Chapter 1
Indications for Pulmonary Function Testing
1. Categorize pulmonary function tests according to specific purposes.
2. List indications for spirometry, lung volumes, and diffusing capacity.
3. Identify at least one obstructive and one restrictive pulmonary disorder.
4. Relate pulmonary history to indications for performing pulmonary function tests.
1. Identify three indications for exercise testing.
2. Name at least two diseases in which air trapping may occur.
3. Describe the use of a technologist-adapted protocol for pulmonary function studies.
Pulmonary function tests
Many different tests are used to evaluate lung function. These tests can be divided into categories based on the aspect of lung function they measure (Box 1-1). Although the tests can be performed individually, they are often performed in combination. Figure 1-1 shows a sample pulmonary function test report that includes spirometry, lung volumes, diffusing capacity, and airway resistance measurements in a format that is commonly used. Determining which tests to do depends on the clinical question to be answered. This question may be explicit, such as, “Does the patient have asthma?” or less obvious, such as, “Does this patient, who needs thoracic surgery, have any pulmonary disease that might complicate the procedure?” In either case, indications for specific tests are useful (see Boxes 1-2 through 1-6).
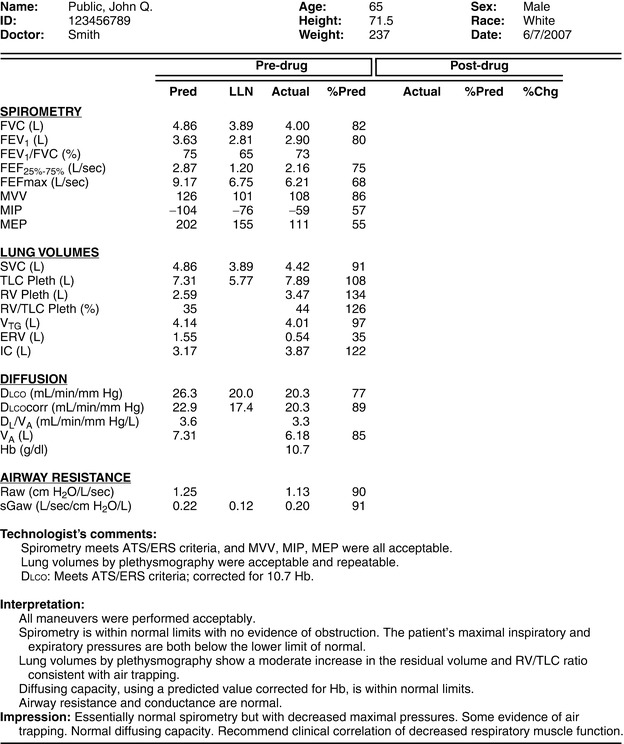
Lung function tests are grouped by category in the left column. The post-drug columns are blank because the patient was not retested after the bronchodilator.
LLN, Lower limit of normal.
Airway Function Tests
The most basic test of pulmonary function is the measurement of vital capacity (VC). This test simply measures the largest volume of air that can be moved into or out of the lungs. In the mid-1800s, a surgeon named Hutchinson developed a simple water-sealed spirometer that allowed measurement of what he named “vital capacity or vital breath” as he noted its relationship to survival. Hutchinson popularized the concept of using VC to assess lung function and named several other lung compartments that are still used today. He observed that VC was related to the standing height of the patient. He also developed tables to estimate the expected VC for a healthy patient. The VC was usually graphed on chart paper, which allowed subdivisions of the VC to be identified (see Chapter 2).
In addition to displaying FVC as a volume-time spirogram, it can also be represented by plotting airflow against volume. In the late 1950s, Hyatt and others began using the flow-volume display to assess airway function. The tracing was termed the maximal expiratory flow volume (MEFV) curve. By combining the forced expiration with an inspiratory maneuver, a closed loop can be displayed. This figure is called the flow-volume loop (see Chapter 2).
The FVC, FEV1, and other flows, along with flow-volume loops, are all used to measure response to bronchodilator medications (see Chapter 2). Tests are performed before and after inhalation of a bronchodilator, and the percentage of change is calculated. The same tests may be used to assess airway response after a challenge to the airways. These tests are referred to as bronchial challenge or bronchial provocation tests. The challenge may be in the form of a nonspecific inhaled agent (e.g., methacholine) or a physical agent (e.g., exercise). In either case, airflow is assessed before and after the challenge. The percent change (normally a decrease) after the challenge is calculated (see Chapter 9).
Airway resistance (Raw) measurements date back to the development of the body plethysmograph in the early 1950s. Comroe, DuBois, and others perfected a technique that provided estimates of alveolar pressure. The patient sits in an airtight box called a plethysmograph (see Chapter 11). The plethysmographic method allows the calculation of the pressure drop across the airways related to flow at the mouth (see Chapter 4). This technique originally required complicated monitoring and recording devices. Microprocessors have simplified the measurement of the required signals so that plethysmography is now widely used. The same equipment can also be used to rapidly and accurately measure thoracic gas volume (VTG).
Lung compliance is measured by passing a small balloon into the esophagus to measure pleural pressure. Intrapleural pressure can then be related to volume changes to estimate the distensibility of the lung (see Chapter 4). Other less invasive techniques are available but not widely used.
Lung Volume and Ventilation Tests
Closed-circuit (He dilution) and open-circuit (N2 washout) techniques are both widely used to measure FRC. Besides determining lung volumes, each technique provides some limited information about the distribution of ventilation within the lungs. The pattern of N2 washout can be displayed graphically. The time required for He to equilibrate during rebreathing provides a similar index of the evenness of ventilation. In the early 1950s and 1960s, Fowler developed a single-breath N2-washout technique. This method plotted N2 concentration in expired gas after a single breath of 100% oxygen. The single-breath N2 washout also provides limited information about gas distribution in the lungs. It also allows estimates of the lung volume at which airway closure occurs when the patient exhales completely (see Chapter 4).
Diffusing Capacity Tests
The basis for the modern single-breath diffusing capacity (Dlco) test was described by August and Marie Krogh in 1911. They showed that small but measurable differences existed between inspired and expired gas containing carbon monoxide (CO). This change could be related to the uptake of gas across the lung. Although they used the method to test a series of patients, they did not use the single-breath technique for clinical purposes. Around 1950, Forrester and others revisited the method. They developed it as a tool to measure the gas exchange capacity of the lung. About the same time, Filley and others were promoting other techniques, using CO to measure Dlco. Most of these techniques allowed patients to breathe normally, rather than hold their breath. These methods are called steady-state techniques. Each method has certain limitations. However, the single-breath technique is the most widely used and standardized in the United States and Europe (see Chapter 5).
Blood Gases and Gas Exchange Tests
Measurement of gases (O2 and CO2) in the blood began with volumetric methods used since the early 1900s. In 1957, Sanz introduced the glass electrode to measure pH of fluids potentiometrically. In 1958, Severinghaus added an outer jacket containing a bicarbonate buffer to the glass electrode. The electrode-buffer was separated from the blood being analyzed by a membrane that was permeable to CO2. This allowed the pressure of CO2 in the blood to be measured as a pH change in the electrode. In 1956, Leland Clark covered a platinum electrode with a polypropylene membrane. When a voltage was applied to the electrode, O2 was reduced at the platinum cathode in proportion to its partial pressure. These three electrodes (pH, Pco2, and partial pressure of oxygen [Po2]) were the basic measurement device in blood gas analyzers for many years. Miniature electrodes gradually replaced the traditional electrodes. Today, blood gas analyzers use a variety of electrochemical techniques (see Blood Gas Analyzers, Chapter 11) to measure not only pH, Pco2, and Po2, but also the various fractions of Hb, such as O2Hb and COHb. Similar methods to measure electrolytes (K++, Na++, Cl–) are also included in many blood gas analyzer systems. Transcutaneous electrodes, using techniques similar to the classical blood gas electrodes, are available for the measurement of O2 and CO2 tensions (tcpO2 and tcpCO2).
Capnography, or monitoring of exhaled carbon dioxide, was developed in conjunction with the infrared gas analyzer (see Chapter 11). This sensitive and rapidly responding analyzer allows exhaled CO2 to be monitored continuously. Most critical care units, operating rooms, and emergency departments use some combination of blood gas analysis, pulse oximetry, and capnography for patient monitoring. Blood gas analysis is an integral part of routine pulmonary function testing because it is the definitive test of the basic functions of the lung.
Indications for pulmonary function testing
Spirometry
Spirometry is the pulmonary function test performed most often because it is indicated in many situations (see Box 1-2). Spirometry is often performed as a screening procedure. It may be the first test to indicate the presence of pulmonary disease. Spirometry is recommended as the “gold standard” for diagnosis of obstructive lung disease by the National Lung Health Education Program (NLHEP), the National Heart Lung and Blood Institute (NHLBI), the World Health Organization (WHO), and numerous other organizations concerned with the diagnosis of lung diseases. However, spirometry alone may not be sufficient to completely define the extent of disease, response to therapy, preoperative risk, or level of impairment. Spirometry must be performed correctly because of the serious impact its results can have on the patient’s life. It is one of the few tests that yields a false-positive response if performed poorly because low values are the result. These low results may lead to further testing, inappropriate diagnosis, treatment, and increased costs for the patient and the health care delivery system.
Lung Volumes
Lung volume determination usually includes the VC and its subdivisions, along with the FRC. From these two basic measurements, TLC and other lung volumes can be calculated (see Chapter 4). Lung volumes are almost always measured in conjunction with spirometry, although the indications for them are distinct (see Box 1-3). The most common reason for measuring lung volumes is to identify restrictive lung disease. A reduced VC (or FVC) measured with spirometry may suggest restriction, particularly if airflow is normal. Measurement of FRC and determination of TLC are necessary to confirm restriction because a low FVC can be caused by either restriction or obstruction. If TLC is reduced below the 5th percentile of the predicted value, restriction is present. The severity of the restrictive process is determined by the extent of reduction of the TLC. TLC and its components can be determined by several methods. For patients with obstructive lung diseases (COPD, asthma), lung volumes measured by body plethysmography may be indicated (see Chapter 4) because multiple-breath or single-breath dilution techniques may underestimate TLC. In obstructive lung disease, lung volumes are necessary to determine whether air trapping or hyperinflation is present. The degree of hyperinflation, measured by indices such as the IC/TLC ratio, correlates with increased mortality in patients who have COPD.
Diffusing Capacity
Diffusing capacity is measured by having the patient inhale a low concentration of CO and a tracer gas to determine gas exchange within the lungs (Dlco). Several methods of evaluating the uptake of CO from the lungs are available, but the single-breath technique (Dlcosb) is most commonly used. This method is also called the breath-hold technique because CO transfer is measured during 10 seconds of breath holding. Dlco is usually measured in conjunction with spirometry and lung volumes. Although many pulmonary and cardiovascular diseases reduce Dlco (see Box 1-4), it may be abnormally increased in some cases (see Chapter 5). Dlco testing is commonly used to monitor diseases caused by dust (pneumoconioses). These are conditions in which lung tissue is infiltrated by substances such as silica or asbestos that disrupt the normal structure of the gas exchange units. Dlco testing is also used to evaluate pulmonary involvement in systemic diseases such as rheumatoid arthritis. Dlco measurements are often included in the evaluation of patients with obstructive lung disease, particularly in emphysema. Dlco tests may be indicated to monitor changes in lung function (i.e., gas exchange) induced by drugs used to treat cardiac arrhythmias, as well as changes caused by chemotherapy and radiation therapy for lung cancer.
Blood Gases
Blood gas analysis is the ideal measure of pulmonary function because it assesses the two primary functions of the lung (oxygenation and CO2 removal). Evaluation of many pulmonary disorders may include blood gas analysis. Specific indications for blood gas analysis are listed in Box 1-5. Blood gas analysis is most commonly used to determine the need for supplemental oxygen and to manage patients who require ventilatory support. Some pulmonary function measurements require blood gas analysis as an integral part of the test (i.e., shunt or dead space studies). Blood gas analysis is invasive; noninvasive measurements of oxygenation or gas exchange are often preferred since they are safer or less costly. Many noninvasive techniques (e.g., pulse oximetry, transcutaneous monitoring, and capnography) rely on a blood gas analysis to verify their validity (see Chapter 6).
Exercise Tests
Physical exercise stresses the heart, lungs, and the pulmonary and peripheral circulatory systems. Exercise testing allows simultaneous evaluation of the cellular, cardiovascular, and ventilatory systems. Cardiopulmonary exercise tests can be used to determine the level of fitness or extent of dysfunction. Appropriately designed tests can determine the role of cardiac or pulmonary involvement. COPD, interstitial lung disease, pulmonary vascular disease, and exercise-induced bronchospasm are respiratory disorders that often require exercise evaluation. Understanding the physiologic basis for the patient’s inability to exercise is an important aspect in prescribing effective therapy (i.e., cardiac or pulmonary rehabilitation). Exercise testing may also be required for the determination of disability. Box 1-6 lists specific indications for exercise tests.
Equipment used to measure oxygen consumption and CO2 production during exercise can also measure resting metabolic rates. This allows estimates of caloric needs in patients who are critically ill. Indications for performing studies of REE are detailed in Chapter 9.
Patterns of impaired pulmonary function
Obstructive Airway Diseases
Chronic Obstructive Pulmonary Disease
The term COPD is often used to describe long-standing airway obstruction caused by emphysema, chronic bronchitis, or asthma. These three conditions may be present alone or in combination (Figure 1-2). Bronchiectasis is sometimes considered a component of COPD. COPD is characterized by dyspnea at rest or with exertion, often accompanied by a productive cough. Delineation of the type of obstruction depends on the history, physical examination, and pulmonary function studies. Unfortunately, the term COPD is used to describe the clinical findings of dyspnea or cough without attention to the actual cause. This may lead to inappropriate therapy. Other similar terms include chronic obstructive lung disease (COLD) and chronic airway obstruction (CAO).
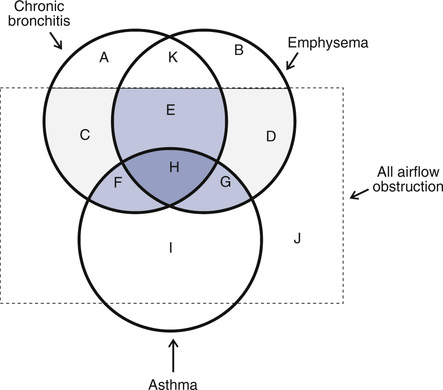
Emphysema, chronic bronchitis, and asthma overlap to varying degrees (shaded areas). Chronic obstruction in small airways and all airway obstructive diseases (large dashed square) also overlap. A, Patients with chronic bronchitis but no airflow obstruction. B, Patients with anatomic changes related to emphysema but no obstruction. C, Patients with chronic cough and airflow obstruction. D, Patients with emphysema and obstruction, as demonstrated by spirometry. E, Combined chronic bronchitis and emphysema, commonly occurring in the same patient, as a result of cigarette smoking. F, Combined chronic bronchitis and asthma. G, Combined emphysema and asthma. H, Combined asthma, chronic bronchitis, and emphysema. I, Patients with asthma manifested by reversible obstruction (spirometry or peak flow). J, Other forms of airway obstruction, including cystic fibrosis, bronchiolitis obliterans, or upper airway abnormalities (e.g., vocal cord dysfunction), are not considered part of COPD. K, Patients with cough and morphologic evidence of emphysema but no obstruction. (Modified from American Thoracic Society. Standards for the diagnosis and care of patients with chronic obstructive pulmonary disease. Am J Respir Crit Care Med. 1995; 152:S77-S120.)
Emphysema
The chest x-ray film of a patient with emphysema shows flattened diaphragms and increased air spaces. The lung fields appear hyperlucent (dark) with little vascularity. The heart appears to be hanging from the great vessels (Figure 1-3). Computerized tomography (CT) scans, especially spiral CT scans, show a three-dimensional picture of enlarged air spaces and loss of supporting tissue. CT scans also delineate whether the emphysematous changes are localized or spread throughout the lungs.
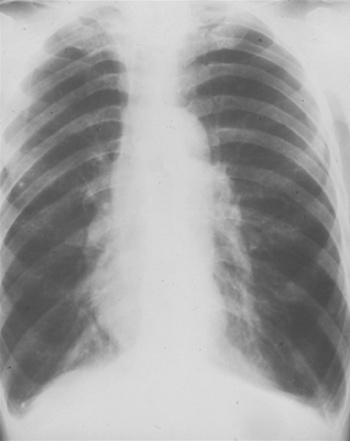
The physical appearance of the chest confirms what is shown radiographically. The chest wall is immobile with the shoulders elevated. The diameter of the chest is increased in the anterior-posterior aspect (so-called barrel chest). There is little diaphragmatic excursion during inspiration. Intercostal retractions may be prominent. Accessory muscles (neck and shoulders) are used to lift the chest wall. Breath sounds are distant or absent. Patients may need to support the arms and shoulders to catch their breath. Breathing is often done through pursed lips in an attempt to alleviate the sensation of dyspnea (Figure 1-4).