow added a fourth stage: overdose.2 During World War I, Guedel more accurately defined and described the signs and stages of anesthesia. A graphic representation of these signs and stages is provided in Fig. 20-1.
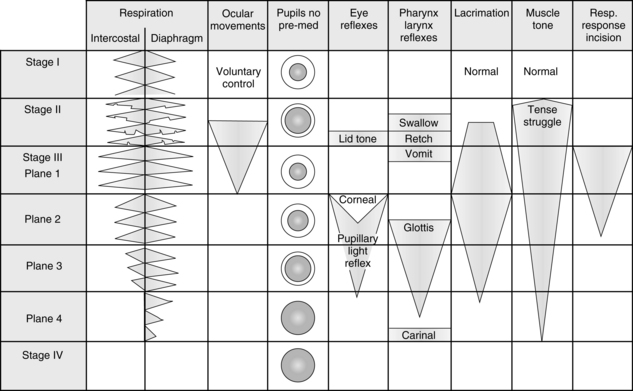
FIG. 20-1 Signs and reflex reactions of stages of anesthesia.
(Adapted from Gillespie NA: Signs of anesthesia, Anesth Analg 22:275, 1943.)
Stage I begins with the initiation of anesthesia and ends with the loss of consciousness. It is commonly called the stage of analgesia. This stage has been described as the lightest level of anesthesia and represents sensory and mental depression. Stage I is the level of anesthesia used with nitrous oxide. Patients are able to open their eyes on command, breathe normally, maintain protective reflexes, and tolerate mild painful stimuli.
Stage III is the stage of surgical anesthesia. With ether anesthesia, this stage is defined as lasting from the onset of a regular pattern of breathing to the cessation of respiration. At this stage of anesthesia, response to surgical incision is absent. The modern concept of minimal alveolar concentration (MAC) is predicated in part with the signs and stages of surgical anesthesia. MAC is exceeded by a factor of 1.3 in stage III because most patients do not respond to surgical incision at this level of anesthesia. Patients who receive 1.3 MAC anesthesia3 have a depression in all elements of nervous system function—that is, sensory depression, loss of recall, reflex depression, and some skeletal muscle relaxation. From this point, with the modern anesthetics, increased MAC results in further respiratory, cardiovascular, and central nervous system (CNS) depression. The difficulty is that each of the newer agents affects the clinical signs, such as blood pressure, differently. Consequently, monitoring of the level of anesthesia depends on the particular properties of each agent.
Most surgical procedures in which ether anesthesia was used were performed at this stage of anesthesia, which is divided into four planes.4 Plane 1 is entered when the lid reflex is abolished and respiration becomes regular. During this plane, the vomiting reflex is gradually abolished. The nurse working in the PACU must know that swallowing, retching, and vomiting reflexes tend to disappear in that order during induction and reappear in the same order during emergence from anesthesia.
Some of the more reliable indicators of depth of anesthesia for the more modern inhalation anesthetics include changes in breathing pattern, eye movement, lacrimation, and muscle tone. Because the ventilation is under autonomic control, it is the most sensitive indicator of depth of anesthesia. In the PACU, a patient who uses diaphragmatic ventilation without the intercostal muscles should be considered to be in surgical anesthesia. As the ventilatory pattern returns to a more normal rate, rhythm, and pattern, the patient can be considered to be in light anesthesia and about to have total emergence. Eye movement as opposed to pupillary size is a good indicator of anesthetic depth. Light anesthesia is present with eye movement. Deeper anesthesia is present when the eyes are close together in a cross-eyed position. Lacrimation does not occur during surgical anesthesia when a patient receives desflurane (Suprane), isoflurane (Forane), or sevoflurane (Ultane). Conversely, if a patient received one of those drugs and has tearing, light anesthesia can be considered to be present. As the depth of anesthesia is increased, the amount of muscle tone decreases. Therefore, if a patient in the PACU lacks muscle tone, especially in the jaw and abdomen, the patient should be considered to be in a surgical depth of anesthesia. With the assessment of the degree of muscle tone, the perianesthesia nurse must critically assess the degree of reversal of skeletal muscle relaxants (see Chapter 23) before determining the depth of anesthesia with the criterion of muscle tone. Finally, because the determinants of anesthesia depth have such a high degree of variability, all possible assessment tools should be incorporated into the care of the patient in the PACU. The bottom line is constant vigilance of the patient’s physiologic parameters during emergence from anesthesia and the institution of appropriate nursing interventions based on an ongoing assessment.
Pharmacokinetics of inhalation anesthetics
The pharmacokinetics of inhalation anesthetics involve uptake, distribution, metabolism, and elimination. Basically, this involves a series of partial pressure gradients starting in the anesthesia machine to the patient’s brain for induction, and vice versa for emergence. The object of anesthesia is a constant and optimal partial pressure in the brain. The key to attainment of anesthesia is the alveolar partial pressure (PA) in equilibrium with the arterial partial pressure (Pa) and brain partial pressure (Pbr) of the inhaled anesthetic.4 The partial pressure of an inhalation anesthetic in the brain is used to determine the depth of anesthesia. The more potent the anesthetic, the lower the partial pressure of the agent needed to produce a certain depth of anesthesia.
Movement of inhalation anesthetic from alveoli to arterial blood
The movement of the inhalation anesthetic agent from the alveoli to the arterial blood depends on the blood-gas partition coefficient and the cardiac output. The rate at which the anesthetic is taken up by the blood and tissues is governed in part by the solubility of the agent in blood. This is expressed as the blood-gas partition coefficient, or the Oswald solubility coefficient, and is defined as the ratio of the concentration of an anesthetic in blood to that in a gas phase when the two are in equilibrium (Table 20-1). This concept is difficult to understand because the more soluble the anesthetic agent is, the slower the agent is in producing anesthesia. This effect is because the blood serves as a reservoir and a large volume of the agent must be introduced to attain an equilibrium between the blood partial pressure and the partial pressure in the lungs.
The blood conveys the anesthetic agent to the tissues. Consequently, a normal cardiac output is needed for facilitation of the movement of the inhalation anesthetic through the tissues to the brain. The partial pressure increases most rapidly in the tissues with the highest rates of blood flow. Of interest is the great variation in blood perfusion of certain tissues in the body. The body tissue compartments can be divided into the following major groups:
• The vessel-rich group, which consists of the heart, brain, kidneys, hepatoportal system, and endocrine glands
• The intermediate group of perfused tissues, which consists of muscle and skin
• The fat group, which includes marrow and adipose tissue
• The vessel-poor group, which has the poorest circulation per unit volume and comprises tendons, ligaments, connective tissue, teeth, bone, and other avascular tissue