CHAPTER 157 Implantable Hearing Aids
Hearing aids are the principal means of auditory rehabilitation for patients with sensorineural hearing loss (SNHL). Hearing aids may also play a role in the management of patients with conductive hearing losses (CHL), particularly losses that result from pathologies that are not amenable to medical and surgical treatment. Improvements in signal processing capabilities and increasing miniaturization of hearing aids have increased their acceptance among patients. Hearing aids remain a “tough sell” to many patients who could potentially benefit from them, however, owing to lack of sufficient perceived benefit, expense, complications of external auditory canal occlusion, and cosmetic concerns. In a restaurant, multiple competing sound sources, such as conversations, the ventilation system, and music, can undermine the ability to detect and resolve speech cues. Many other patients simply cannot wear a hearing aid because of problems with the external auditory canal, chronic infections, or extreme discomfort. Although newer hearing aid technologies have tried to address many of these difficulties, many patients still find that hearing aids fall short in meeting expectations in a variety of situations (Box 157-1). Partly for these reasons, less than one in eight eligible adults actually use a hearing aid.1
These inherent limitations in hearing aids, combined with the social stigma of their use, have led to the development of implantable hearing aids (Figs. 157-1 and 157-2). Implantable hearing aids offer patients with hearing loss several potential advantages over conventional hearing aids, including increased gain and dynamic range, reduced feedback, reduced maintenance, improved appearance, and freedom from ear canal occlusion. These potential benefits must be weighed against the possible downsides of their use, including the risk of surgical implantation, difficulty of maintenance, and increased initial device cost (Table 157-1). Complicating this risk-benefit calculation is the uncertain future of corporations marketing and supporting various implantable hearing aids. Before discussing each of the implantable hearing aids currently available or in development, it is important to review in more detail why there is a desire for their implementation in the first place.
Limitations of Auditory Rehabilitation Using Traditional Hearing Aids
Physical Factors
Insufficient Gain
For patients with severe to profound hearing loss, sound amplification (or gain) is a primary concern. For a patient with air-conduction thresholds of 80 dB hearing level (HL) to perceive quiet sounds at a normal threshold of 0 dB HL, a hearing aid must amplify sound intensity by 80 dB, generating a 10,000-fold increase in sound pressure wave amplitude and a 100,000,000-fold increase in sound power intensity.2 This represents the limit of existing traditional hearing aids. The maximum amplification at 1 kHz for a behind-the-ear (BTE) Phonak SuperFront PPCL4 power digital hearing aid is about 75 to 82 dB.3 Gain generally scales with hearing aid size, so that cosmetically less obtrusive hearing aids offer less gain. The maximum gains for digital in-the-ear (ITE), in-the-canal (ITC), and completely-in-canal (CIC) hearing aids currently are about 55 to 65 dB, 45 to 55 dB, and 35 to 50 dB.3
Poor Appearance
Many patients reject hearing aids because of the social stigma of being seen as old or infirm. Eight percent of hearing aid candidates wearing the less noticeable ITC hearing aids listed poor cosmetic appearance as a major contributing factor in their decision not to use a hearing aid.4 Hearing aids are difficult to conceal in patients who are balding or who wear their hair short. Miniaturization of electronics continues to improve hearing aids in this regard, and CIC hearing aids are essentially invisible to the casual observer. Miniaturization comes at the cost of lower gain, more feedback, and higher cost, however. Ultimately, battery size becomes the limiting factor, with smaller batteries costing more and requiring more frequent replacement.
Poor Transduction Efficiency
Loss of energy because of impedance mismatching and transduction losses is another inherent drawback of conventional hearing aids. The mechanical impedance (change in pressure for a given displacement flow) of the air-filled external auditory canal differs from that of the fluid-filled cochlea. Without a middle ear mechanism, most of the acoustic energy striking the stapes footplate reflects back into the air. When the tympanic membrane and ossicular chain are functioning normally, they act as an impedance-matching transformer by virtue of the relative areas of the tympanic membrane and stapes footplate, and by the lever action of the ossicular chain.5 The relatively large-displacement, low-pressure movements of air against the tympanic membrane are transduced to the relatively small-displacement, high-pressure movements of the footplate. Except for bone-conducting hearing aids, all traditional hearing aids use a speaker to output an (amplified) acoustic wave into the air of the external auditory canal. When this middle ear apparatus is malfunctioning, as occurs in otosclerosis, tympanic membrane perforation, or a canal wall down mastoidectomy, traditional hearing aids must overcome the impedance mismatch. The result is reduced effective gain, increased distortion, or both.
Human Factors
Recruitment and Compression of Dynamic Range
A primary source of frustration of patients with SNHL is reduced speech intelligibility. The common complaint, “I can hear, but I cannot understand,” underscores this point. This problem relates to abnormal frequency resolution and aberrant patterns of growth in loudness, each of which reduces speech intelligibility in challenging listening conditions such as noisy surroundings.6,7
SNHL also imposes severe constraints on the dynamic range of perceived sound. For normal listeners, the dynamic range from sensing soft sounds to the loudest tolerable noise is more than 100 dB. Within this wide dynamic range of hearing lies an approximately 35-dB dynamic range of conversational speech. In contrast, the dynamic range of patients with SNHL is often narrowed by an increase in the threshold of audibility and a lowering of the ceiling of tolerance to high-intensity sounds. This compaction of dynamic range leads to “recruitment,” an abnormal growth in loudness as sound intensity increases.8,9 What sounds normal for someone with normal hearing may be too soft for someone with recruitment, whereas what is too loud for someone with normal hearing is also too loud for a patient with recruitment. In effect, there is a much narrower range of sound intensity that a patient with recruitment can tolerate. Further adding to the difficulty, recruitment is observed in frequencies that are most impaired, in the high frequencies, which also carry crucial information for speech understanding.
Recruitment remains one of the principal challenges of hearing aid rehabilitation, and is responsible for a common phenomenon most clinicians dealing with hearing loss have witnessed or experienced: At average speaking levels, an individual with recruitment may ask a speaker to talk more loudly, yet with even a slight increase in intensity the speech becomes intolerably loud, and the speaker is told not to shout. Although many hearing aids can be programmed to prevent sound from amplifying into a range that is uncomfortable, even the most advanced aids cannot fully replicate the complex, nonlinear response patterns of a healthy cochlea.9
Disordered Perception of Pitch and Sound Localization
All of the aforementioned factors play a role to various degrees in patients, and combine to increase the noncompliance rate in hearing aid users. For most patients, these are the principal reasons why hearing aids are unacceptable.10 With all these challenges concerning conventional hearing aids, it is not surprising that many hearing aid users feel frustrated when hearing aids fail to perform to expectations.
Promise of Implantable Hearing Aids
It is widely accepted that implantable hearing aids should provide distinct advantages over conventional hearing aids, including better cosmetics, improved fidelity, broader frequency response, less distortion, reduction or elimination of feedback, and better speech understanding, without reducing residual hearing, limiting patient activities, or predisposing patients to infection.11,12 In other words, implantable hearing aids should be at least as good as, and preferably better than, the best available noninvasive method of hearing rehabilitation: binaural amplification with the best available aid.13
Although significant strides have been made in many of these areas, implantable hearing aids have yet to deliver on all these features. There also remain questions regarding candidacy for implantation and willingness of patients to accept the costs and surgical risks. As noted by Junker and colleagues,14 the number of patients in a fairly typical population of hearing-impaired individuals who would be considered realistic candidates is limited, on the order of 0.09%. The market for implantable hearing aids may not be sufficiently large to support the current array of device manufacturers. The 2002 financial failing of Symphonix Corp., the first company to gain U.S. Food and Drug Administration (FDA) approval and market their implantable middle ear hearing aid in the United States, strongly underscores this point, as do the subsequent withdrawal of the Soundtec and TICA devices described subsequently in the review of technologies.
Middle Ear Implants
Basic Design Features
Transducer Design
Conventional hearing aids function by receiving acoustic energy through a microphone, processing and amplifying the signal (in either an analog or a digital fashion), and transmitting the signal through a speaker adjacent to the eardrum. This amplified sound travels through the normal auditory pathway of the tympanic membrane and ossicles to the inner ear. Implantable middle ear hearing aids also employ a microphone that senses sounds and a signal processor that amplifies and alters the sound signal, just as in a conventional hearing aid. From here, they differ from conventional hearing aids. In one of several mechanisms unique to each device, the implantable middle ear hearing aids convert the electric signal into a mechanical energy that is coupled directly to the ossicular chain (see Fig. 157-1).The critical components of these systems are the transducer, which enables the device to output a signal that can be received by the ossicles, and the mechanism by which the transducer is coupled to the ossicles, which can either be a direct or indirect contact.15 Variations in these critical components distinguish the various implant designs (see Fig. 157-2). Goode and colleagues11 previously outlined the history of the development of implantable middle ear hearing aids.
Implantable middle ear hearing aids may also incorporate an electromagnetic transducer. These transducers generate a magnetic field using a coil carrying a current that encodes the microphone output. This magnetic field from the coil can cause motion in a ferromagnetic substance that is nearby, causing it to vibrate.15 As applied to middle ear implants, sound received from a microphone is converted by the signal processor, amplified, and sent to the electromagnetic transducer. The electromagnetic field that is set up vibrates a ferromagnetic unit coupled directly to the ossicles. In contrast to the piezoelectric unit that must be in direct contact with the ossicles, electromagnetic transducers need only to be near the ossicles, which have attached to them the ferromagnetic device that transmits the auditory signal to the ossicles.
These two types of amplification have different advantages for use in an implantable hearing aid. Electromagnetic transducers do not directly contact the ossicular chain, but rely on electromagnetic transmission to a ferromagnetic unit that is attached to the ossicles. Electromagnetic transducers can be packaged in a smaller housing, an important factor in the ear. In contrast, piezoelectric devices are larger and couple directly to the ossicular chain. The advantage of this type of transducer is its ability to deliver more distortion-free amplification directly to the ossicular chain.15 In either event, to accommodate patients with moderate to severe SNHL in the 55- to 90-dB hearing level range, any implant, whether electromechanical or piezoelectric, should be able to deliver a maximum mechanical stimulation output equivalent to 120 to 130 dB sound pressure level (SPL).16,17 How each implant achieves this output level is described subsequently.
Ossicular Coupling
As noted by Huttenbrink,18 to obtain optimal efficiency in implantable hearing aids, they must adapt to the mechanics of the middle ear and ossicular chain. As the third ossicle in the chain, and the one in continuity with the inner ear, the motion of the stapes is crucial in sound transmission. The stapes moves in a piston-like fashion.19 The ideal motion of the stapes by a prosthesis would be in this piston-like fashion. Any implant that directly attached to the stapes would function best by mimicking this motion.20,21
Electromagnetic Middle Ear Implantable Hearing Aids
Vibrant Soundbridge
The Vibrant Soundbridge is a semi-implantable hearing aid that was first introduced in 1997 (Fig. 157-3). Since its introduction in 1997, more than 2300 patients have been implanted worldwide.14 The device was approved for commercial availability in Europe in 1998, and in the United States in 2000. The electromagnetic transducer uses a proprietary vibrating ossicular prosthesis (VORP), which typically is attached to the long process of the incus. The VORP contains a rare earth magnet surrounded by a receiving coil, a demodulator package, a conductor link, and a proprietary floating mass transducer (FMT).22,23 The transducer was developed by Ball, and incorporated prior conceptual work performed by Goode and colleagues.11,22,24 The VORP is electrically connected to the internal receiver.25 These internal components are coupled to the audio processor via a telemetry link, similar to a cochlear implant. The processor, which is worn behind the ear, contains the microphone, audio processing electronics, magnet, telemetry transmission equipment, and room for a standard 675 zinc battery to power the implant.
The Vibrant Soundbridge is indicated in patients with moderate to severe SNHL and desire for an alternative to an acoustic hearing aid (Fig. 157-4).23 Studies have indicated that it may be suitable for patients with hearing loss of 70 dB pure-tone average (PTA) hearing thresholds.26 When first introduced, the externally worn audio processor was an analog device that incorporated a wide dynamic range compression. Because of inadequate gain of the device, particularly at lower frequencies and with low sound levels,27 a newer, more powerful digital audio processor was introduced in 1999. Studies done shortly after its introduction failed to show superior hearing results compared with conventional aids, although patients preferred the device, in part because of ability to wear it for a much longer time without discomfort.26
The device typically is implanted using a standard transmastoid, facial recess approach to the middle ear. The VORP is crimped into the long process of the incus. The internal receiver is placed in a bony trough in the retrosigmoid bone several centimeters behind the ear, in a location similar to the receiver placed during cochlear implantation. One potential drawback of this design concerns the size of the VORP. Because of the confined space between the eardrum and the incus, the dimensions and mass of the FMT are limited, restricting its output.28 A second potential drawback is erosion of the incus long process, owing to ischemia at the clip attachment site. Modifications of the typical surgical approach allow treatment of mixed hearing losses resulting from otosclerosis or ossicular erosion or agenesis through direct placement of the VORP on the stapes superstructure, round window, or oval window.29–32
Owing to its primacy in regulatory approval processes and patient availability, to date more clinical data are available for the Vibrant Soundbridge than for all other middle ear implants. Results from the phase III clinical trial of 53 patients were sufficiently favorable to merit FDA approval of the device (phase I trials typically test feasibility and safety in a small group of subjects, phase II trials typically test effectiveness in a slightly larger group, and phase III trials assess safety and effectiveness in a large group).23 Patients in the study were analyzed with conventional analog hearing aids, digital hearing aids, and the Vibrant Soundbridge. Standard audiologic tests included pure-tone air-conduction and bone-conduction thresholds (250 to 8000 Hz), functional gain, speech recognition tests, the Profile of Hearing Aid Performance, and the Hearing Device Satisfaction Scale.
Although one patient had a shift of 18 dB after device placement, the effect of implantation on residual, native hearing was believed not to be significant (<10 dB PTA at 500 to 2000 Hz). This finding corroborated earlier studies that showed little to no effect of the presence of the FMT on hearing sensitivity.33 When compared with presurgical conventional hearing aid test scores, the implant showed a statistically significant improvement in functional gain at all frequencies and greater than 10 dB improvement at 2 kHz, 4 kHz, and 6 kHz. No significant difference was noted in speech recognition (NU-6 word list) between the preoperative hearing aid and the implant. On the revised speech reception in noise score, 7 patients (13%) showed significantly better scores, 70% showed no change, and 17% had worsening of their scores. As an entire group, there were no significant differences between the conventional hearing aid and implant group test scores on speech reception scores. Significant improvements were noted in the implanted group on the two self-assessment questionnaires (Profile of Hearing Aid Performance and Hearing Device Satisfaction Scale).
The European experience with the Vibrant Soundbridge seems similar to the experience in the United States.24,25,34 A multicenter study from Europe, which included 63 patients, focused on the gain provided by the implant and attempted to assess the quality of the amplified speech.25 In contrast to the U.S. FDA phase III study, the European study did not compare results with a conventional hearing aid, but used the unaided condition as a comparison. The study noted a large variability in gain for each patient. For most patients, the greatest gain was in the 1- to 2-kHz region, where the transducer has its resonance.22 The authors reasoned that an inefficient coupling in some patients (e.g., the FMT may be loose) may be responsible for the wide variability seen, and highlighted the importance of effective placement of the VORP on the incus.
When the authors compared gain at threshold and gain at normal speech, there was only a small difference noted (6 dB), indicating that the gain is effective at normal speech levels. The authors also concluded that the amplified sound from the Vibrant Soundbridge had adequate quality for speech intelligibility. Overall, the authors believed that there was clear benefit from the Vibrant Soundbridge, but there was a subgroup of patients with low gain, secondary to the limited bandwidth of the amplified signal. Other work from this same multicenter European group has shown a significant improvement in communication in various listening conditions with the Vibrant Soundbridge compared with conventional aids.24,34
A more recent report relates the Belgian experience with the Vibrant Soundbridge.35 In a study of 13 patients, the average functional gain between 500 Hz and 4000 Hz was 30 dB, similar to prior European and American studies published. The authors noted, however, that not all of their patients studied were able to afford the best possible digital hearing aid preoperatively, and they could not adequately compare satisfaction levels. The cost of the implant is severalfold higher than for the top-of-the-line digital aids, and it is likely that these patients could not afford the implant on their own. The authors reported high satisfaction of all the users of the device, and the “impressive” functional gains, particularly at higher frequencies.
One study that has specifically addressed the functional gain of the Vibrant Soundbridge with conventional hearing aids is from Todt and associates.36 Examining five patients first fit with an analog and then digital conventional processor, the authors compared results with a digital processor of the Vibrant Soundbridge. As with previous studies, no significant differences were noted in underlying, residual hearing as a result of implant placement. Their data showed a significant improvement of hearing compared with conventional aids in quiet (approximately 10 dB PTA), and a better functional gain in noise for the Soundbridge compared with conventional aids. Subjective outcomes of a hearing aid questionnaire (Abbreviated Profile of Hearing Aid Benefit) also showed significant improvement of the Symphonix implant compared with conventional aids. As with other studies, the greatest gains were noted in the higher frequencies.
MET (Middle Ear Transducer) and Carina
The Otologics MET (Middle Ear Transducer) is an electromechanical middle ear hearing aid using a mechanism initially developed by a group headed by Fredrickson in collaboration with Storz Instrument Co. It is now manufactured by Otologics LLC.16 Although the original MET (on which most data reviewed here are based) was semi-implantable, that design has been replaced by a fully implantable version marketed as the Carina (Fig. 157-5).
The MET implantable unit consists of the transducer coupled to an electronic receiver.16,37 The transducer vibrates a probe that is in direct contact with the incus. For the semi-implantable version, two external devices were developed. The device initially used in clinical trials was a modified version of Oticon’s JUMP-1 hearing aid (Oticon Inc., Copenhagen, Denmark), and comprised a BTE signal processor, microphone, amplifier, and battery. This device was attached to a transmitter coil via a short cable. A newer unit housed the transmitter coil together with the BTE components. These semi-implantable designs enabled the MET to deliver the equivalent of greater than 135 dB SPL over a frequency range of 250 to 6250 Hz.16 This output should theoretically provide adequate gain for patients with moderate to severe SNHL.
Placement of the MET Ossicular Stimulator requires a general anesthetic and approximately a 2-hour operation (Fig. 157-6). Using a standard, postauricular approach to the mastoid cortex, the root of the zygoma, posterior canal, and linea temporalis are clearly delineated (see Fig. 157-6).16 A limited mastoidectomy (antrostomy) is performed directly superior to the external canal and inferior to the tegmen tympani using a 6-mm bur, and extended until the incus body (approximately a 10-mm width) and head of malleus are exposed. When complete, a mounting system is attached over the opening using bone screws. The MET Ossicular Stimulator is maneuvered into the mounting system with the transducer probe tip fully retracted. A laser is used to make a small hole in the incus body to accept the probe tip. The tip is advanced into position using a micrometer screw, and ultimately becomes firmly attached to the incus from fibrous tissue. The receiver capsule and transducer electronics are placed in a recess drilled in the mastoid, similar to cochlear implant electronics.
Ultimately, 282 patients were implanted with the semi-implantable MET in Europe and the United States during phase I and II trials.16 Pure-tone audiometry, speech recognition, and subjective assessment of benefit were tested before the surgery and 12 months after. Group mean postoperative bone-conduction and air-conduction thresholds were not significantly changed from preoperative levels compared with a well-fit digital hearing aid used preoperatively. A slight (approximately 5 dB) increase in CHL was attributed to mass loading by the transducer on the ossicles. Sufficient gain was available to make the device useful for subjects with moderate to severe SNHL. Speech and subjective assessment of patient preference indicated that patients did as well or better with the MET compared to their “walk-in” aid or the standardized digital hearing aid (Fig. 157-7).37
The Otologics Carina fully implantable hearing system is similar to the original MET except that it requires no external components apart from a charger and remote.38 It uses a subcutaneous microphone rather than an external microphone, and it is designed to work for waking hours of 1 day between charging sessions that require 1 hour. Phase I trial results in the United States showed 10 to 20 dB of functional gain across audiometric frequencies at 3 months postoperatively.39,40 Pure-tone bone averages were minimally changed from preoperative values. Pure-tone air averages and monaural word recognition scores were initially slightly worse with the device than measured preoperatively with the walk-in aid, but patients reported greater subjective benefit with the implanted device.
Within 12 months postoperatively, a high rate of device failure was observed, leading to explantation in 5 of the 20 adult study subjects as of early 2008. Failure modes included partial device extrusion, inability to communicate to external components, and failure of the recharging mechanism. Even among devices that did not require explantation, degradation in hearing performance commonly occurred at approximately 6 months after implantation and was attributed to movement of the subcutaneous microphone. These observations led to a redesign and revision in surgical technique emphasizing creation of a well adequately deep to prevent device extrusion and a tie-down mechanism to prevent microphone migration. Intraoperative measurement and optimization of ossicular coupling compression resulted in more consistent and better coupling efficiency of the transducer to the ossicles.41,42
The Carina has received the CE Mark for clinical use in Europe, and phase II trials are under way in the United States. As of early 2008, more than 50 patients had been implanted with the redesigned device, and the failure modes identified during the phase I trial had not recurred.39 Variations on the size and length of the ossicular coupling have expanded the application of the Carina to patients with aural atresia and ossicular discontinuity, via direct attachment of the device on the stapes capitulum, stapes footplate, and round window.41,43
Piezoelectric Middle Ear Implantable Hearing Aids
Esteem-Hearing Implant
A piezoelectric bimorph placed on the malleus head can effectively use the tympanic membrane as a microphone, offering a viable alternative to a subcutaneously implanted microphone. Studies in cadaveric temporal bones have shown that such a device had a dynamic range of 80 dB at 1000 Hz, with precise ossicular tracking out to 4000 Hz.44 The Esteem-Hearing Implant developed by Envoy Medical (formerly called the Envoy device and developed by St. Croix Medical, Inc., Minneapolis, MN) is a fully implantable piezoelectric device that uses this approach (Fig. 157-8). It received the CE Mark in Europe in 2006 and as of 2008 was in a phase II clinical trial in the United States.
Similar to all passive transducers, piezoelectric devices are bidirectional. The Esteem/Envoy uses a piezoelectric crystal to convert malleus vibrations (owing to sound waves impinging on the tympanic membrane) into a voltage encoding sound. After amplification, this electric signal is applied to a piezoelectric output transducer, which converts from the applied voltage to mechanical vibration coupled to the stapes. Power is provided by a nonrechargeable lithium-iodine battery designed to last 5 years between replacements, according to the manufacturer.45 Programming and control of the device are accomplished through a radiofrequency transcutaneous link to a hand-held device or laptop computer.
The Esteem/Envoy is designed for patients with moderate to severe hearing loss. Indications include age 18 years or old, mild to severe (35 to 85 dB) SNHL between 500 Hz and 4000 Hz in the implanted ear that is equal to or worse than the hearing loss in the nonimplanted ear, a healthy ear with normal pneumatization and adequate space for device implantation on computed tomography (CT), normal tympanometry, and speech discrimination 60% or greater (Fig. 157-9). A phase I trial in the United States and Germany was completed in 2003. By 1 year after implantation, three of seven subjects continued to use the implant, three had been revised and then explanted, and one was awaiting revision surgery. For the three subjects with functioning implants, there was no significant change in bone thresholds, and the 4-frequency (500 Hz, 1000 Hz, 2000 Hz, and 3000 Hz) average functional gain was 17 dB ± 6 dB, which was comparable to conventional hearing aids except at 3000 Hz, where the Envoy performed less well than conventional hearing aids. The decline in performance was attributed to a gradual ingress of moisture in the transducers.46
Implantable Bone-Conduction Hearing Aids
Conductive and mixed hearing losses are highly prevalent disorders that often may be effectively treated using standard surgical techniques or rehabilitated with traditional hearing aids. For a variety of reasons, a large subset of these patients are not suitable surgical candidates for correction of their deficit, however, or are unable to tolerate a conventional hearing aid. This group includes patients with chronically draining ears despite multiple corrective attempts, patients with discomfort from the sound levels required from a traditional hearing aid, and patients unable to tolerate a hearing aid because of a large mastoid bowl or meatoplasty after chronic ear surgery. Additionally, patients with otosclerosis, tympanosclerosis, or canal atresia, and who have a contraindication to surgical repair may also fall into this category of patients who defy traditional attempts at hearing restoration. Patients who have undergone external auditory canal closure after extensive skull base surgery also are not amenable to traditional hearing aid rehabilitation. The osseointegrated BAHA device was originally intended for this group of patients.47–49 The BAHA device now has also been used extensively for patients with unilateral deafness with good results.49
Conventional bone-conducting hearing aids have been advocated as an alternative for patients with conductive or mixed hearing loss who cannot benefit from a traditional hearing aid. The utility of these devices is constrained, however, by several physical factors. Because the bone conductor must be applied with steady pressure to the mastoid cortex (usually via a headband or eyeglasses), patients often experience pain, headache, and skin irritation at the contact site. Sound fidelity is limited by soft tissue attenuation, variable placement of the vibrator, and flaccidity of the securing device (usually eyeglass frames).50,51 Coupling the bone vibrator to an osseointegrated implant, as originally performed by Tjellstrom and colleagues51 at the Institute of Applied Biotechnology in Sweden in 1977, circumvents many of these drawbacks. This new generation of osseointegrated conductive hearing aids now offers an effective alternative to hearing rehabilitation for this difficult subset of hearing-impaired patients.
Osseointegration
Background
In the 1960s, Branemark and associates52 from Gothenburg, Sweden, discovered that titanium had a unique ability to become firmly anchored in bone without interposed soft tissue, while at the same time achieving a reaction-free epithelial penetration of the implant. In these permanent fixtures, Branemark presciently foresaw the ability of implanted titanium to support functional prostheses, and termed this state osseointegration. Osseointegration is considered to be successful if the implant became rigidly anchored into adjacent bone with no reaction at the implant-bone interface.53 This concept was initially applied to dental implants, but in 1975, Branemark’s group began studying the possibility of creating a skin-penetrating implant. By the 1980s, Branemark and colleagues, including Albrektsson, Jacobsson, and Tjellstrom,54 had developed a clinical program with percutaneous titanium implants to provide an anchor for facial prosthetics (pinna, eye, or nose), and an attachment for a BAHA device.
Biophysical Principles
Implant Material
One initial determinant of successful osseointegration is the chemical composition of the implant. Titanium has survived osseointegration’s “test of time,” and now represents the standard by which all other biomaterials are measured. Titanium is available commercially as “pure” titanium, which is 99.75% pure titanium, and titanium alloy, containing 90% titanium, 6% aluminum, and 4% vanadium. Titanium, similar to all other metals, forms several types of oxides on exposure to oxygen. In contrast to many of its metallic counterparts, however, titanium has the ability to create a tightly bonded and corrosion-resistant oxide layer on the surface of the implant. This oxide coating of the implant is in contact with the host tissue, which seems most important in determining the biocompatibility of titanium.55,56 Because the implant may be worn for several decades or longer, the toxicity and carcinogenicity of the oxide coating take on particular importance.53 Reports have shown that titanium is superior to stainless steel, lacking steel’s high potential for corrosion or the toxicity of its components.56 To date, pure titanium has shown none of these adverse sequelae, and continues to represent an ideal implant material.55
Implant Design
Implant microstructure and macrostructure also impact the likelihood of osseointegration. Although a rough-surfaced implant would theoretically better distribute a weight-bearing load, a rough, porous surface less than 100 µm would greatly increase the surface area and increase the risk of implant corrosion, even with a relatively corrosion-free material such as titanium. According to Eriksson and Branemark,53 it is important that the microarchitecture of the implant surface features a topography with micropits that fit the dimensions of the cell membrane and large biomolecules; this facilitates direct bonding of the implant’s oxide surface with the bone matrix. Parametric studies also show that threaded, screw-shaped implants have a greater likelihood of osseointegrating, and seem to distribute stress more efficiently than T-shaped or cylindric types of implants.53,57 The threaded design success is probably most critical in conferring stability in the initial healing phase. If an implant is not absolutely stable after placement, connective tissue may form between the implant and the bone, preventing sufficient osseointegration.
Biology and Histology
Various studies have addressed the biologic correlates of osseointegration. First, there must be direct contact between the bone matrix and the implant, without interposed fibrous or soft tissue.52 Pure (99.75%) titanium has shown this capability histologically, whereas titanium alloys, despite some clinical success, have not. Additionally, for successful osseointegration to occur, there should be no evidence of inflammation at the implantation site, including an infiltrate surrounding the implant or evidence of osteolysis with bone loss.58 Pure titanium, as opposed to alloyed titanium, has also been shown to be superior in this regard.51,55 Lastly, there should ideally be no evidence of a connective tissue capsule on the implant surface, although studies have shown that an implant with a capsule less than 30 µm in thickness may achieve osseointegration.59 By all the above-mentioned criteria, pure titanium seems superior in every category for successful osseointegration compared with alloyed titanium. This superiority is borne out by several clinical studies as well.50,60,61 Most authors continue to recommend pure titanium for implant material.
Radiologic Implications
A chief disadvantage of any metallic implant is interference with subsequent radiologic studies. Many osseointegration implant candidates are considered for implantation because of surgical defects caused by tumor extirpation, or other medical problems that require long-term surveillance with repeated postoperative CT or MRI scans. The presence of an implant that diminishes the quality of these scans, by introducing echo artifact, takes on increased importance. Pure titanium produces little degradation of images on either CT or MRI. Because of backscatter emissions, a radiation dose delivers 11% of the radiation dose in front of the implant, and 89% behind it.62 This scatter effect produces little effect on the quality of CT images produced. Similarly, MRI is not contraindicated with the presence of a titanium implant. Any attached components must be removed before the MRI scan, however.
Operative Technique
Complete insertion of osseointegrated implants for the BAHA generally is done in a single stage (Figs. 157-10 and 157-11).63 Although most surgeons perform the BAHA application in the operating room, there are reports of performing the procedure in an outpatient clinic setting.64 Because of the importance of the implant’s surface-bone interface, the surgeon must use a meticulous and precise method of implant insertion. This method sets the stage for osseointegration with minimal surrounding tissue damage. Only a single implant is needed for a BAHA. According to Branemark’s original studies, insertion of the implant must be performed meticulously and as gently as possible to minimize thermal damage to surrounding bone.65 This insertion is optimally performed with a high-torque drill at slow speeds (1500 to 3000 rpm) with sharp drill bits. The use of disposable drill bits avoids the possibility of worn cutting surfaces that damage the implant bed. Maximal irrigation is employed to prevent temperature elevations greater than 33° C. Previous studies have shown that heating tissue to 47° C for 1 minute obviates osseointegration because osteocytes are lost. High-speed drilling typically used in surgery causes temperature elevations to 89° C.66
During the surgery, the postauricular skin surrounding the implant must be prepared for percutaneous attachment. The cutaneous-implant interface, as originally conceived by Branemark, is based on biologic principles found elsewhere in the body and throughout nature. Teeth and nails in humans, and talons, tusks, or claws as found in other species, all interface with a thin, firmly attached cutaneous or mucosal border, with little to no hair. This arrangement limits tissue mobility and increases stability of the area, while inhibiting the penetration of microbes with subsequent inflammation or infection.67,68
To recreate surgically a reaction-free implant-cutaneous junction, two preconditions must be met: The skin penetrated by the implant must be hairless, to facilitate keeping the implant site clean, and subcutaneous tissue should be aggressively thinned to minimize skin mobility in relation to the implant. The dermal layer is allowed to heal directly on the mastoid periosteum, creating a solid, immobile foundation for the implant.63 To achieve these prerequisites in the temporal bone, hair-bearing skin overlying the implant is aggressively thinned, and hair follicles are removed from the level of the dermis. Some authors originally advocated removal of skin and replacement with a full-thickness skin graft, but this was associated with an increased incidence of skin breakdown surrounding the implant.69 More recently, a dermatome has been developed specifically for use with the BAHA that has decreased the incidence of skin necrosis.70 The final skin flap or graft should be less than 1 mm thick at the implant site and adjacent area. The edges of the surrounding skin flaps are sutured down to the periosteum to minimize tension on the flap.
Bone-Anchored Hearing Aid in Young Children
The manufacturer of the BAHA recommends the osseointegration procedure in children older than 5 years. For children younger than 5 years in need of bone-conduction hearing rehabilitation, the BAHA-Softband is available.71 Similar to a conventional bone-conduction hearing aid, the Softband allows bone-conduction hearing by coupling a standard BAHA device to a soft headband, providing hearing that is nearly equivalent to the osseointegrated hearing aid.
Some groups have advocated a traditional osseointegrated implant in this younger age group.72,73 One complication that must be overcome, however, is the higher incidence of nonintegration in this younger age group, reported to be 15%.74 Several recommendations have been proposed to avoid this complication. One is to use a two-stage procedure, whereby the implant is placed into the bone without the abutment, and the skin closed directly over this.72,73 At a later second stage (6 months later if the skull is particularly thin), the skin flap is re-elevated, the abutment is placed, and the remainder of the procedure is finished as with the single stage. One can also leave the abutment in place during the first stage.72 During the second stage when elevating the skin flap, a scalpel is used rather than the dermatome when the skin is tented by the healing screw, or the flap is infiltrated with local anesthetic to balloon it before using the dermatome. A final recommendation is to create an extremely thin flap to prevent cellulitis during the healing period.72 Studies have shown that equal hearing results can be obtained when applying this staged approach in children younger than 5 years.73
Complications Associated with Osseointegration
The most frequently encountered complication associated with osseointegration is a soft tissue reaction at the percutaneous implant site. Soft tissue and skin reactions associated with percutaneous attachments were studied during the initial development phases of osseointegration technology. In one of the first large studies to address this issue specifically, Holgers and coworkers75 monitored the soft tissue reactions of 67 patients who underwent osseointegrated implantation. With an average follow-up of 3 to 96 months, 14 of the 67 patients eventually developed adverse tissue reactions. One patient required removal of the abutment because of an infection, 5 patients developed redness and moisture at the abutment site requiring surgical revision (usually subcutaneous tissue thinning), 2 patients had redness with moisture requiring only local treatment, and 13 patients had developed minor erythema requiring temporary local treatment.
Jacobsson and colleagues76 also published a case report of a patient who developed a soft tissue infection at an abutment site, eventually necessitating implant removal. Despite the presence of an ongoing infection, the implant showed histologic evidence of osseointegration. Niparko and associates61 reported the first U.S. results with extraoral osseointegrated implants in 14 patients, noting a 3.5% incidence of symptomatic soft tissue reactions, with no implant extrusion. In the largest series to date, Tjellstrom and Hakansson63 reported implant results on 456 patients, noting a 3.4% incidence of adverse tissue reactions; 3 patients required implant removal for infection, 3 required revision surgery for continued erythema and moisture, and 23 had tissue reactions requiring local treatment only. In the first large U.S. survey of BAHA devices, soft tissue complications were minimal and consisted of local inflammation at the percutaneous-implant junction (three patients).47 A study of 149 consecutive cases showed that skin overgrowing the abutment was the most common complication (7%), which occurred on average 1 year after the initial surgery.77
Observations from these studies indicate that soft tissue reactions with percutaneous implants are largely prevented by soft tissue reduction and attachment of dermis to subjacent bone, to achieve immobility, and patient compliance with routine cleaning of the abutment site and close monitoring of skin attachment of the implant to the abutment. Complications other than adverse skin reactions are rare. Osseointegration failure is the second most common complication of the technique. In the study by Tjellstrom and Hakansson,63 of 149 patients, 5 were considered failures; 4 failed to osseointegrate, whereas the fifth implant was lost through direct trauma. The first large U.S. series had only one patient with osseointegration failure,47 whereas a more recent study by House and Kutz77 had a 3.4% rate of implant extrusion. A rarely described complication is an intracerebral abscess.78 Overall, studies consistently show that when Branemark’s original surgical guidelines are followed, osseointegrated implantation is associated with high surgical success, and a low rate of morbidity and subsequent complications.
Results
In the largest series to date, Hakansson and colleagues79 reported on their 10-year experience in 147 patients who received the BAHA. Patients were divided into three groups based on their PTA bone thresholds: 0 to 45 dB, 46 to 60 dB, and greater than 60 dB. The authors noted a strong relationship between PTA thresholds and successful rehabilitation. In the group with the best cochlear reserve (PTA <45 dB), 89% of patients’ hearing was subjectively improved by the implant, whereas 8% believed their hearing was worse. Conversely, in the groups with progressively less cochlear function, 46 to 60 dB and greater than 60 dB, 61% and 22% of patients reported subjective hearing improvement. On average, speech discrimination scores improved from 14% unaided and 67% with a traditional hearing aid to 81% with the BAHA. This number increased to 85% if subjects with SNHL less than 60 dB HL were excluded, and to 89% if subjects with PTA less than 45 dB were excluded. Based on these results, the authors recommended that to be in consideration for a “high success rate” with the BAHA, patients should have a PTA less than 45 dB, although improvements in hearing should still be expected for a PTA of 60 dB (Table 157-2).
Table 157-2 Indications and Contraindications for Bone-Anchored Hearing Aid
Indications | Contraindications |
---|---|
Any patient using a conventional BC hearing aid | PTA BC thresholds (0.5-3.0 kHz) worse than 45 dB HL, SDS >60% |
Any AC hearing aid user with chronic otorrhea | Emotional instability, development delay, or drug abuse |
Any AC hearing aid user experiencing too much discomfort because of chronic otitis media or otitis externa | Age <5 yr |
Any AC hearing aid user experiencing uncontrollable feedback because of a radical mastoidectomy or large meatoplasty | |
Otosclerosis, tympanosclerosis, canal atresia with a contraindication to repair, such as in an only-hearing ear. Also, otosclerosis in combination with second, third, and fourth indications |
AC, air conduction; BC, bone conduction; HL, hearing loss; PTA, pure-tone average; SDS, speech discrimination score.
In describing the Birmingham experience, Stevenson and colleagues80 reported on the BAHA in seven children. Four children obtained improvements in PTA by 5 dB or more compared with their previous hearing aid, whereas four had changes in their thresholds of less than 5 dB. Only one of the six children tested for speech discrimination postoperatively had a significant improvement over his prior hearing aid, although the average speech discrimination score in this group was about 90% compared with 0% unaided. Liepert and DiToppa,81 citing the Edmonton experience in 15 patients, reported an average gain of 30 dB SRT with the NAS compared with unaided speech. In the 10 patients queried, all reported that the BAHA was “better” to “much better” than their previous hearing, with or without their prior hearing aid, in all situations. Overall, the BAHA seems to offer patients with a PTA less than 45 dB a high degree of hearing rehabilitation, and excellent patient satisfaction with the device.
In the initial review of the U.S. experience with the BAHA,47 the most common indications for implantation included chronic otitis media or draining ears and external auditory canal stenosis or aural atresia. Patients who had undergone skull base surgery and had complete closure of the external auditory canal were also included. Overall, each patient had an average improvement of 32 dB ± 19 dB with the use of the BAHA. Closure of the air-bone gap to within 10 dB of the preoperative bone-conduction thresholds occurred in 80% of patients, and closure to within 5 dB occurred in 60%. Nearly one third of patients showed “overclosure” of the preoperative bone-conduction threshold of the better hearing ear.
The BAHA Compact and the older BAHA Classic 300, on which these earlier studies are based, use an analog technology. A newer device, termed the Divino, featuring more contemporary signal processing has been developed. A more recent review comparing the Compact and Divino showed roughly equivalent results, with an average improvement over all frequencies of 27 to 28 dB for each device.82 For speech understanding in noise, an advantage of 2.3 dB for the BAHA Divino versus the BAHA Compact was found, if noise was emitted from a loudspeaker to the rear of the listener, and the directional microphone noise reduction system was activated. Subjectively, the Divino was rated better in terms of overall sound quality by the patients.82
The BAHA Cordelle is the device with the most powerful gain. Although the recommended levels of hearing loss for the traditional BAHA (Classic, Compact, and Divino) encompass a PTA bone-conduction loss at 500 Hz, 1000 Hz, 2000 Hz, and 3000 Hz at less than 40 dB HL, the body-worn BAHA Cordelle is recommended for patients with a more compromised cochlear reserve. Studies have shown that bone-conduction PTAs of less than 65 dB are acceptable for fitting.83 There is also the BAHA Intenso, and similar to the Divino, but with additional power for an intermediate sensorineural component.
Bone-Anchored Hearing Aid for Unilateral Sensorineural Hearing Loss
BAHA is gaining increasing popularity for the rehabilitation of unilateral SNHL.49,84,85 It is hypothesized that using the BAHA device on the deafened ear can expand the sound field for the patient and improve the patient’s speech understanding in noise, similar to a contralateral routing of signal (CROS) hearing aid or transcranial CROS system. A prospective trial of subjects with unilateral deafness using benefit surveys, source identification testing, and hearing in noise testing was undertaken by Niparko and colleagues.84 This study consisted of 10 adults with unilateral deafness (PTA >90 dB, SD <20%) for a variety of etiologies, including acoustic neuroma, meningitis, sudden SNHL, and sudden SNHL with chronic suppurative otitis media. Patients were fitted with contralateral routing of offside signal amplification devices (CROS) for 1 month and tested with contralateral routing of offside signal before mastoid implantation of the deaf ear, fitting, and testing for BAHA.
Another study by Wazen and colleagues85 has also evaluated the BAHA for unilateral SNHL. This prospective study of nine patients included hearing loss from congenital aural atresia or mastoidectomies secondary to chronic ear infections with or without cholesteatoma, or temporal bone tumor excision. Comparing BAHA-aided with the unaided condition, the authors showed that all patients had tonal and spondee threshold improvement with BAHA compared with thresholds before treatment. Speech recognition performance in BAHA-aided conditions was comparable to the patient’s best score in unaided condition, and patients reported a significant improvement in their hearing handicap scores with the BAHA.
A more recent study by Lin and coworkers86 for patients with unilateral SNHL showed consistent satisfaction with BAHA amplification and poor acceptance of CROS amplification. Sound localization was worse when using the CROS aid, but showed no benefit with the BAHA. In keeping with prior studies, the BAHA produced significantly better speech recognition in noise. Similar findings have been borne out by other studies.87 These studies show that the BAHA is an excellent tool for rehabilitation of unilateral SNHL, and seems to outperform the only other rehabilitative option available for this difficult problem, the CROS hearing aid. The primary benefit seems to be improved hearing in noise, whereas the BAHA provides no objective benefit of sound localization.
Implantable Adjuncts to Conventional Hearing Aids
RetroX
The RetroX (Auric Hearing Systems, Rheine, Germany) consists of an inert titanium metal tube that passes from the postauricular sulcus to the posterior aspect of the external auditory canal, passing just lateral to the mastoid cortical bone and external auditory canal bone (Fig. 157-12). Its major purported advantages are that it does not block the external auditory canal, and it does decrease feedback by separating the device microphone (which lies in a BTE unit) and the output of the speaker in the external auditory canal. Secondarily, there is a cosmetic advantage over traditional BTE aids in that the device is largely hidden behind the pinna, with no speaker tube visibly entering the external auditory canal. According to the manufacturer, the device is appropriate for patients with SNHL or CHL to 30 dB HL at 1 kHz and less and 30 to 80 dB HL loss at 3 to 8 kHz, and is especially suitable for cases of high-frequency SNHL with a steep slope (ski-slope audiogram).
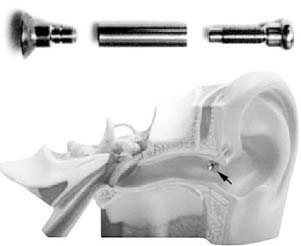
Figure 157-12. Auric metallic tube and transcutaneous placement from retroauricular sulcus to external auditory canal.
To date, few peer-reviewed reports of device results have appeared in the literature. In one study of 10 patients, all were satisfied with the hearing improvement provided by the RetroX.88 There was an improvement of pure-tone thresholds at 1 kHz, 2 kHz, and 4 kHz, whereas in quiet, the speech reception threshold improved by 9 dB. Speech audiometry in noise showed that intelligibility improved by 26% for a signal-to-noise ratio of −5 dB, by 18% for a signal-to-noise ratio of 0 dB, and by 13% for a signal-to-noise ratio of +5 dB. Complications included one patient with acoustic feedback and one patient with a granulomatous reaction to the foreign body that necessitated removing the implant. Refinements to the surgical process of placing the titanium tube seem to have diminished the rate of this complication.89
A subsequent study of 25 patients by this same group was reported after 2.5 to 15 months of use.90 Of 25 patients, 23 were satisfied with the device, whereas 4 patients developed a persistent granulomatous reaction that disappeared after explantation, and 2 patients complained of acoustic feedback and needed supplementary fitting. The authors of this study observed a statistically significant improvement of the pure-tone thresholds, with similar speech in quiet and noise scores as their initial study. A study in 2005 by Barbara and colleagues91 showed that the RetroX provided better hearing in noise with higher rates of satisfaction compared with a CIC hearing aid.91
Barbara M, Bandiera G, Serra B, et al. Digital hearing aids for high-frequency sensorineural hearing loss: preliminary experience with the RetroX device. Acta Otolaryngol. 2005;125:693-696.
Chen DA, Backous DD, Arriaga MA, et al. Phase 1 clinical trial results of the Envoy System: a totally implantable middle ear device for sensorineural hearing loss. Otolaryngol Head Neck Surg. 2004;131:904-916.
Colletti V, Soli SD, Carner M, et al. Treatment of mixed hearing losses via implantation of a vibratory transducer on the round window. Int J Audiol. 2006;45:600-608.
Davids T, Gordon KA, Clutton D, et al. Bone-anchored hearing aids in infants and children younger than 5 years. Arch Otolaryngol Head Neck Surg. 2007;133:51-55.
Goode RL, Rosenbaum ML, Maniglia AJ. The history and development of the implantable hearing aid. Otolaryngol Clin North Am. 1995;28:1-16.
Hol MK, Cremers CW, Coppens-Schellekens W, et al. The BAHA Softband: a new treatment for young children with bilateral congenital aural atresia. Int J Pediatr Otorhinolaryngol. 2005;69:973-980.
House JW, Kutz JWJr. Bone-anchored hearing aids: incidence and management of postoperative complications. Otol Neurotol. 2007;28:213-217.
Jenkins HA, Atkins JS, Horlbeck D, et al. Otologics fully implantable hearing system: Phase I trial 1-year results. Otol Neurotol. 2008;29:534-541.
Jenkins HA, Atkins JS, Horlbeck D, et al. U.S. Phase I preliminary results of use of the Otologics MET Fully-Implantable Ossicular Stimulator. Otolaryngol Head Neck Surg. 2007;137:206-212.
Jenkins HA, Niparko JK, Slattery WH, et al. Otologics Middle Ear Transducer Ossicular Stimulator: performance results with varying degrees of sensorineural hearing loss. Acta Otolaryngol. 2004;124:391-394.
Kiefer J, Arnold W, Staudenmaier R. Round window stimulation with an implantable hearing aid (Soundbridge) combined with autogenous reconstruction of the auricle—a new approach. ORL J Otorhinolaryngol Relat Spec. 2006;68:378-385.
Kompis M, Krebs M, Hausler R. Speech understanding in quiet and in noise with the bone-anchored hearing aids Baha Compact and Baha Divino. Acta Otolaryngol. 2007;127:829-835.
Lin LM, Bowditch S, Anderson MJ, et al. Amplification in the rehabilitation of unilateral deafness: speech in noise and directional hearing effects with bone-anchored hearing and contralateral routing of signal amplification. Otol Neurotol. 2006;27:172-182.
Luetje CM, Brackmann D, Balkany TJ, et al. Phase III clinical trial results with the Vibrant Soundbridge implantable middle ear hearing device: a prospective controlled multicenter study. Otolaryngol Head Neck Surg. 2002;126:97-107.
Lustig LR, Arts HA, Brackmann DE, et al. Hearing rehabilitation using the BAHA bone-anchored hearing aid: results in 40 patients. Otol Neurotol. 2001;22:328-334.
Niparko JK, Cox KM, Lustig LR. Comparison of the bone anchored hearing aid implantable hearing device with contralateral routing of offside signal amplification in the rehabilitation of unilateral deafness. Otol Neurotol. 2003;24:73-78.
Siegert R, Mattheis S, Kasic J. Fully implantable hearing aids in patients with congenital auricular atresia. Laryngoscope. 2007;117:336-340.
Snik AF, Mylanus EA, Proops DW, et al. Consensus statements on the BAHA system: where do we stand at present? Ann Otol Rhinol Laryngol Suppl. 2005;195:2-12.
Todt I, Seidl RO, Gross M, et al. Comparison of different Vibrant Soundbridge audioprocessors with conventional hearing aids. Otol Neurotol. 2002;23:669-673.
Venail F, Lavieille JP, Meller R, et al. New perspectives for middle ear implants: first results in otosclerosis with mixed hearing loss. Laryngoscope. 2007;117:552-555.
Yellon RF. Bone anchored hearing aid in children—prevention of complications. Int J Pediatr Otorhinolaryngol. 2007;71:823-826.
1. 1977 Food and Drug Administration Regulations: Hearing aid devices—professional and patient labeling and conditions for sale. Audiology Update. 1993;12:3-5.
2. Blair B. Audio Engineering Handbook. New York: McGraw-Hill; 1988.
3. Phonak. Available at www.phonak.com, June 2008.
4. Henrichsen J, Noring E, Christensen B, et al. In-the-ear hearing aids: the use and benefit in the elderly hearing-impaired. Scand Audiol. 1988;17:209-212.
5. Huttenbrink KB, Zahnert TH, Bornitz M, et al. Biomechanical aspects in implantable microphones and hearing aids and development of a concept with a hydroacoustical transmission. Acta Otolaryngol. 2001;121:185-189.
6. Boothroyd B. Observations on embryonic chick-bone crystals by high resolution transmission electron microscopy. Clin Orthop. 1975;106:290-310.
7. Eisenberg LS, Shannon RV, Martinez AS, et al. Speech recognition with reduced spectral cues as a function of age. J Acoust Soc Am. 2000;107:2704-2710.
8. Boothroyd A, Springer N, Smith L, et al. Amplitude compression and profound hearing loss. J Speech Hear Res. 1988;31:362-376.
9. Moore BC. Characterization and simulation of impaired hearing: implications for hearing aid design. Ear Hear. 1991;12(6 Suppl):154S-161S.
10. Carle R, Laugesen S, Nielsen C. Observations on the relations among occlusion effect, compliance, and vent size. J Am Acad Audiol. 2002;13:25-37.
11. Goode RL, Rosenbaum ML, Maniglia AJ. The history and development of the implantable hearing aid. Otolaryngol Clin North Am. 1995;28:1-16.
12. Maniglia AJ, Ko WH, Garverick SL, et al. Semi-implantable middle ear electromagnetic hearing device for sensorineural hearing loss. Ear Nose Throat J. 1997;76:333-338.
13. Goode RL. Current status and future of implantable electromagnetic hearing aids. Otolaryngol Clin North Am. 1995;28:141-146.
14. Junker R, Gross M, Todt I, et al. Functional gain of already implanted hearing devices in patients with sensorineural hearing loss of varied origin and extent: Berlin experience. Otol Neurotol. 2002;23:452-456.
15. Ko WH, Zhu WL, Kane M, et al. Engineering principles applied to implantable otologic devices. Otolaryngol Clin North Am. 2001;34:299-314.
16. Kasic JF, Fredrickson JM. The Otologics MET ossicular stimulator. Otolaryngol Clin North Am. 2001;34:501-513.
17. Mueller H, Hall J. Audiologists Desk Reference. San Diego: Singular Publishing Group; 1998.
18. Huttenbrink KB. Middle ear mechanics and their interface with respect to implantable electronic otologic devices. Otolaryngol Clin North Am. 2001;34:315-335.
19. Guinan JJJr, Peake WT. Middle-ear characteristics of anesthetized cats. J Acoust Soc Am. 1967;41:1237-1261.
20. Vlaming MS, Feenstra L. Studies on the mechanics of the normal human middle ear. Clin Otolaryngol. 1986;11:353-363.
21. Vlaming MS, Feenstra L. Studies on the mechanics of the reconstructed human middle ear. Clin Otolaryngol. 1986;11:411-422.
22. Dietz T, Ball G, Katz B. Partially implantable vibrating ossicular prosthesis. Presented at Transducers ‘97: 1997 International Conference on Solid-State Sensors and Actuators, Chicago, 1997.
23. Luetje CM, Brackman D, Balkany TJ, et al. Phase III clinical trial results with the Vibrant Soundbridge implantable middle ear hearing device: a prospective controlled multicenter study. Otolaryngol Head Neck Surg. 2002;126:97-107.
24. Fraysse B, Lavieille JP, Schmerber S, et al. A multicenter study of the Vibrant Soundbridge middle ear implant: early clinical results and experience. Otol Neurotol. 2001;22:952-961.
25. Snik AFM, Mylanus EAM, Cremers CW, et al. Multicenter audiometric results with the vibrant soundbridge, a semi-implantable hearing device for sensorineural hearing impairment. Otolaryngol Clin North Am. 2001;34:373-388.
26. Snik A, Cremers C. Vibrant semi-implantable hearing device with digital sound processing. Arch Otolaryngol Head Neck Surg. 2001;127:1433-1437.
27. Snik A, Cremers C. First audiometric results with the vibrant Soundbridge, a semi-implantable hearing device for sensorineural hearing loss. Audiology. 1999;38:335-338.
28. Huttenbrink K. Current status and critical reflections on implantable hearing aids. Am J Otol. 1999;20:409-415.
29. Dumon T. Vibrant soundbridge middle ear implant in otosclerosis: technique—indication. Adv Otorhinolaryngol. 2007;65:320-322.
30. Wollenberg B, Beltrame M, Schonweiler R, et al. Integration of the active middle ear implant Vibrant Soundbridge in total auricular reconstruction. HNO. 2007;55:349-356.
31. Kiefer J, Arnold W, Staudenmaier R. Round window stimulation with an implantable hearing aid (Soundbridge) combined with autogenous reconstruction of the auricle—a new approach. ORL J Otorhinolaryngol Relat Spec. 2006;68:378-385.
32. Colletti V, Soli SD, Carner M, et al. Treatment of mixed hearing losses via implantation of a vibratory transducer on the round window. Int J Audiol. Oct 2006;45:600-608.
33. Snik FM, Cremers WR. The effect of the “floating mass transducer” in the middle ear on hearing sensitivity. Am J Otol. 2000;21:42-48.
34. Fisch U, Cremers C, Lenarz T, et al. Clinical experience with the Vibrant Soundbridge Implant device. Otol Neurotol. 2001;22:962-972.
35. Thill MP, Gerard JM, Garin P, et al. Belgian experience with the Vibrant Soundbridge prosthesis. Acta Otorhinolaryngol Belg. 2002;56:375-378.
36. Todt I, Seidl RO, Gross M, et al. Comparison of different Vibrant Soundbridge audioprocessors with conventional hearing aids. Otol Neurotol. 2002;23:669-673.
37. Jenkins HA, Niparko JK, Slattery WH, et al. Otologics Middle Ear Transducer Ossicular Stimulator: performance results with varying degrees of sensorineural hearing loss. Acta Otolaryngol. 2004;124:391-394.
38. Traynor R, Fredrickson JM. The future is here: the otologics fully implantable hearing system. Audiology Online. 2007; available at http://www.audiologyonline.com/articles/article_detail.asp?article_id=1903
39. Jenkins HA, Atkins JS, Horlbeck D, et al. Otologics fully implantable hearing system: Phase I trial 1-year results. Otol Neurotol. 2008;29:534-541.
40. Jenkins HA, Atkins JS, Horlbeck D, et al. U.S. Phase I preliminary results of use of the Otologics MET Fully-Implantable Ossicular Stimulator. Otolaryngol Head Neck Surg. 2007;137:206-212.
41. Siegert R, Mattheis S, Kasic J. Fully implantable hearing aids in patients with congenital auricular atresia. Laryngoscope. 2007;117:336-340.
42. Jenkins HA, Pergola N, Kasic J. Intraoperative ossicular loading with the Otologics fully implantable hearing device. Acta Otolaryngol. 2007;127:360-364.
43. Venail F, Lavieille JP, Meller R, et al. New perspectives for middle ear implants: first results in otosclerosis with mixed hearing loss. Laryngoscope. 2007;117:552-555.
44. Kroll K. The effects of physiological pressure extremes on a piezoelectric malleus sensor. Presented at ARO 1998 Midwinter Meeting, St. Petersburg, FL, 1998.
45. St. Croix Medical, Inc. Document # FS0000582EN. Minneapolis, MN: St. Croix Medical, Inc.; 2000.
46. Chen DA, Backous DD, Arriaga MA, et al. Phase 1 clinical trial results of the Envoy System: a totally implantable middle ear device for sensorineural hearing loss. Otolaryngol Head Neck Surg. 2004;131:904-916.
47. Lustig LR, Arts HA, Brackmann DE, et al. Hearing rehabilitation using the BAHA bone-anchored hearing aid: results in 40 patients. Otol Neurotol. 2001;22:328-334.
48. Lustig LR, Niparko JK. Osseo-integrated implants in otology. Adv Otolaryngol Head Neck Surg. 13, 1998.
49. Snik AF, Mylanus EA, Proops DW, et al. Consensus statements on the BAHA system: where do we stand at present? Ann Otol Rhinol Laryngol Suppl. 2005;195:2-12.
50. Hakansson B, Tjellstrom A, Carlsson P. Percutaneous vs. transcutaneous transducers for hearing by direct bone conduction. Otolaryngol Head Neck Surg. 1990;102:339-344.
51. Tjellstrom A, Lindstrom J, Hallen O, et al. Osseointegrated titanium implants in the temporal bone: a clinical study on bone-anchored hearing aids. Am J Otol. 1981;2:304-310.
52. Branemark PI, Adell R, Breine U, et al. Intra-osseous anchorage of dental prostheses, I: experimental studies. Scand J Plast Reconstr Surg. 1969;3:81-100.
53. Eriksson E, Branemark PI. Osseointegration from the perspective of the plastic surgeon. Plast Reconstr Surg. 1994;93:626-637.
54. Albrektsson T, Branemark PI, Jacobsson M, et al. Present clinical applications of osseointegrated percutaneous implants. Plast Reconstr Surg. 1987;79:721-731.
55. Johansson CB, Sennerby L, Albrektsson T. A removal torque and histomorphometric study of bone tissue reactions to commercially pure titanium and Vitallium implants. Int J Oral Maxillofac Implants. 1991;6:437-441.
56. Pazzaglia UE, Bernini F, Zatti G, et al. Histology of the metal-bone interface: interpretation of plastic embedded slides. Biomaterials. 1994;15:273-277.
57. Albrektsson T, Branemark PI, Hansson HA, et al. Osseointegrated titanium implants. Requirements for ensuring a long-lasting, direct bone-to-implant anchorage in man. Acta Orthop Scand. 1981;52:155-170.
58. Branemark PI, Albrektsson T. Titanium implants permanently penetrating human skin. Scand J Plast Reconstr Surg. 1982;16:17-21.
59. Laing PG, Ferguson ABJr, Hodge ES. Tissue reaction in rabbit muscle exposed to metallic implants. J Biomed Mater Res. 1967;1:135-149.
60. Mylanus EA, Cremers CW, Snik AF, et al. Clinical results of percutaneous implants in the temporal bone. Arch Otolaryngol Head Neck Surg. 1994;120:81-85.
61. Niparko JK, Langman AW, Cutler DS, et al. Tissue-integrated prostheses in the rehabilitation of auricular defects: results with percutaneous mastoid implants. Am J Otol. 1993;14:343-348.
62. Granstrom G, Bergstrom K, Tjellstrom A. The bone-anchored hearing aid and bone-anchored epithesis for congenital ear malformations. Otolaryngol Head Neck Surg. 1993;109:46-53.
63. Tjellstrom A, Hakansson B. The bone-anchored hearing aid: design principles, indications, and long-term clinical results. Otolaryngol Clin North Am. 1995;28:53-72.
64. Catalano PJ, Choi E, Cohen N. Office versus operating room insertion of the bone-anchored hearing aid: a comparative analysis. Otol Neurotol. 2005;26:1182-1185.
65. Branemark PI, Hansson BO, Adell R, et al. Osseointegrated implants in the treatment of the edentulous jaw: experience from a 10-year period. Scand J Plast Reconstr Surg Suppl. 1977;16:1-132.
66. Eriksson RA, Albrektsson T, Magnusson B. Assessment of bone viability after heat trauma: a histological, histochemical and vital microscopic study in the rabbit. Scand J Plast Reconstr Surg. 1984;18:261-268.
67. Adell R, Lekholm U, Rockler B, et al. Marginal tissue reactions at osseointegrated titanium fixtures, I: a 3-year longitudinal prospective study. Int J Oral Maxillofac Surg. 1986;15:39-52.
68. Lekholm U, Adell R, Lindhe J, et al. Marginal tissue reactions at osseointegrated titanium fixtures, II: a cross-sectional retrospective study. Int J Oral Maxillofac Surg. 1986;15:53-61.
69. Snyder MC, Moore GF, Johnson PJ. The use of full-thickness skin grafts for the skin-abutment interface around bone-anchored hearing aids. Otol Neurotol. 2003;24:255-258.
70. Tjellstrom A, Granstrom G. How we do it: frequency of skin necrosis after BAHA surgery. Clin Otolaryngol. 2006;31:216-220.
71. Hol MK, Cremers CW, Coppens-Schellekens W, et al. The BAHA Softband: a new treatment for young children with bilateral congenital aural atresia. Int J Pediatr Otorhinolaryngol. 2005;69:973-980.
72. Yellon RF. Bone anchored hearing aid in children—prevention of complications. Int J Pediatr Otorhinolaryngol. 2007;71:823-826.
73. Davids T, Gordon KA, Clutton D, et al. Bone-anchored hearing aids in infants and children younger than 5 years. Arch Otolaryngol Head Neck Surg. 2007;133:51-55.
74. Tjellstrom A, Hakansson B, Granstrom G. Bone-anchored hearing aids: current status in adults and children. Otolaryngol Clin North Am. 2001;34:337-364.
75. Holgers KM, Tjellstrom A, Bjursten LM, et al. Soft tissue reactions around percutaneous implants: a clinical study of soft tissue conditions around skin-penetrating titanium implants for bone-anchored hearing aids. Am J Otol. 1988;9:56-59.
76. Jacobsson M, Tjellstrom A, Thomsen P, et al. Soft tissue infection around a skin penetrating osseointegrated implant: a case report. Scand J Plast Reconstr Surg Hand Surg. 1987;21:225-228.
77. House JW, Kutz JWJr. Bone-anchored hearing aids: incidence and management of postoperative complications. Otol Neurotol. 2007;28:213-217.
78. Scholz M, Eufinger H, Anders A, et al. Intracerebral abscess after abutment change of a bone anchored hearing aid (BAHA). Otol Neurotol. 2003;24:896-899.
79. Hakansson B, Liden G, Tjellstrom A, et al. Ten years of experience with the Swedish bone-anchored hearing system. Ann Otol Rhinol Laryngol Suppl. 1990;151:1-16.
80. Stevenson DS, Proops DW, Wake MJ, et al. Osseointegrated implants in the management of childhood ear abnormalities: the initial Birmingham experience. J Laryngol Otol. 1993;107:502-509.
81. Liepert DR, DiToppa JC. The Nobelpharma auditory system bone-anchored hearing aid: the Edmonton experience. J Otolaryngol. 1994;23:411-418.
82. Kompis M, Krebs M, Hausler R. Speech understanding in quiet and in noise with the bone-anchored hearing aids Baha Compact and Baha Divino. Acta Otolaryngol. 2007;127:829-835.
83. Bosman AJ, Snik AF, Mylanus EA, et al. Fitting range of the BAHA Cordelle. Int J Audiol. 2006;45:429-437.
84. Niparko JK, Cox KM, Lustig LR. Comparison of the bone anchored hearing aid implantable hearing device with contralateral routing of offside signal amplification in the rehabilitation of unilateral deafness. Otol Neurotol. 2003;24:73-78.
85. Wazen JJ, Spitzer J, Ghossaini SN, et al. Results of the bone-anchored hearing aid in unilateral hearing loss. Laryngoscope. 2001;111:955-958.
86. Lin LM, Bowditch S, Anderson MJ, et al. Amplification in the rehabilitation of unilateral deafness: speech in noise and directional hearing effects with bone-anchored hearing and contralateral routing of signal amplification. Otol Neurotol. 2006;27:172-182.
87. Wazen JJ, Ghossaini SN, Spitzer JB, et al. Localization by unilateral BAHA users. Otolaryngol Head Neck Surg. 2005;132:928-932.
88. Garin P, Genard F, Galle C, et al. The RetroX auditory implant for high-frequency hearing loss. Otol Neurotol. 2004;25:511-519.
89. Wesendahl T. Insertion procedure, aftercare, and soft-tissue reaction of the 2.5-mm titanium tube system for a transcutaneous air conduction hearing aid system. Int Tinnitus J. 2006;12:3-8.
90. Garin P, Genard F, Galle C, et al. Rehabilitation of high-frequency hearing loss with the RetroX auditory implant. B-ENT. 2005;1:17-23.
91. Barbara M, Bandiera G, Serra B, et al. Digital hearing aids for high-frequency sensorineural hearing loss: preliminary experience with the RetroX device. Acta Otolaryngol. 2005;125:693-696.