CHAPTER 37 Imaging of Coronary Revascularization
Coronary Stents and Bypass Grafts
NONINVASIVE EVALUATION OF CORONARY STENTS
Since Dr. Andreas Gruentzig performed the first successful percutaneous transluminal coronary angioplasty (PTCA) in 1977,1 this procedure has become commonplace with more than 550,000 PTCA procedures performed in the United States in 2000. In the late 1980s Dr. Julio Palmaz invented the bare-metal stent (BMS). In 1993, randomized clinical trials demonstrated decreased angiographic restenosis rates (defined as >50% narrowing of a previously stented site) compared with PTCA alone—ushering in the era of elective stent implantation.2 Currently, more than 80% of patients undergoing percutaneous coronary intervention also receive intracoronary stents (Fig. 37-1).3 Intracoronary stent implantation is not, however, without risk with two major complications—occlusion secondary to thrombosis and restenosis.4 Many patients undergo repeat angiography to ascertain the presence of these complications; however, given the invasive nature of coronary angiography and its potential complications, a noninvasive technique for detection of these complications would be clinically important.
In-Stent Restenosis and Thrombosis—Etiology, Prevalence, Pathophysiology
Restenosis after angioplasty can result from early vessel recoil, late constrictive remodeling (also called negative remodeling), and neointimal proliferation. Elastic recoil occurs nearly instantaneously, secondary to passive recoil of elastic media. Late constrictive remodeling occurs within 1 to 6 months and is secondary to increased collagen in the extracellular matrix and adventitial thickening.1 Intracoronary stents prevent these mechanisms (acute recoil and constrictive remodeling) (Fig. 37-2).
Unfortunately, stent-induced vascular injury causes increased neointimal hyperplasia/proliferation. The pathophysiology includes proliferation and migration of smooth muscle cells and extracellular matrix formation.1 In-stent restenosis, the principal problem after angioplasty, has a clinical incidence of 20% to 40% for bare-metal stents.5,6 Although several characteristics of high-risk populations have been described as clinical predictors, the likelihood of restenosis in a particular patient remains largely unpredictable.6,7
Drug-eluting stents (DES) have revolutionized the treatment of coronary artery disease through marked reduction of in-stent restenosis.8 The components of these stents can be divided into a platform (the stent), a carrier (usually a polymer), and an agent (a drug) to prevent restenosis (Fig. 37-3).2 The advantage of using a stent as a delivery system is that it allows for local delivery of the drug and averts the need for higher systemic doses. The biology of vascular cells and the cell cycle is being used as a target to prevent restenosis and current agents used in drug-eluting stents all interfere with the cell cycle in some manner.1 Numerous trials have established that sirolimus and paclitaxel drug-eluting stents markedly reduce the incidence of in-stent restenosis. DES have reduced restenosis rates to less than 10% at a 12-month follow-up.6,9,10
Although drug-eluting stents have significantly reduced restenosis events, its very effectiveness has led to increased rates of an uncommon but potentially very severe complication, in-stent thrombosis. To understand this phenomenon, it is important to be aware of risk factors for development of in-stent thrombosis. Persistent, slow coronary blood flow such as occurs with dissection or hypoperfusion, exposure of the blood to prothrombotic subendothelial constituents, such as tissue factor or to the stent itself, and failure to suppress platelet adhesion/aggregation at a time of prothrombotic risk predispose the patient to in-stent thrombosis.11
A probable explanation for an increased risk of thrombosis in DES compared to BMS after cessation of antiplatelet therapy is delayed arterial healing.11 Normally after vascular injury, the vessel wall undergoes a number of changes including migration and hypertrophy of vascular smooth muscle cells. During this period, endothelial cells colonize the surface of the stent and regain their normal function.12 DES cause incomplete neointimal coverage and delay endothelialization of the stent. Subsequently, there is eventual propagation of thrombi over the surface of the stent.
The reported incidence of thrombosis of bare-metal stents 1 month postprocedure has ranged from 0.5% to 2.5% in clinical trials. Bare-metal stent thrombosis usually occurs within the first 24 to 48 hours or much less commonly within the first month after stent placement. Eighty percent of stent thrombosis events have been found to occur within the first 2 days and events occurring more than 1 week after the procedure were rare.13 Stent thrombosis within 1 year of implantation occurs with similar frequency between DES and BES; however beyond 1 year, there is a small but significant increased risk of very late stent thrombosis in DES.14 Because of this increased risk, extension of antiplatelet therapy is recommended.
The decision to place a drug-eluting rather than a bare-metal stent therefore rests on clinical grounds and evaluation of the benefits and risks of these stents. The lower rate of repeat target revascularization with DES must be weighed against the cost of longer term antiplatelet therapy to prevent stent thrombosis, the risk of bleeding, and the complications of noncompliance with drug therapy (Fig. 37-4).
Clinical Presentation
Restenosis following angioplasty and stent implantation is classically considered a benign process in which the typical patient presents with exertional angina.14 Importantly, however, an appreciable proportion of these patients present with an acute coronary syndrome. One study from the Cleveland Clinic demonstrated that 9.5% of patients with restenosis presented with acute myocardial infarction and 26.4% presented with unstable angina.15
Stent thrombosis is particularly concerning because of its potential catastrophic consequences. As stents are often placed in proximal segments of major coronary arteries, thrombotic occlusion usually manifests as severe ischemia or myocardial infarction. One study demonstrated that 70% of cases of stent thrombosis manifested as acute myocardial infarction.8,16 Mortality rates are very high ranging from 11% to 15% for BMS thromboses and 25% to 45% with DES thrombosis. The higher mortality with DES thrombosis has been suggested to be secondary to a combination of abrupt thrombotic events and decreased collateral formation.14 Again, the risk of coronary artery stent thrombosis and its frequent consequences of myocardial infarction or death are minimized by the use of dual antiplatelet therapy. Patient compliance is also an important issue when determining the type of stent to use.
Imaging Techniques and Findings
Radiography
To date, the majority of patients with chest pain after coronary artery stent placement undergo catheter angiography. This invasive method, however, has the disadvantage of moderate-to-high cost and the possibility of severe complications. Therefore, a noninvasive alternative for the assessment of stented segments in these patients would be highly desirable.17 To that end, attempts have been made to noninvasively assess coronary artery stents with varying degrees of success.
Ultrasound
Transcatheter intravascular ultrasound (IVUS) imaging is another invasive technique in which a miniaturized ultrasound transducer, mounted on the tip of catheter, is inserted directly into a vessel to produce in-vivo real time assessment of the vascular lumen as well as plaque composition. IVUS has been used during stent placement with the promise that it will improve the clinical outcome of stent placement via reduction of incomplete expansion and incomplete apposition of the stent to the vessel wall. Also, IVUS allows for the measurement of minimal stent area which has been found to predict angiographic and clinical restenosis.18
MRI
The coronary arteries can be evaluated using cardiac MRI techniques; however, coronary magnetic resonance angiography (MRA) is currently not as easily performed or as fast as coronary computed tomographic angiography (CTA). A number of unsolved problems limit MRI reliability. Long acquisition times, the small size of the coronary arteries, and cardiac and respiratory artifacts causing nonevaluable segments hamper the clinical implementation of coronary MRA.19
Furthermore, although multiple studies have demonstrated that MRI performed less than 8 weeks after coronary stent placement is safe,20–22 local susceptibility artifact leads to signal voids/artifacts at the site of the stent on MR images. These artifacts can be substantial and preclude direct MR evaluation of in-stent and persistent coronary patency.23
CT
Noninvasive assessment using computed tomography to assess in-stent stenosis has been attempted since the era of electron beam CT (EBCT). Early studies with EBCT could not directly visualize in-stent restenosis and quantification was not possible.24,25
Multidetector CT (MDCT) has several advantages over EBCT; of note, MBCT has increased spatial resolution and improved signal-to-noise ratio.17 Early MDCT scanning with four-detector scanners were inadequate for stent interpretation with inability to visualize the majority of the stent lumen.17,26 Subsequent studies using 16-, 40-, and 64-detector scanners have had mixed results. Most studies demonstrated high sensitivity and specificity but in general ignored nonevaluable segments from analysis. The number of stents found nonevaluable (up to 54% of 232 stents in a study by Gilard and colleagues), is a major limitation of this analysis. The negative predictive value was generally greater than 95%; however, the positive predictive value ranged from 29% to 78%.6,27–34
Hamon and colleagues performed a meta-analysis of 15 studies analyzing the diagnostic capabilities of 16-, 40-, and 64-detector CT scanners in comparison with invasive coronary angiography for detection of in-stent stenosis. 13% of 1175 stents in these studies were nonevaluable. After exclusion of these stents from analysis, they found a sensitivity of 84% and a specificity of 91%.35 The positive-predictive value (PPV) was almost uniformly low secondary to nonevaluable stents being classified as in-stent restenosis (ISR) for statistical analysis. An additional meta-analysis of 16- to 64-detector row CT scanners performed by Sun and colleagues demonstrated similar results. Although there was the hope that 64-detector row CT scanners would be more accurate than slower 16-detector row CT scanners, both meta-analyses demonstrated equivalent sensitivity and specificity.35,36 Kumbani and coworkers recently performed a meta-analysis of 14 studies solely using 64-detector row CT scanners for detection of ISR. Overall sensitivity was 91%, specificity was 91%, PPV was 68%, and the negative-predictive value (NPV) was 98%. Nine percent of 1447 stents were deemed nonevaluable with decreased performance with inclusion of these stents. Only five studies in the analysis included stents less than 3 mm.37 Given that a large number of stents in the general population are less than 3 mm, this further decreases the utility of MDCT in nonselected stent patients.
A post-hoc analysis of 75 stents in 52 patients from the Core-64 trial, the first multicenter international, single-blinded study to determinate the accuracy of 64-detector row CT MDCT, was performed to assess the accuracy of MDCT in detecting in-stent restensosis. This analysis demonstrated less favorable results than the previous meta-analyses. Only 48 of 75 stents (64%) were considered evaluable and there was an overall accuracy of 77.1% for detection of 50% in-stent restenosis of evaluable stents. The PPV and NPV was 57.1% and 80.5%, respectively. A quantitative approach using in-stent and peri-stent attenuation didn’t improve accuracy. The researchers attribute the poor performance of MDCT to the fact that 80% of stents were <3 mm with secondary problems of calcifications, motion artifact, and blooming artifact.38
In general, there is increased evaluability and improved detection of in-stent restenosis with larger stents and unfavorable results with stents less than 3 mm diameter. High heart rates, calcification, and increased body mass index decreased the accuracy of MDCT.32 When considered in the evaluation, thicker stent struts also reduced the evaluability of the stents.36
Dual-source CT scanners have improved temporal resolution compared with single source scanners and promise to potentially minimize motion artifacts that limit in-stent evaluation. Pugliese and associates evaluated a dual-source CT scanner for evaluation of instant restenosis in 100 patients with 178 stents. Nine of the stents were uninterpretable (all of which were smaller than 2.75 mm in diameter) secondary to high-density artifacts obscuring the stent lumen. Sensitivity, specificity, PPV, and NPV in detecting >50% restenosis was 94%, 92%, 77%, and 98%, respectively. The diagnostic performance of the dual source CT scanner at heart rates less than 70 bpm did not differ significantly from its performance with faster heart rates. The stent diameter was important as the sensitivity was 100% in >3 mm diameter stents but dropped to 84% in <2.75 mm stents. Similarly the specificity and the PPV dropped precipitously as the stent diameter decreased. This study demonstrated that the dual-source CT scanner was able to negate the effect of heart rate on evaluation of stent stenosis. The NPV was very good (97% to 98%), even in patients with fast heart rates. Stent diameter was the most important feature in this study.39
In the evaluation of the coronary arteries in a patient with history of percutaneous coronary intervention and stent placement, a clinical history with specific locations of stent placement is of utmost importance. Given that concentric calcification can mimic a stent and not all stents are uniformly recognized on MDCT;40 a history of stent placement allows for a more accurate interpretation—understanding that even with the most current scanners, evaluation of in-stent restenosis remains challenging.
CT scanners with 64 or more detectors are, in general, currently considered the standard for coronary CTA because the reduced breath-holding time afforded by these scanners is better tolerated by patients owing to minimization of motion artifacts.7,41 Metallic stents cause beam hardening on CT imaging resulting in stent struts appearing thicker (or “bloomed”) than they really are with secondary overlap of the vessel lumen and underestimation of the in-stent luminal diameter. In addition, this artifact can cause streaky dark bands which can simulate stenosis (Fig. 37-5).42 Calcification of the vessel wall near or at the outer surface of an implanted stent also contributes to beam hardening. Partial volume averaging is another artifact inherent in cross-sectional imaging and particularly problematic given the small caliber of the coronary arteries.7,43
Sharp filters and thin slices (0.5 to 0.6 mm) reduce blooming and partial volume averaging for improved assessment of stent patency.44 Studies have demonstrated that dedicated edge-enhancing convolution kernels decrease severity of blooming artifacts, resulting in superior depiction of the stent lumen.7 The disadvantages of these filters are an increase in image noise. The most appropriate filter must be chosen to account for this trade-off.
Analysis
When these technical factors are optimized and the data are obtained, the stent lumen should be evaluated using multiplanar reformation (MPR) and cross-sectional/short axis images. Appropriate display window settings must be considered to most accurately evaluate these images. Wide window settings (e.g., width 1500 HU) and center level of 300 HU have been recommended to further decrease image interpretation difficulties with blooming artifact.7
When deemed evaluable, the in-stent lumen should be check for in-stent restenosis. The level and quality of enhancement should be ascertained and any in-stent filling defects should be noted. Homogeneous high attenuation in the stent similar to the attenuation in the proximal or distal reference vessel implies normal flow (Figs. 37-6 and 37-7). Different grades of restenosis similar to those used by Gasper and colleagues and Ehara and associates27,34 can be used (Figs. 37-8 and 37-9), including mild neointimal proliferation (<50% stenosis), significant (>50% restenosis), severe (>75% stenosis)/possible occlusion, and definitive occlusion (100% occlusion).
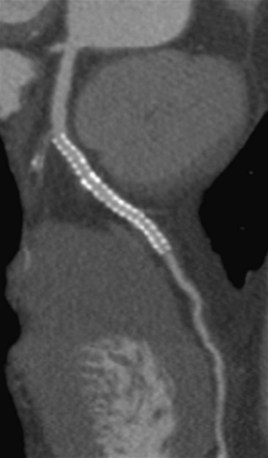
FIGURE 37-6 Homogeneous contrast attenuation within LAD stent demonstrates lack of significant in-stent restenosis.
(Courtesy of Dr. Sablayrolles, Saint-Denis France.)
The perigraft vasculature should also be analyzed as restenosis at stent borders and is reported to occur frequently.45 No contrast distal to the stent indicates definitive occlusion.46 The presence of contrast distal to the stent does not, however, exclude occlusion secondary to the possibility of collateral vasculature feeding the vessel segments distal to the occluded stent in a retrograde direction (Fig. 37-10). It has also been noted that with stent restenosis, the CT attenuation of vessels distal to the stent is decreased compared with the attenuation of the artery proximal to the stent; however, no specific cutoff point has been ascertained.31