10 IMMUNE-LYMPHATIC SYSTEM
Organization of the immune-lymphatic system
The lymphatic system includes primary and secondary lymphoid organs.
The primary lymphoid organs produce the cell components of the immune system. They are (1) the bone marrow and (2) the thymus. The main cell type is the lymphocyte originated from a lymphoid stem cell in bone marrow (Figure 10-1).
The main function of the lymphoid organs, as components of the immune system, is to protect the body against invading pathogens or antigens (bacteria, viruses, and parasites). The basis for this defense mechanism, or immune response, is the ability to distinguish self from nonself.
The two key cell components of the immune system are lymphocytes and accessory cells (Figure 10-2). Lymphocytes include two major cell groups: (1) B cells, responding to cell-free and cell-bound antigens; and (2) T cells, subdivided into two categories: helper T cells and cytolytic or cytotoxic T cells. T cells respond to cell-bound antigens presented by specific molecules.
INNATE (NATURAL) AND ADAPTIVE (ACQUIRED) IMMUNITY
Immunity in general is the reaction of cells and tissues to foreign (nonself) substances or pathogens including bacterial, viral, and parasite antigens. Two types of immunity are distinguished: (1) innate or natural immunity and (2) adaptive or acquired immunity (Figure 10-3).
Innate or natural immunity of the newborn is the simplest mechanism of protection. It does not require previous exposure to a pathogen and elicits rapid responses by macrophages and dendritic cells. Toll-like receptors (see Box 10-A) initiate innate immunity against components of invading pathogens (such as nucleic acids, proteins, lipids and polysaccharides). Stimulation of macrophages and dendritic cells by their ligand-bound Toll-like receptors leads to the production and secretion of proinflammatory cytokines, thereby initiating an inflammatory response.
Box 10-A Toll-like receptors
Adaptive or acquired immunity develops when an individual is exposed to a pathogen with the aims of eliminating the pathogen as well as the generation of immunologic memory. To achieve adaptive or acquired immunity, it is necessary to select lymphocytes (clonal selection) from a vast repertoire of cells bearing antigen-specific receptors generated by a mechanism known as gene rearrangement. Essentially, adaptive immunity is the perfection of innate or natural immunity that recognizes vital components of the microorganism utilizing a limited number of pattern-recognition receptors expressed on all cells of a given type (nonclonal) and independent of immunologic memory.
Adaptive immunity involves two types of responses to an antigen (pathogen): The first response is mediated by antibodies produced by plasma cells, the final differentiation product of B cells as we have seen in Chapter 4, Connective Tissue. This response is known as humoral immunity and operates against antigens located outside a cell or bound to its surface. When antibodies bind to an antigen or toxins produced by a pathogen, they can facilitate the phagocytic action of macrophages or recruit leukocytes and mast cells to take advantage of their cytokines and mediators, respectively, and strengthen a response. Humoral immunity results in persistent antibody production and production of memory cells.
Properties of adaptive or acquired immunity
B CELLS
The bone marrow is the site of origin of B and T cells from a lymphoid stem cell. In Chapter 6, Blood and Hematopoiesis, we discussed developmental aspects of the myeloid and erythroid lineages from a hematopoietic stem cell. The same hematopoietic stem cell gives rise to a lymphoid stem cell that generates precursors for B cells and T cells (see Figure 10-1). B cells mature in the bone marrow, whereas the thymus is the site of maturation of T cells.
Stem B cells in the bone marrow proliferate and mature in a microenviron-mental niche provided by bone marrow stromal cells producing interleukin-7 (IL-7) (Figure 10-4). During maturation, B cells express on their surface immunoglobulins M (IgM) or D (IgD) interacting with two additional proteins linked to each other, immunoglobulins α (Igα) and β (Igβ). The cell surface IgM or IgD, together with the conjoined Igα and Igβ, form the B cell antigen receptor complex. The intracellular domains of Igα and Igβ contain a tyrosine-rich domain called immunoreceptor tyrosine-based activation motif (ITAM).
Self-antigens present in the bone marrow test the antigen-binding specificity of IgM or IgD on B cell surfaces. This is a required testing step before B cells can continue their maturation, enter peripheral lymphoid tissues, and interact with foreign (non-self) antigens. Self-antigens binding strongly to two or more IgM or IgD receptor molecules on B cells induce apoptosis. Self-antigens with a weaker binding affinity for the B cell antigen receptor complex enable the survival and maturation of these B cells when ITAMs of IgM- or IgD-associated Igα and Igβ transduce signaling events, resulting in further differentiation of B cells and the entrance of mature B cells into the circulation.
T CELLS
Major histocompatibility complex and human leukocyte antigens
The presentation of antigens to T cells is carried out by specialized proteins encoded by genes in the major histocompatibility locus and present on the surface of antigen-presenting cells. Antigen-presenting cells survey the body, find and internalize antigens by phagocytosis, break them down into antigenic peptide fragments, and bind them to major histocompatibility complex (MHC) molecules (Figure 10-5) so that the antigen peptide fragment–MHC complex can be exposed later on the surface of the cells. The MHC gene locus expresses gene products responsible for the rejection of grafted tissue between two genetically incompatible hosts.
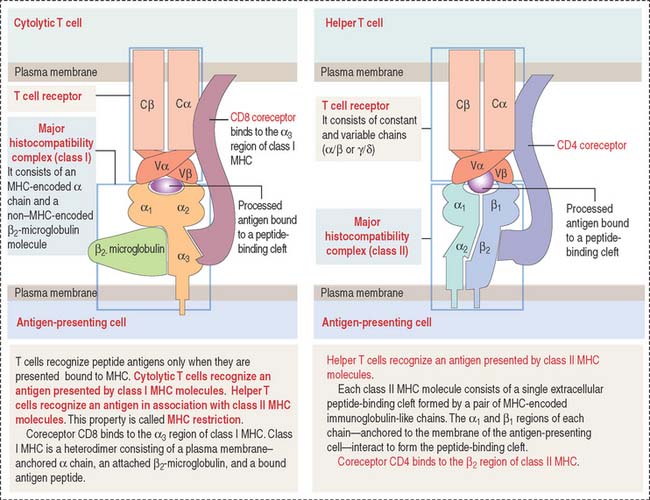
Figure 10-5 Structure of the T cell receptor and class I and II major histocompatibility complex (MHC)
Class II MHC consists of two polypeptide chains, an α chain and a β chain. Both chains are encoded by the MHC gene locus. The α1 and β1 domains form an antigen-binding cleft. CD4, a coreceptor on the surface of helper T cells, binds to the β domain of class II MHC. CD4 and CD8 are cell surface identifiers, members of the cluster of differentiation or designation (abbreviated as CD). See Box 10-B.
Box 10-B CD antigens
T cell receptor complex
In addition to MHC molecules, subsets of T cells have cell surface receptors that enable each of them to recognize a different antigen peptide–MHC combination. Antigen recognition involves an immunologic synapse mechanism consisting in the formation of stable antigen-presenting cell–T cell adhesiveness followed by an activating signaling cascade by T cells (see Box 10-C).
Box 10-C The Immunologic synapse
The TCR consists of two disulfide-linked transmembrane polypeptide chains: the α chain and the β chain (see Figure 10-5). A limited number of T cells have a TCR composed of γ and δ chains. Each α and β chain consists of a variable (Vα and Vβ) domain and a constant (Cα and Cβ) domain. When compared with the immunoglobulin molecule, the Vα and Vβ domains are structurally and functionally similar to the antigen-binding fragment (Fab) of immunoglobulins.
The TCR molecule is associated with two proteins, CD3 and ζ (not shown in Figure 10-5), forming the TCR complex. CD3 and ζ have a signaling role and are present in all T cells. CD3 contains the ITAM cytoplasmic domain previously mentioned as part of the B cell antigen receptor complex and involved in signaling functions.
CD4 and CD8 coreceptors
CD4 and CD8 are T cell surface proteins interacting selectively with class II MHC and class I MHC molecules, respectively. When the TCR recognizes an antigen bound to the cleft of MHC, CD4 or CD8 coreceptors cooperate in the activation of T cell function (see Figure 10-5).
CD4 and CD8 are members of the immunoglobulin (Ig) superfamily. In Chapter 1, Epithelium, we discussed the function and structure of cell adhesion molecules belonging to the Ig superfamily.
Members of the Ig superfamily have a variable number of extracellular Ig-like domains. The two terminal Ig-like domains of CD4 bind to the β2 domain of the class II MHC (see Figure 10-5). The single Ig-like domain of CD8 binds to the α3, domain of the class I MHC.
Thus, CD4+ helper T cells recognize antigens associated with class II MHC, and CD8+ cytolytic T cells (cytolytic thymus-derived lymphocytes [CTL]) respond to antigens presented by class I MHC (Figure 10-6).
MHC molecules and adaptive immune responses
During their maturation in the cortex of the thymus, T cells are selected to be self-MHC–restricted and self-tolerant. This selective process, known as positive selection, occurs only when self-MCH–restricted T cells are selected (see Figure 10-7). Negative selection takes place when T cells do not bind to any MHC or bind to the body’s tissue-specific antigens (self-molecules). We discuss later how a portfolio of self-antigens expressed in the thymus permits the elimination of autoreactive T cells by apoptosis. Only those T cells that can recognize foreign peptides and self-MHC survive, leave the thymus, and migrate into the secondary lymphoid organs.
The cortex of the thymus contains branching and interconnected thymic cortical epithelial cells involved in the positive selection of T cells. The medulla of the thymus houses thymic medullary epithelial cells involved in the negative selection of potentially autoreactive T cells. Contact between MHC molecules on the thymic epithelial cell surfaces and TCRs of developing T cells is an important feature in positive selection. This is another example of the importance of the immunological synapse (see Box 10-C).
T cells developing in the thymus express specific cell surface molecules
TCR consists of two pairs of subunits: αβ chains or γδ chains (see Figure 10-3). Each chain can vary in sequence from one T cell to another. This variation is determined by the random combination of gene segments and has a bearing on which foreign antigen T cells can recognize.
T cell-mediated immunity
Cytolytic (or killer) T cells display both the TCR and CD8 coreceptor. Cytolytic T cells recognize class I MHC on antigen-presenting cells. We will return to the clinical significance of helper and cytolytic T cells when we discuss their involvement in the pathology of human immunodeficiency virus-type 1 (HIV-1) infection, allergy, and cancer immunotherapy.
How do helper T cells help?
Helper T cells are activated when they recognize the antigen peptide–class II MHC complex (Figure 10-8).
Plasma cells synthesize only one class of immunoglobulin (several thousand immunoglobulin molecules per second; lifetime of a plasma cell is from 10 to 20 days). Five classes of immunoglobulins are recognized in humans: IgG, IgA, IgM, IgE, and IgD (see Box 10-D). Abnormal plasma cells may accumulate in bones and bone marrow, causing bone destruction and affecting the production of normal blood cells. This pathologic condition is called multiple myeloma (see Box 10-E).
Box 10-D Immunoglobulins