Chapter 104 Inflammation and Immunity
Systemic Inflammatory Response Syndrome, Sepsis, Acute Lung Injury, and Multiple Organ Failure
The Inflammation/Coagulation/Immune Dysfunction/Dysregulated Metabolism Hypothesis
Roger Bone popularized the notion that systemic inflammation led to the development of sepsis syndrome and MOF in the 1990s. According to this theory, an initial proinflammatory cytokine response to foreign or self-antigen caused SIRS, a systemic inflammatory response characterized by two of four criteria: tachycardia, tachypnea, fever, and leukocytosis. This syndrome was thought to be mediated by increased interleukin (IL)-1 and tumor necrosis factor (TNF)-α levels (Figure 104-1). It could be blunted by nonsteroidal antiinflammatory drugs and hence was prostaglandin mediated. A systemic inflammatory response was called sepsis if related to infection and SIRS if related to surgery, pancreatitis, or other noninfectious processes. This syndrome was self-limited when antibiotics were used to kill the infection or time allowed recovery from surgery, pancreatitis, and other noninfectious causes. This proinflammatory response was turned off by an antiinflammatory response led by the antiinflammatory cytokine IL-10. When IL-10 resulted in reduction of IL-1 and TNF-α to normal control values, sepsis or SIRS stopped and organ failure did not occur (Figure 104-2). In patients who developed severe sepsis and MOF, the compensatory anti-inflammatory response was unsuccessful in turning off inflammation. Instead, these patients had persistent elevation of the cytokine IL-6 along with IL-10. Bone coined the term immunologic dissonance to describe this process. He viewed MOF as an inflammatory condition in which the normal antiinflammatory response was unable to turn off the proinflammatory process (Figure 104-3).
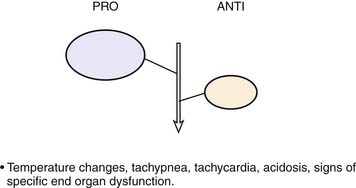
Figure 104–1 SIRS shows proinflammation self-limited by antiinflammation and eradication of the source of inflammation.
Proulx and colleagues1 defined pediatric MOF syndromes as primary and secondary. Primary MOF occurred in 90% of children and was present at the time of admission, and secondary MOF occurred in 10% of children and was not present until 7 days after admission.1 To evaluate the role of inflammation in pediatric sepsis-induced MOF, this group defined children as having no MOF (NMOF) if they had two-organ failure at presentation but never reached three-organ failure, resolved MOF (RMOF) if they had three-organ failure at admission that resolved to two-organ or less at 48 hours, persistent MOF (PMOF) if they had three or more organ failures at presentation that did not resolve by 48 hours, and sequential MOF (SMOF) if they had acute lung injury/adult respiratory distress syndrome at admission and then progressed to hepatorenal failure/dysfunction.2 Similar to Proulx’s classification, children in the latter investigation with SMOF represented 10% of the population. The mortality rates with NMOF, RMOF, PMOF, and SMOF were 2%, 8%, 35%, and 50%, respectively. The authors then investigated Bone’s hypothesis in this categories and reported that patients with NMOF had an increase in IL-10 with subsequent turning off of inflammation; however, those with PMOF or SMOF had persistently high IL-6 and IL-10 levels—the hallmark of Bone’s “immunologic dissonance.”2
Bone’s untimely death prevented him from further pursuing the mechanisms by which immunologic dissonance could mediate organ injury. However, Proulx et al. first reviewed the autopsy bank at their hospital and noted that children who died from sepsis-induced MOF exhibited two unsuspected findings compared with children who died of other causes: persistent or unrecognized infection and thrombotic and bleeding complications with organ infarctions. Accordingly, they pursued the notion that immunologic dissonance might lead to an inability to mediate coagulation homeostasis as well as an inability to clear infection. Proinflammatory cytokines including TNF-α and IL-1 induce endothelial activation in vitro with an increased prothrombotic and antifibrinolytic state and expression of adhesion molecules (Figures 104-4 to 104-6). Patients with PMOF had increased tissue factor and plasminogen activator-1 activity as well as circulating adhesion molecules, intercellular adhesion molecule, and vascular adhesion molecule consistent with the hypothesis that immunologic dissonance was associated with an activated prothrombotic/antifibrinolytic endothelium.3,4 IL-6 also inhibits ADAMTS-13, the von Willebrand factor (vWF) multimer-cleaving protease, in vitro. PMOF patients also had deficient ADAMTS-13 activity compared with NMOF patients, consistent with increased risk of vWF multimer–mediated thrombosis.5 Increased IL-10 is the hallmark of the TH2 antiinflammatory cytokine response. When the TH2 response is dominant, the TH1 response can be turned off, a state known as immune paralysis (Figure 104-7). These patients have a diminished ex vivo ability to secrete TNF-α from endotoxin-stimulated monocytes or macrophages. Without this ability, infections cannot be terminated. PMOF patients exhibited immune paralysis more frequently than did NMOF patients.6
Cytokines and nitric oxide also inhibit cytochrome P450 activity and mitochondrial respiration in vitro. One study group found that patients with PMOF had reduced cytochrome P450 (10-fold) activity compared with patients with NMOF (twofold).7 Immunologic dissonance in PMOF was associated with endotheliopathy, immune paralysis, and poor metabolism, but how was PMOF different from SMOF? The authors determined that patients with SMOF were more likely to have viral or lymphoproliferative disease with very high soluble Fas ligand (sFasL) levels. In vitro, sFasL levels greater than 500 pg/mL cause hepatocyte necrosis. Children with MOF with sFasL levels greater than 500 pg/mL demonstrated liver injury at autopsy.8 The mechanism of injury in SMOF was different than in patients who presented with MOF (Figure 104-8).
In this decade, investigators from the United Kingdom have investigated the inflammation/immune dysregulation hypothesis in SIRS/sepsis, hypothesizing that reduced complement activity increases the risk of SIRS/sepsis.9 Mannose-binding lectin (MBL) is the first complement component released and is required for the first 12 hours of complement activity. Approximately 30% of children are MBL deficient. Complement factor H inhibits complement activity. The complement H factor H Y402H polymorphism reduces this complement inhibition activity. Using genotype analyses, these investigators have demonstrated that children at risk for SIRS/sepsis more commonly have deficiencies in MBL production and a reduction in this H factor polymorphism. These two polymorphisms together lead to an even greater risk of SIRS/sepsis than either alone. The interpretation is that diminished complement function prevents rapid clearance of antigen, leading to the SIRS/sepsis syndrome. The inflammation hypothesis is alive and well in SIRS, sepsis, severe sepsis, and MOF.
Definitions and Scoring
As previously noted, SIRS is defined by two of the following four criteria: tachypnea, tachycardia, fever, and leukocytosis. Sepsis is defined as SIRS plus suspected or documented infection. Severe sepsis is defined as sepsis plus organ failure. Acute lung injury is defined by bilateral pulmonary infiltrates and a PaO2/FIO2 ratio less than 300. MOF or multiple organ dysfunction syndrome is defined by failure of more than one organ. Many definitions exist for defining organ failure. Wilkinson and Pollack10