CHAPTER 120 Ileus and Pseudo-obstruction
The functions of the small intestine and colon are regulated through the integrated activities of intestinal smooth muscle, the interstitial cells of Cajal (ICC), intrinsic and extrinsic nerves, neurohumoral peptides, and gastrointestinal hormones (see Chapters 97 and 98). Neurons located in the intestine wall constitute the intrinsic neural network known as the enteric nervous system (ENS) (Fig. 120-1). The extrinsic network consists of visceral sensory afferents in the vagus, thoracic, and pelvic splanchnic nerves (Fig. 120-2) and visceral motor efferents of the autonomic nervous system. These extrinsic neurons synapse with the ENS and connect it to the central nervous system.
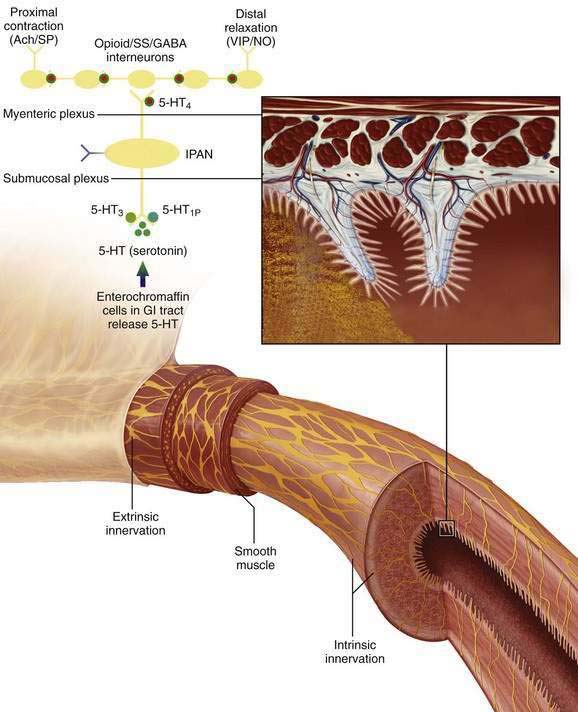
Figure 120-1. Intrinsic pathways that govern intestinal motility. The enteric nervous system (ENS) includes intrinsic motor neurons (efferent), extrinsic motor neurons (efferent), interneurons, and sensory neurons, which together make the ENS capable of carrying reflexes and acting as a center of integration in the absence of input from the central nervous system. Ach, acetylcholine; CGRP, calcitonin gene-related peptide; GABA, γ-aminobutyric acid; GI, gastrointestinal; 5-HT, serotonin; 5-HT1P, serotonin 1p isotype; 5-HT3, serotonin 3 isotype; 5-HT4, serotonin 4 isotype; IPAN, intrinsic primary afferent neuron; NO, nitric oxide; PACAP, pituitary adenylate cyclase-activating peptide; SP, substance P; SS, somatostatin; VIP, vasoactive intestinal peptide. (See text and Chapters 97 and 98.)
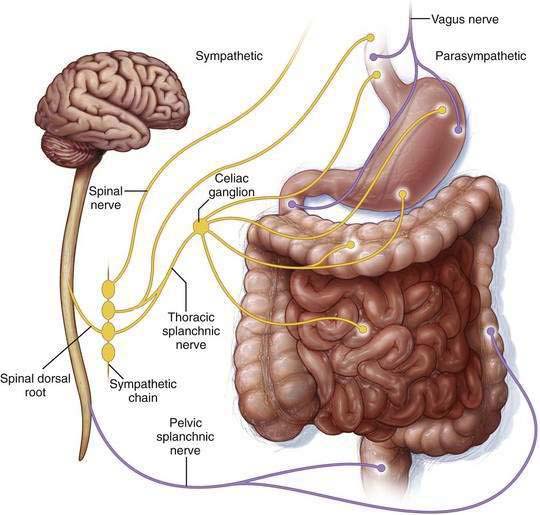
Figure 120-2. Extrinsic pathways governing intestinal motility. Enteric control is modulated by the parasympathetic and sympathetic nerves of the autonomic nervous system, which respectively stimulate and inhibit nonsphincteric muscle. (See text and Chapters 97 and 98.)
EPIDEMIOLOGY
The prevalence of intestinal dysmotility varies according to the underlying pathology and pathophysiology. Postoperative ileus is an unavoidable adverse response to abdominal or retroperitoneal surgery that accounts for delayed refeeding, prolonged hospitalization, and increased costs. It is the main determinant of length of hospital stay after uncomplicated abdominal surgery.1 The economic impact of postoperative ileus in the United States is estimated to be more than $7.5 billion annually, not including lost work expense. Acute colonic pseudo-obstruction is estimated to occur in 0.1% of surgical patients, 0.05% of patients admitted for trauma,2,3 and 0.3% of critically ill patients with burn injury.4 More than 95% of patients with acute colonic pseudo-obstruction have factors that predispose them to develop the condition,3 including nonsurgical trauma, pelvic or hip surgery, cardiovascular disease, or infection.5 Spontaneous perforation rates in this condition range from 3% to 15%, with an associated 50% mortality rate.
Most cases of chronic pseudo-obstruction result from primary acquired defects in nerves, ICC, and smooth muscle cells, or they are secondary to metabolic disorders, inflammation, infiltrating disease, autoimmune conditions, or cancer, namely paraneoplastic chronic pseudo-obstruction.6,7 Of the developmental neuropathies, the prototypical congenital dysmotility disorder, Hirschsprung’s disease, occurs in one in 5000 live births (see Chapter 96). The literature documents several heritable degenerative smooth muscle disorders—familial visceral myopathies (FVMs) and childhood visceral myopathies (CVMs)—and inherited degenerative disorders of the myenteric plexus (familial visceral neuropathies [FVNs]) that are rarely encountered, even in tertiary centers. A small number of families, mostly whites and less often African Americans8 and Latin Americans,9 has been documented with these conditions.
NEURAL CONTROL OF SMALL BOWEL AND COLONIC MOTILITY
The enteric nervous system (ENS) consists of extensive ganglionated plexuses located in the muscular wall of the gastrointestinal tract10; functionally most important are the myenteric and submucosal plexuses (see Fig. 120-1). In association with the muscle layers, the networks of ICC are recognized as pacemakers that activate neuromuscular function. The ENS consists of approximately 100 million neurons in higher mammals, roughly equal to the number of neurons in the spinal cord. Histologic and physiologic studies11–13 of the intestinal tract have characterized the properties of the neurons and transmitters mediating its functions, including the peristaltic reflex, and the neuroimmune interactions between neurons and inflammatory cells.
Precursor cells arise from three axial levels of the neural crest: vagal, rostral-truncal, and lumbosacral14 levels. The enteric neurons mainly arise from the vagal neural crest of the developing hindbrain and colonize the bowel by migration in a rostrocaudal direction. Vagal crest cells are not restricted to a particular intestinal region. Some enteric neurons arrive in the hindgut from the lumbosacral level via a caudorostral wave of colonization. Rarely, these migrating cells do not reach the entire bowel; usually this affects the terminal portion of the colon, as in the classical forms of Hirschsprung’s disease.15
Neural control of the intestines is covered in Chapters 97 and 98.
ILEUS
Ileus refers to the inhibition of propulsive intestinal motor activity in the absence of a mechanical obstruction. Abdominal or retroperitoneal surgery is the most common cause of ileus, which develops in essentially all such cases16 and is the focus of this section. Other causes of ileus are listed in Table 120-1 and include general anesthesia, medications, inflammation, infection, ischemia, and various metabolic and neurologic processes.
Infection |
The autonomic nervous system plays a key role in regulating gastrointestinal motility.17 Parasympathetic nerve activity stimulates intestinal motility by inducing the release of acetylcholine from excitatory neurons in the myenteric plexus. Sympathetic nerve activity inhibits activity by blocking the release of acetylcholine from the same excitatory fibers while also providing the efferent limb of multiple reflex pathways. Sympathetic neural input appears to be the main physiologic mechanism involved with impaired intestinal motility in the postoperative period. Afferent neural input caused by irritation (incision) or inflammation of the peritoneum results in an increase in inhibitory sympathetic efferent neural activity via the splanchnic nerves and an overall decrease in bowel activity.18 Sympathetic blockade does not entirely prevent the delayed intestinal motility induced by abdominal surgery, and so alternate mechanisms such as nonadrenergic, noncholinergic (NANC) nerves are believed to contribute to peripheral autonomic neuroeffector transmission and to influence postoperative ileus.19
Uncomplicated postoperative ileus resolves in a predictable fashion. The small intestine resumes activity within 24 hours, the stomach in 24 to 48 hours, and the colon generally within 72 hours.20,21 Ileus is an important cause of prolonged hospitalization and escalating costs associated with surgery.1 Determining when ileus has resolved depends on the endpoint chosen, and all endpoints have relative weaknesses. Return of bowel sounds requires frequent examination, and their presence does not ensure propulsive activity.21 Passage of flatus requires verbal feedback and a willingness of the patient to report such activity. Bowel movements may be the most reliable end point, but they represent distal bowel activity rather than global gastrointestinal function. Resolution of postoperative ileus is complete when oral intake is tolerated without pain, bloating, or emesis.
PATHOPHYSIOLOGY
The pathophysiology of postoperative ileus involves several mechanisms including disruption in autonomic neural pathways, release of neurohumoral stress mediators, intestinal inflammatory response, perioperative fluid excess, and various pharmacologic elements.19,22,23
Disrupted Neural Pathways
Parasympathetic (vagal) neural input stimulates intestinal motor activity, whereas sympathetic (splanchnic) input is inhibitory. Sympathetic activity is the predominant inhibitory influence on intestinal motility and provides the efferent limb of multiple reflex pathways. Sympathetic stimulation is a key factor in the development of postoperative ileus. Local stimuli trigger an inhibitory reflex that results in intestinal dilatation, for which the somatic and splanchnic nerves provide both the afferent and efferent pathways.24 Local stimuli include incising the abdominal wall and handling of the intestine; blocking sympathetic stimuli during surgery does not fully prevent such inhibition of intestinal motility.
NANC neurotransmitters also play a role in postoperative ileus. Nitric oxide (NO) may be the predominant NANC neurotransmitter that mediates the inhibitory influence of ENS neurons on smooth muscle. Other NANC neurotransmitters, including vasoactive intestinal peptide (VIP) and neuropeptide Y act through inhibitory pathways similar to the NO pathway to relax intestinal smooth muscle; substance P and adenosine triphosphate (ATP) act through excitatory motor pathways to influence postoperative ileus.25,26 NO inhibitors, and VIP and substance P antagonists decrease postoperative ileus in animal studies, but the potential usefulness of these agents in humans is unclear.18,25
Release of Neurohumoral Stress Mediators
Surgical stress and circulating inflammatory mediators activate pathways that trigger release of hypothalamic corticotrophin-releasing factor (CRF).27 CRF modulates feeding behavior under stressful conditions—explaining why anorexia is a common reaction to stress—and is considered essential in orchestrating the stress response.28 Experimental studies have shown that administration of CRF or CRF-related peptides delays gastric emptying and inhibits gastric motility29,30 similar to postoperative gastric ileus, whereas administration of the CRF1 antagonist, CP-154,526, blocks surgery-induced delay in gastric emptying.19
Abdominal surgery also stimulates capsaicin-sensitive afferent fibers, activating the inhibitory efferent pathways that disrupt coordinated intestinal motility.31 These fibers have been shown to play a crucial role in acute gastroprotection. Release of neurotransmitters such as calcitonin gene-related peptide (CGRP) and the related increase in mucosal blood flow have been identified as key factors in the protective effect of the stimulation of these fibers by capsaicin.32 Capsaicin-sensitive afferent nerves can influence ulcer healing by mediating the hyperemic response through the release of CGRP and facilitating acid disposal in the mucosa.32 Studies are ongoing to determine mechanisms of CRF activity and other stress mediators in the inhibition of gastrointestinal motility.
Intestinal Inflammation
Handling the intestine during surgery results in a marked inflammatory reaction,33–35 leading to induction of inducible nitric oxide synthase (iNOS) and cyclooxygenase (COX)-2 and the release of mast cells, monocytes, and neutrophils within the muscularis propria, but not the mucosa. Bowel handling also leads to secretion of numerous proinflammatory cytokines including interleukin (IL)-1β, IL-6, tumor necrosis factor (TNF)-α, and monocyte chemotactic protein (MCP)-1 and up-regulates intercellular adhesion molecule (ICAM)-1.36 Thus, extensive manipulation of the intestine or exposure of the peritoneum to irritants (e.g., pus or blood) increases the probability of developing postoperative ileus. The effect on intestinal transit appears to be proportional to the magnitude of the inflammatory response.37
In addition to autonomic signaling pathways, extrinsic noradrenergic nerves contribute to the regulation of various digestive functions, including intestinal propulsion, mucosal secretions, and bowel sensation, through activation of α2-adrenoceptors. In the presence of intestinal inflammation, enteric α2-adrenoceptors are up-regulated, giving enhanced inhibitory control of cholinergic and noradrenergic transmission.38,39 Located on neurons and inflammatory cells, α2-adrenoreceptors may be important in the pathogenesis of postoperative ileus, because their location provides a link between the inflammation induced by intestinal handling and activation of neuronal pathways.40
Perioperative Fluid Excesses
Intravenous fluid overload during or after surgery delays recovery of gastrointestinal function41 and is associated with poor survival and complications.42 In patients undergoing colon resection, use of perioperative and postoperative restricted fluid regimens has resulted in reduced hospital stays, faster return of gastrointestinal function, and fewer postoperative complications including reduced nausea and vomiting compared with higher-volume strategies.43
Pharmacologic Mechanisms
Anesthesia
All anesthetic agents have an inhibitory effect on intestinal motility, although the technique of administration can significantly influence the duration of postoperative ileus.44 Mid-thoracic (T6-T9) epidural anesthesia often is used as an adjunct to general anesthesia in abdominal operations. The technique allows local placement of the anesthetic for sympathectomy, which substantially reduces the severity and incidence of postoperative ileus44 and theoretically blocks afferent and efferent inhibitory reflexes associated with abdominal surgery.19,45 Local epidural anesthesia appears to increase splanchnic blood flow to the intestine, disrupt afferent inhibitory effects, and further reduce sympathetic neural input.18,46,47 Use of systemic opioid therapy or epidural opioids, regardless of whether administered by a low-thoracic or a low-lumbar route, does not shorten the duration of ileus compared with nonopioid epidural anesthetics.48,49
Opiates
Endogenous and exogenous opiates contribute significantly to the development of postoperative ileus.50,51 Of the three main classes of opiate receptors (µ, κ, δ) located in the central nervous system (CNS) and gastrointestinal tract,52 the CNS µ receptors mostly modulate analgesia within the brain and the spinal cord. Endogenous opioids released from neurons within the submucosal and myenteric plexus of the gastrointestinal tract modulate sphincteric and peristaltic activity in a coordinated fashion.53 Activation of intestinal µ receptors suppresses release of acetylcholine from cholinergic neurons, resulting in delayed intestinal motility.54
Exogenous opioids increase antral and proximal duodenal tone with an overall inhibitory effect on motility. The effect of morphine on the small intestine is biphasic, initially stimulating MMC activity followed by atony, which impedes propulsion and delays intestinal transit.55 In the colon, morphine increases the tone and amplitude of nonpropagating contractions, thus reducing propulsive activity and slowing transit. The overall effect of opiates is to decrease intestinal motility.
CLINICAL FEATURES
Factors that contribute to the development of ileus are listed in Table 120-1. Plain abdominal films can show air-filled stomach or distended loops of intestine or colon. Abdominal computed tomography (CT) can confirm the diagnosis while delineating extraluminal findings including abscess, retroperitoneal hematoma, pancreatitis, ascites, and inflammation, which might contribute to nonmechanical obstruction.
TREATMENT
Standard
Bowel rest neither shortens the time to first bowel movement nor decreases the time to oral intake. Large meals can induce nausea and bloating, but small meals can stimulate gastrointestinal motility and reduce the duration of postoperative ileus.56,57 Nasogastric tube decompression, once considered standard of care, is not recommended in routine abdominal operations because it does not hasten recovery from ileus.58,59 In fact, routine use of nasogastric decompression has been associated with a higher postoperative complication rate of atelectasis and aspiration pneumonia and prolonged hospitalization. Early mobilization after surgery is recommended to reduce complications of atelectasis, pneumonia, and deep venous thrombosis; however, there is little evidence that ambulation hastens resolution of ileus.60
Preventive
Operative Period
Nature of Surgery
When compared with open surgical procedures, laparoscopy decreases the duration of postoperative ileus.61 Theoretically, reducing the mechanisms known to cause postoperative ileus (see earlier) by making small incisions and minimizing tissue handling should improve surgical outcome. Circulating levels of cytokines (e.g., IL-1β and IL-6) and C-reactive protein are significantly lower after laparoscopy than after open abdominal procedures and may reflect reduced tissue injury.62 Return to a normal interdigestive pattern occurs 40% faster after laparoscopic colectomy compared with conventional colectomy, and time to first bowel movement is reduced 27%.63 Also, tolerance of oral feedings and improved transit occur earlier after laparoscopic resection.64
Anesthesia
Epidural anesthesia, but not general anesthesia, interrupts sympathetic outflow, blocking afferent and efferent mechanisms that trigger inhibitory reflexes and the CRF-mediated stress response that can restore metabolic activities and reduce postoperative morbidity.65 A significant reduction in the duration of ileus occurs in patients who receive epidural bupivacaine compared with those receiving epidural opioid.66–68 Epidural bupivacaine alone was superior to epidural bupivacaine combined with opioid in reducing the duration of ileus without compromising pain relief. Delivery of local anesthetic via a mid-thoracic (T6-T9) epidural catheter for abdominal surgery significantly reduces duration of ileus and hospitalization,18 whereas low thoracic and lumbar epidural deliveries do not show similar benefit.46,47 Local anesthesia may be more important in reducing postoperative ileus when used as an adjunct to open abdominal surgery rather than to laparoscopic surgery.69 The optimal duration of epidural anesthetic appears to be two to three days beyond the operation.70 Epidural anesthesia might not be necessary in laparoscopic colorectal surgery and, in some cases, it may be replaced by opioid-sparing multimodal analgesia, including oral acetaminophen, NSAIDs, systemic local anesthetics, or continuous infusion of the wound with local anesthetic.71,72
Perioperative Fluid Management
Several large trials have shown that excessive hydration in the perioperative period increases morbidity. Fluid excess, which can cause bowel edema42 and pulmonary compromise, is linked to prolonged postoperative ileus and extended hospital stay.73 Fluid management by perioperative optimization of hemodynamic function, known as goal-directed therapy, maximizes cardiac stroke volume by using small fluid challenges43,74 and has been shown to improve patient outcome. Avoiding fluid overload by using goal-directed therapy can reduce postoperative complications and facilitate rapid functional gastrointestinal recovery.43
Postoperative Period
Postoperative nausea and vomiting (PONV) occurs in 20% to 30% of low-risk surgical patients and in up to 80% of high-risk surgical patients.75 General anesthesia increases the risk of PONV nine-fold greater than the use of regional anesthesia.76 Reducing risk factors (Table 120-2) when possible and using prophylactic agents in patients at high risk for PONV can shorten the duration of ileus; low-risk patients are less likely to receive benefit. Dexamethasone, droperidol, and the 5-HT3 antagonist ondansetron, alone or in combination, can provide effective antiemetic prophylaxis against PONV77 in high-risk patients. Aprepitant is a substance P antagonist that acts on the neurokinin 1 receptor and is FDA approved for prevention of PONV.
Table 120-2 Risk Factors for Postoperative Nausea and Vomiting
Anesthesia |
Modified from Gan TJ, Meyer TA, Apfel CC, et al. Society for Ambulatory Anesthesia guidelines for the management of postoperative nausea and vomiting. Anesth Analg 2007; 105(6):1615-28.
Chewing Gum
Several studies show gum chewing in the postoperative period significantly reduces the time to first flatus and the time to first passage of feces compared with standard treatment alone,78,79 although the length of hospital stay is only marginally reduced. Chewing gum does not increase complications or readmissions and is a low-risk, inexpensive, first-line approach to resolving ileus after colorectal surgery.80
Early Oral Intake
Several randomized, controlled trials support early postoperative feeding (within 24 hours) in patients undergoing gastrointestinal surgery.81 Although early postoperative feeding can increase vomiting, mortality is reduced with a trend toward reduction of risk of postsurgical complications and shorter hospitalization. Early oral nutrition attenuates catabolism and reduces intestinal permeability that can lessen infectious complications.82,83 Despite ongoing controversy, studies suggest early postoperative feeding may be of benefit.
Drug Therapy
Opioid-Sparing Analgesia
Ketorolac tromethamine is an NSAID that can be administered parenterally, thereby circumventing the time lag accompanying postoperative oral intake. Ketorolac is equivalent to morphine in analgesic effect after major abdominal surgery without the functional gastrointestinal side effects of morphine.84 Ketorolac has anti-inflammatory and antipyretic activity and reduces postoperative ileus and morphine requirements.85
Continuous epidural infusion of lidocaine86 after colonic resection can reduce morphine use, improve pain relief, and hasten recovery. Ketamine, gabapentin, pregabalin, and neural blockade are recognized opioid-sparing agents or techniques with the potential to accelerate postoperative recovery; additional study is required because results of their effects are inconsistent.87 In addition, use of postoperative patient-controlled analgesia (PCA) that allows on-demand intravenous delivery may be as effective as continued epidural administration and can reduce medication dose and duration.88
Opioid Antagonists
Stimulation of µ-opioid receptors in the brain and intestine by morphine and other opioids imparts a potent central analgesic effect plus dose-limiting debilitating constipation and other unwelcome side effects.51 Therefore, effective blockade of peripheral opioid receptors in the gastrointestinal tract should manage opioid-related bowel dysfunction. Alvimopan and methylnaltrexone are selective µ-opioid antagonists with activity that is restricted to the peripheral receptors.
Alvimopan (12 mg, orally two hours before surgery, then twice daily for seven days) shortened the time to gastrointestinal recovery (passage of flatus, tolerance of solid food, first evacuation) by 15 to 18 hours and the hospital stay by one day following bowel resection.89 The effects of alvimopan are achieved without adverse effects on abdominal pain scores or patient-administered analgesia.89–92 The alvimopan studies used general (not epidural) anesthesia and nasogastric tubes up to 24 hours postoperatively; oral feeding was initiated at 48 hours postoperatively. Avoiding known risk factors and implementing measures to prevent postoperative ileus as it occurs in fast-track postoperative surgery can alter outcome.
Methylnaltrexone has been shown to reverse morphine-induced delay in gastric and orocecal transit time in healthy subjects.93 In patients with opioid-induced constipation with advanced illness, methylnaltrexone induced laxation and reduced symptoms of constipation.93
Prokinetic Agents
There are no available effective prokinetic agents for the treatment of postoperative ileus. Cisapride is a serotonin agonist that facilitates acetylcholine release from the intrinsic neural plexus with inconsistent effect on resolution of postoperative ileus. Cisapride was removed from the market because of potentially dangerous cardiac side effects, but it is available under a limited-access program through Janssen Pharmaceutica.94 Neostigmine and lidocaine require additional studies with clinically relevant outcomes to prove treatment effectiveness.92 Erythromycin has demonstrated a consistent absence of effect in hastening postoperative recovery in several prospective randomized clinical trials. Metoclopramide, a mixed cholinergic agonist and dopamine antagonist, shows only small or insignificant benefit in the treatment of postoperative ileus.92
Laxatives
There is some evidence to support the use of laxatives in the postoperative period. Osmotic and stimulant (suppository) laxatives appear safe and beneficial in producing early bowel evacuation and might shorten the duration of postoperative ileus. Small trials have examined the effect of bisacodyl suppository alone,95 bisacodyl in combination with milk of magnesia,96 and magnesium oxide combined with disodium phosphate,97 the latter used in a fast-track rehabilitation protocol following abdominal hysterectomy. No significant adverse events have been reported with any agent. High-quality prospective trials are needed.
Emerging Drugs
Lubiprostone, a chloride-2 channel activator that stimulates intestinal fluid secretion and increases intestinal transit, is under investigation as an agent to treat postoperative ileus. Other drugs emerging from trials or preclinical testing as potential agents for the treatment of postoperative ileus include linaclotide (a guanylate cyclase-C agonist), motilin agonists (KOS-2187, GM-611, GSK-326416), a neurokinin-2 receptor antagonist (MEN-11420), and growth hormone secretagogues (ipamorelin, TZP-101).31
Multimodal Approach to Preventing Postoperative Ileus
The advent of laparoscopic surgery, opioid-sparing analgesics, regional anesthesia, and other factors that hasten recovery from postoperative ileus (Table 120-3) has led to improved care of patients undergoing abdominal surgery. First described in the 1990s, enhanced recovery management strategies—fast-track surgery methods—combine treatment modalities that individually improve outcome following major surgery.98 An overarching goal of fast-track surgery is to lower rates of organ dysfunction, thereby reducing morbidity, hastening recovery, and shortening hospital stay.69 Some benefits of fast-track surgery are listed in Table 120-4. The concept has proved valid across all surgical specialties, but the most physiologic data are available for colonic surgery. Fast-track surgery has been shown to enhance recovery from postoperative ileus: More than 90% of patients have a normal oral intake, defecate within 48 hours, and have a hospital stay of two to four days (reduced from five to 10 days) after uncomplicated open colonic surgery.71,99,100
Table 120-3 Factors That Contribute to Enhanced or Delayed Recovery from Postoperative Ileus
FACTOR | RANDOMIZED CONTROLLED STUDIES (RCTs)* |
Enhances Recovery | |
Chewing gum | x |
Early oral feeding† | |
Goal-directed fluid therapy and avoidance of fluid overload | x |
Intravenous or wound (local) anesthetics | x |
Laparoscopic vs. open surgery|| | x |
Laxatives† | |
Length and/or type of incision‡ | |
Mobilization‡ | |
Peripheral opioid antagonists | x |
PONV antiemetic agents | x |
Thoracic epidural local anesthetics | x |
Delays Recovery | |
Administration of excess perioperative fluid | x |
Nasogastric tubes | x |
Opioids | x |
Restrictions on oral intake† |
PONV, postoperative nausea and vomiting.
* Based on two or more RCTs or meta-analyses.
† Preliminary studies are positive, but further studies are required before general recommendations are made.
‡ Data are limited or inconclusive.
|| Data are difficult to interpret because of the use of nasogastric tubes, use of opioid analgesia, and restrictions for oral intake in “open” groups.
Used with permission and adapted from Kehlet H. Postoperative ileus—an update on preventive techniques. Nat Clin Pract Gastroenterol Hepatol 2008; 5(10):552-558.
Table 120-4 Benefits of Fast-Track* Surgery
* Fast track refers to the use of enhanced recovery management strategies to improve outcomes after surgery.
ACUTE COLONIC PSEUDO-OBSTRUCTION
Acute colonic pseudo-obstruction, also known as Ogilvie’s syndrome,101 is characterized by acute massive colon dilatation involving primarily the right side of the colon and without a mechanical cause. It is most often diagnosed in hospitalized, debilitated medical or surgical patients with a wide array of medical conditions (Table 120-5). Ogilvie’s syndrome is estimated to occur in 0.1% of all surgical patients,3 and patient outcome depends on the severity of the underlying illness, the person’s age, the maximum diameter of the cecum, the delay until colonic decompression, and the presence of colonic ischemia.
Table 120-5 Conditions Commonly Associated with Acute Colonic Pseudo-obstruction
Cardiovascular |
Used with permission from De Giorgio R, Stanghellini V, Barbara G, et al. Prokinetics in the treatment of acute intestinal pseudo-obstruction. IDrugs 2004; 7(2):160-165.
PATHOPHYSIOLOGY
Disrupted autonomic nervous system activity is considered a key factor in the pathogenesis of acute colonic pseudo-obstruction. Parasympathetic (excitatory, causing contraction) innervation of the colon is from the vagus nerve, which supplies the right colon and extends to the splenic flexure; parasympathetic innervation of the distal colon and rectum is from the spinal supply sacral plexus. Sympathetic (inhibitory, causing relaxation) innervation of the colon is from the celiac and mesenteric ganglia (see Fig. 120-2). The current proposed mechanisms of acute colonic pseudo-obstruction are summarized in Table 120-6.
Table 120-6 Proposed Mechanisms for Acute Colonic Pseudo-obstruction
Intestine Fails to Contract |
Modified from Delgado-Aros S, Camilleri M. Pseudo-obstruction in the critically ill. In: Scholmerich J, editor. Bailliere’s best practice & research in clinical gastroenterology: gastrointestinal Disorders in the Critically Ill, vol. 17. London: Elsevier Science; 2003. pp. 427-44.
Local stimuli effect an inhibitory reflex that results in dilatation of the colon, for which the splanchnic nerves provide both the afferent and efferent pathways. Local stimuli, including peritoneal inflammation, infection, and handling of the colon, result in the release of mast cells, leukocytes, and monocytes.33–35 Inflammatory mediators such as ICAM-1, MCP-1, iNOS, and COX-2 are up-regulated during the first 18 hours after abdominal surgery, and the degree of postoperative intestinal impairment correlates with the intestinal inflammatory response.
The release of endogenous opioids after surgery has been related to the inflammation and impaired motor activity that characterize the physiologic response to surgery. Administration of antidepressant, phenothiazine, anti-parkinsonian, or narcotic medications can induce acute colonic pseudo-obstruction.102 Opioids inhibit release of NO from inhibitory motor neurons in vitro and delay transit in vivo.
CLINICAL FEATURES
Acute colonic pseudo-obstruction typically occurs in older (mean age, 60 years) men who are hospitalized or institutionalized (60%) with severe underlying medical or surgical conditions (see Table 120-5).
Symptoms and Signs
The most characteristic feature of acute colonic pseudo-obstruction is abdominal distention, which can develop gradually over three to seven days or more acutely within 24 hours. About 60% of patients experience nausea and vomiting. Abdominal pain (80%) tends to be mild and constant, with occasional slight rebound tenderness and painless distention.103 Low-grade fever may be present. Disturbances of electrolyte levels occur, notably hypokalemia, hypocalcemia, hyponatremia, and hypomagnesemia. New abdominal pain or tenderness, increasing fever, and increasing white blood cell count are features of ischemia or perforation. About 90% of patients have abnormal bowel sounds, which vary from absent to hyperactive. In many cases, the abnormal bowel sounds are high-pitched and suggest mechanical obstruction.2
Radiology
The most distinctive feature of acute colonic pseudo-obstruction on a plain abdominal film is dilatation of the colon that preferentially affects the right side of the colon. The maximal diameter of the cecum typically ranges from nine to 25 cm, often with a cutoff sign at the hepatic or splenic flexure (Fig. 120-3). The left colon, including the rectosigmoid, and the small bowel also may be dilated. Air-fluid levels can be seen in the small intestine but usually do not occur in the colon. Haustral folds often are visualized despite severe distention.
PROGNOSIS
The mortality rate of patients with acute colonic pseudo-obstruction varies from 0% to 32% and is partly determined by their comorbidity.3,104 Older patients, poor clinical condition, and surgical treatment for acute colonic pseudo-obstruction are associated with an increased risk of mortality. There are no randomized clinical trials comparing surgical and medical treatment to clarify whether surgery itself or selection bias influences mortality associated with surgical treatment. Intestinal ischemia or perforation, which supervenes in about one in every six or seven cases, is associated with a 40% increase in the risk of death.3
The diameter of the colon is also a risk factor for mortality. When surgical decompression is utilized in mechanically obstructed patients with a cecal diameter greater than nine centimeters, there is a dramatic reduction in mortality,105 which is the basis for the nine-centimeter cutoff as a sign of impending perforation in patients with acute colonic pseudo-obstruction. In one study, perforation rates for cecal diameters less than 12 cm, 12 to 14 cm, or larger than 14 cm were 0%, 7%, and 23%, respectively.3 Mortality also was associated with delay in decompression: 15% in those decompressed less than four days after onset of dilatation; 27% when the decompression occurred after four to seven days; and 73% after day seven.
PREVENTION
Minimally invasive surgery, thoracic epidural anesthesia, and nominal use of opioid analgesia have led to improved care of surgical patients. Enhanced operative recovery programs, referred to as multimodal or fast-track programs, combine modalities that individually have a lower morbidity compared with standard treatment.72,106 Methods used in some fast-track programs are listed in Table 120-7.69 Studies have shown various fast-track programs can reduce the duration of ileus and the costs and duration of hospital stay while decreasing cardiopulmonary morbidity and postoperative convalescence.
Table 120-7 Some Methods Used in Fast-Track* Rehabilitation Programs
* Fast track refers to the use of enhanced recovery management strategies to improve outcomes after surgery.
Modified from Kehlet H. Fast-track colorectal surgery. Lancet 2008; 371(9615):791-3.
TREATMENT
The goal of management is to achieve colonic decompression. With correction of reversible potential causes or associated imbalances (e.g., infection, hypovolemia, hypoxemia, electrolyte levels), discontinuation of medications that can induce ileus (e.g., anticholinergics, opiates), the ileus disappears by day six in 83% to 96% of patients.3 Intravenous saline and glucose solutions suffice for hydration because of the short-lived and reversible nature of the dysfunction in most cases; in patients with prolonged acute colonic pseudo-obstruction, parenteral or enteral nutrition may be necessary.107 Nasogastric aspiration for nausea and vomiting may be beneficial, but often it is ineffective because the functional obstruction affects the colon. Rectal tubes may be useful if the sigmoid colon is dilated. Enemas might “cleanse” the colon, but only Gastrografin enemas have shown efficacy in anecdotal reports. Enema use has been complicated by colonic perforation.108 When the diameter of the cecum is greater than nine centimeters and has not responded to treatment within the first 72 hours after diagnosis, decompression should be performed to reduce the risk of ischemia, perforation and death.3 A treatment algorithm is shown in Figure 120-4.
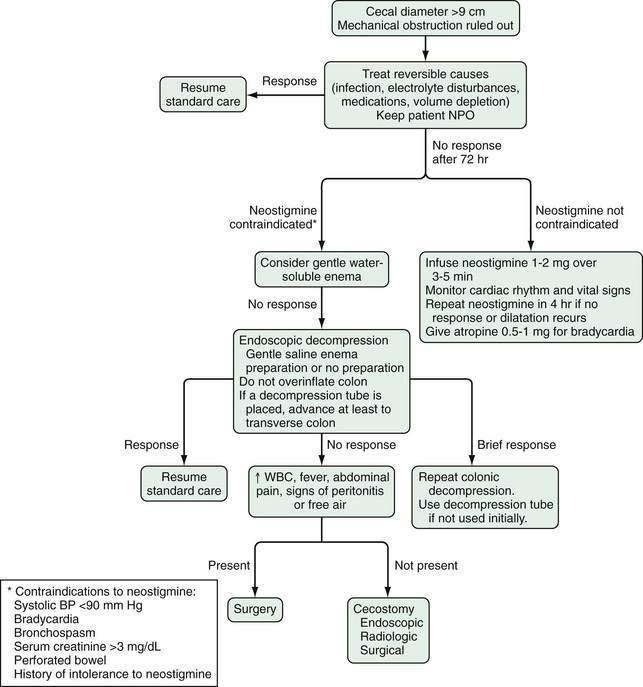
Figure 120-4. Algorithm for the treatment of acute colonic pseudo-obstruction. NPO, nothing by mouth; WBC, white blood cell count.
Medical Decompression
Adrenergic blockers and the acetylcholinesterase inhibitor neostigmine have been tested in open-label studies. Only neostigmine has been tested in a randomized, controlled trials and has an effective initial treatment response rate of 60% to 90%.109,110
5-HT4 receptor agonists, motilin receptor agonists, and muscarinic receptor agonists have been the subject of anecdotal reports, but none have been formally tested in the setting of acute colonic pseudo-obstruction.108 Metoclopramide has shown very small or insignificant benefit in the treatment of postoperative ileus.92 The peripherally acting µ-opioid receptor antagonist alvimopan significantly shortens the duration of postoperative ileus, but neither metoclopramide nor alvimopan has been sufficiently tested as treatment for acute colonic pseudo-obstruction.89–92
Endoscopic Decompression
Randomized, controlled trials of endoscopic decompression are lacking. Colonoscopic decompression can be achieved technically in 80% of patients with acute colonic pseudo-obstruction, albeit with a high risk of cecal perforation.110 The complication rate ranges from about 1% to 5%,5 with a 3% perforation rate.104 Colonic decompression has not been shown to improve the outcome of these patients. It has a high recurrence rate and colonoscopy of unprepared bowel can distend the colon further and result in perforation.3 Placement of a decompression tube into either the right or transverse colon has a similar technical success rate.104 Endoscopic decompression should be considered in patients with a high risk of cecal perforation when conservative and pharmacologic maneuvers have failed.
Percutaneous Cecostomy
In view of the high rate of recurrence of colonic dilatation after endoscopic decompression, alternative techniques to decompress the colon have been proposed to avoid surgery. Percutaneous cecostomy has been reported to be successful in several case reports and one small case series, but it is associated with significant morbidity. Percutaneous cecostomy can be performed endoscopically, radiographically, or surgically.111,112
Surgical Decompression
Surgical decompression, which includes cecostomy, colostomy, or resection, is associated with a 6% morbidity and a 30% mortality overall.3 It is used for patients with more-severe disease who have failed to respond to conservative or other measures. In cases of ischemia or perforation, segmental or subtotal colectomy is indicated.111
CHRONIC INTESTINAL PSEUDO-OBSTRUCTION
PATHOPHYSIOLOGY
Causes of chronic pseudo-obstruction are classified as either primary or secondary, but in most cases a cause is never determined. When a cause is found, it most often is from an underlying and potentially reversible condition affecting the intrinsic or extrinsic enteric neural pathways (neuropathies), the ICC (mesenchymopathies), or the intestinal smooth muscle cells (myopathies) (Table 120-8).113 Overlap, such as neuropathy with features of myopathy or neuropathy plus mesenchymopathy, can occur. Familial causes are rare and are far outnumbered by sporadic and secondary causes. When a thorough diagnostic evaluation fails to reveal a cause, obtaining a full-thickness tissue specimen by laparoscopy with subsequent specific pathologic testing, including detailed light microscopic evaluation and ultrastructural analysis, may aid in making a diagnosis (Table 120-9).114
Table 120-8 Classification of Chronic Intestinal Pseudo-obstruction According to Etiology
From Sutton DH, Harrell SP, Wo JM. Diagnosis and management of adult patients with chronic intestinal pseudoobstruction. Nutr Clin Pract 2006; 21(1):16-22.
Table 120-9 Markers for and Targets of Intestinal Neuropathy
MARKERS | CELL TARGETS AND SITES | DESCRIPTION |
---|---|---|
PGP9.5, NSE, MAP-2 NFs, tubulins, Hu C/D | Neurons: membrane and cytoplasmic | Identification of the general structure of the ENS |
B-S-100, GFAP | Glial cells: cytoplasmic | Detection of enteroglial cells |
C-Kit | ICCs: membrane and cytoplasmic | Different ICC networks |
Substance P, VIP, PACAP, CGRP, neuropeptide Y, galanin, 5-HT, NOS, ChAT, somatostatin, calbindin, NeuN; NK1, NK2, NK3 | Subclasses of enteric neurons; ICC: membrane and cytoplasmic | Characterization of neurochemical coding and enteric neuron subclasses; subsets of ICC |
Bcl-2, TUNEL, caspase-3, caspase-8, APAF1 | Apoptosis and related mechanisms: nuclear and cytoplasmic | Assessment of apoptosis and related pathways |
Actin, myosin, desmin, smoothelin | Smooth muscle cells: cytoplasmic | Assessment of smooth muscle integrity |
CD3, CD4, CD8, CD79α, CD68; MIP-1α, TNF-α, IFN-γ | Immune cells, chemokines, and cytokines: membrane and cytoplasmic | Evaluation of B (CD79α) and T lymphocytes (CD3), T-helper (CD4) cells, T-suppressor (CD8) cells, macrophages (CD68) in enteric ganglionitis; MIP-1α is a chemokine; TNF-α and IFN-γ are inflammatory cytokines |
APAF1, apoptotic peptidase activating factor 1; Bcl-2, B cell lymphoma-2 protein; ChAT, choline acetyltransferase; ENS, enteric nervous system; CGRP, calcitonin gene-related peptide; GFAP, glial fibrillary acidic protein; 5-HT, 5-hydroxytryptamine (serotonin); Hu C/D, Hu C/D molecular antigen; ICC, interstitial cells of Cajal; IFN-γ, interferon γ; MAP-2, microtubule associated protein-2; MIP1-α, macrophage inflammatory protein 1-α; NeuN, neuronal-specific nuclear protein; NF, neurofilament; NK, neurokinin; NOS, nitric oxide synthase; NSE, neuron-specific enolase; PACAP, pituitary adenylate cyclase activating polypeptide; PGP9.5, protein gene product 9.5; TNF-α, tumor necrosis factor α; TUNEL, terminal deoxynucleotidyl transferase–mediated doxyuridine triphosphate nick-end labeling; VIP, vasoactive intestinal polypeptide.
From Antonucci A, Fronzoni L, Cogliandro L, et al. Chronic intestinal pseudo-obstruction. World J Gastroenterol 2008; 14(19):2953-61.
Enteric Neuropathies
Inflammatory neuropathies that cause chronic pseudo-obstruction may be primary or secondary to a variety of infectious, paraneoplastic, and neurologic disorders. Inflammatory neuropathies are characterized by an intense inflammatory infiltrate of CD4 and CD8 lymphocytes that are CD3 positive and confined to the myenteric plexus.115,116 The juxtaposition of lymphocytes that are CD3 positive with myenteric neurons is the foundation by which immunologic interactions affect ganglion cell structure and survival.114,117 In the gastrointestinal tract, inflammation and immune activity have been shown to significantly influence the morphology and function of the enteric nerves.
Many patients with intestinal dysfunction secondary to paraneoplastic visceral neuropathy exhibit circulating antineuronal autoantibodies, such as anti-Hu antineuronal antibodies; autoantibodies reinforce a probable role of the immune system in neuronal dysfunction.112 Experimentally, these autoantibodies can elicit neuronal hyperexcitability118 and modify the ascending reflex pathway of peristalsis.113 When incubated with a primary culture of myenteric neurons, anti-Hu neuronal antibodies have been shown to trigger activity of caspase-3 and apaf-1 together with apoptosis,119 which implies that anti-Hu antibodies contribute in a direct way to the lymphocytic infiltrate in ENS dysfunction and degeneration observed in patients with inflammatory neuropathy. Indication of viral infection, such as herpes virus DNA, has been isolated deep in the myenteric plexus of some patients with severe neuropathic intestinal dysmotility, lending evidence to viral infectious agents as a cause of inflammatory ganglionitis.120 Eosinophilic ganglionitis has been recognized in some pediatric patients with pseudo-obstruction,121 although in these cases, eosinophilic ganglionitis did not appear to cause neuronal deterioration or loss.113 Also, mast cell ganglionitis has been described in patients with severe intestinal dysfunction122 and is associated with a reduced nitric oxide synthase expression, suggesting impaired inhibitory innervation of the ENS.
Noninflammatory (degenerative) neuropathies may be familial or sporadic and the result of dysfunctional mitochondria, altered calcium signaling, and accumulation of free radicals that leads to eventual degeneration and loss of neurons.123 Sporadic cases can be primary or secondary to a wide range of conditions including radiation, diabetes mellitus, amyloidosis, myxedema, and drug toxicity.113 Two predominant pathologic patterns have emerged in visceral neuropathy from degenerative sporadic causes. One pattern shows a reduction in the number of intramural cells that occur in relation to swollen processes and nerve cell bodies, an increase in glial cells, and fragmentation and loss of axons; in the second pattern, in the absence of dendritic swelling or glial proliferation there is a loss of the normal staining that occurs in subsets of enteric neurons.113,114,124 Severe forms of idiopathic intestinal intrinsic neuropathy have been associated with a decreased expression of the protein encoded by Bcl-2, a gene related to one of the intracellular pathways leading to apoptosis.125–127
Enteric Mesenchymopathies
The ICC are derived from mesenchymal cells that express c-kit. ICC are important for pacing electrical slow-wave activity and for gastrointestinal motor contractions128,129 (see Chapters 97 and 98). Spindle-shaped ICC are distributed within the muscularis (ICC-IM), and network-forming ICC are closely associated with the myenteric plexus (ICC-MY) of the ENS. The division of morphologically distinct classes of ICC within different layers of the GI musculature suggests that different ICC can perform discrete physiologic roles in intestinal motility.130
ICC are closely apposed to nerve terminals and electrically coupled via gap junctions to neighboring smooth muscle cells. Studies indicate they play a fundamental role in the reception and transduction of both inhibitory and excitatory enteric motor neurotransmission.131 Confocal electron microscopy shows abnormalities of ICC in patients with chronic intestinal pseudo-obstruction that include irregular cell surface markings,132 damaged intracellular organelles and cytoskeleton, and decreased ICC density.113,133 As a result, it has been proposed that ICC involved in pacemaker activity and neurotransmission to smooth muscle can contribute to the enteric motility abnormalities detectable in patients with chronic pseudo-obstruction.
Enteric Myopathies
Smooth muscle fibrosis and vacuolization are histologic abnormalities that have been observed in the circular and longitudinal muscle layers of some patients with primary visceral myopathies.124,134 In a large series of well-characterized patients with chronic pseudo-obstruction, a deficiency of one isoform of the cytoskeletal smooth muscle protein actin, α-actin, was described in approximately 25% of patients with the myopathic phenotype.135 The authors proposed that a selective decrease or absence of α-actin staining in the circular muscle of the small bowel wall could be a biologic marker of chronic intestinal pseudo-obstruction,135 although controlled studies have not yet been done.
PRIMARY CAUSES
Sporadic Nonfamilial Visceral Neuropathy
Also known as chronic idiopathic intestinal pseudo-obstruction (CIIPO), sporadic, nonfamilial visceral neuropathy can result from injury to the myenteric plexus from various causes including drug toxicity, ischemia, radiation, or viral infections such as cytomegalovirus, Epstein-Barr virus, and herpes simplex virus.136 CIIPO is the most common diagnosis given to cases of intestinal pseudo-obstruction, followed by various secondary causes that are covered later. Delayed gastric emptying and intestinal dysmotility have been observed in patients after viral gastroenteritis, which is associated with permanent damage to the myenteric plexus in some patients. Kamm and colleagues have documented such a syndrome in association with herpes viral elements in the myenteric plexus of patients with pseudo-obstruction.120
Patients with CIIPO often have disturbed motility of the entire gastrointestinal tract. The intestine may be dilated. Histologic examination of the myenteric plexus may reveal a reduction in the number or an abnormal morphology of neurons, which may be enlarged with thick, irregular clubbed processes. Hypertrophy of one or both muscle layers of the muscularis propria has been described. Intestinal phasic pressure studies are abnormal, but routine histology findings often were normal in patients whose tissue was available for analysis.137
Familial Intestinal Pseudo-obstruction
Primary familial cases of intestinal pseudo-obstruction result from abnormalities of smooth muscle cells of the muscularis propria (familial visceral myopathies) or abnormalities of the enteric neuronal structures (familial visceral enteropathies). These are rare genetic disorders with autosomal dominant, autosomal recessive, or X-linked transmission.115 Abnormal genes and loci have been recognized in various syndromic forms of CIIPO, including the DNA polymerase gamma gene (POLG) on chromosome 21 (21q17), the transcription factor SOX10 on chromosome 22 (22p12), and a locus on chromosome 8.113,115,116,130 X-linked recessive forms of CIIPO have been mapped to Xq28I, and a large cytoskeletal protein filamin A has been identified as vital for proper enteric neuron development.136 Some familial disorders have been well characterized based on the pattern of inheritance and predominant abnormalities.
Familial Visceral Myopathies
Type II, called MNGIE (mitochondrial DNA neurogastrointestinal encephalopathy) is the best-characterized myopathic phenotype, although the pathogenetic mechanisms causing its intestinal dysmotility remain unclear. It is an autosomal recessive mitochondrial encephalopathy that can lead to chronic pseudo-obstruction because of dysfunction of the mitochondrial respiratory chain.138 MNGIE is characterized by exacerbations with severe symptoms on a background of chronic intestinal pseudo-obstruction with mildly dilated small intestine and dilated stomach, lactic acidosis, ptosis, ophthalmic paralysis, peripheral neuropathy, alterations in the white matter on magnetic resonance imaging (MRI) of the brain, skeletal muscle ragged red fibers (Fig. 120-5), and specific mitochondrial changes at the ultrastructural level.138,139 Patients manifest skeletal muscle pain and cramps and lactic acidosis with elevated circulating muscle enzyme levels, including creatine phosphokinase (CPK), alanine transferase (ALT), and aldolase. Small intestinal diverticulosis complicated by inflammation and perforation is the cause of death in a majority of these patients in early adulthood.138
MNGIE syndrome results from mutations in the thymidine phosphorylase gene, which leads to markedly reduced thymidine phosphorylase activity that, in turn, results in accumulation of thymidine (dThd) and deoxyuridine (dUrd) in blood and tissues.140 High levels of dThd and dUrd lead to nucleoside pool imbalances that cause abnormalities in DNA including depletion, point mutations, and multiple deletions.140,141 A link has been established between DNA depletion and myopathic changes involving the external layer of the muscularis propria.138 Screening tests for MNGIE include measurements of serum lactic acid, circulating muscle enzymes, and thymidine phosphorylase in circulating leukocytes.142
Familial Visceral Neuropathies
FVNs are rare genetic diseases characterized by degeneration of the neural structures of the myenteric plexus. There are at least two distinct phenotypes of FVN.143 Type I is autosomal dominant, results in segmental dilatation of varying lengths of the small intestine, megacolon, and gastroparesis and has its onset of gastrointestinal symptoms at any age; more than 75% of patients with FVN type I have gastrointestinal symptoms. Histology demonstrates degeneration of argyrophilic neurons and reduced numbers of nerve fibers. Type II FVN is autosomal recessive and its findings include hypertrophic pyloric stenosis, malrotation of the small intestine, and short and dilated small intestine; CNS malformation and patent ductus arteriosis are seen in some cases. Onset of symptoms is in infancy, and histologically there is a deficiency of argyrophilic neurons and increased numbers of neuroblasts. There is no effective treatment (medical or surgical) for type II FVN, and prognosis is poor.
Several syndromic congenital neuropathies exist that can be classified broadly144 as:
Hirschsprung’s disease, perhaps the most common congenital neuropathy, occurs in one in 5000 live births (see Chapter 96). It is often associated with chromosomal abnormalities, the most frequent being trisomy 21 (Down syndrome) and affecting 2% to 10% of patients. Mutations in gene-encoding receptors or ligands, such as GDNF-Ret or ET-3-ETB, can occur. Mutations in the receptors are much more commonly encountered than mutations in the ligands. Different mechanisms can cause the terminal colon to become aganglionic: A deficiency of GDNF-Ret that is less severe and does not cause the entire bowel to become aganglionic; a deficiency of ET-3-ETB in which neural crest-derived cells differentiate prematurely and precursors cease dividing prematurely, leaving the last segment uncolonized; and other syndromes that result from mutations of transcription factors.
Waardenburg syndrome (WS) is an autosomal dominant familial disorder that affects body pigments and has a sensorineural hearing loss.145 It occurs with an incidence of 1/50,000 live births and accounts for 2% to 5% of congenital hearing loss. There are at least four types of the syndrome, and four genes have been identified in this condition: PAX3, MITF, EDNRB, and EDN3. The combination of Hirschsprung’s disease with WS defines the WS4 type.146 Patients with a Sox10 mutation and WS4 can present with ataxia, central and peripheral demyelinating neuropathies, and megacolon.147
Multiple endocrine neoplasia type 2B syndrome (MEN 2B) is a severe congenital familial neuropathic condition transmitted as an autosomal dominant trait and characterized by medullary carcinoma and tumor development in the neuroendocrine system (see Chapter 122). Presenting symptoms can include severe constipation or megacolon, diarrhea (when associated with enterocolitis), or obstruction, typically occurring shortly after birth.144 External stigmata of MEN 2B include a characteristic facies with “blubbery” lips due to mucosal neuromas that involve the lips, tongue, face, and eyelids (Fig. 120-6)148; marfanoid habitus; medullated corneal nerve fibers; and medullary thyroid carcinoma. Biopsies characteristically show findings of transmural ganglioneuromatosis with massive proliferation of neural tissue, including neurons, supporting cells; nerve fibers appear as thickened nerve trunks among mature nerve cells.
Childhood Visceral Myopathies
CVMs have been recognized as two distinctive forms of disease that differ from FVM in their mode of inheritance and clinical presentations (Table 120-10). Degeneration and fibrosis of gastrointestinal and urinary smooth muscle can be detected in both types of CVM and result in obstructive symptoms, bowel dilatation, and hydroureteronephrosis or megacystis, the latter resulting from degeneration of bladder muscle.149
Table 120-10 Classification of Childhood Visceral Myopathies
CHARACTERISTIC(S) | TYPE I | TYPE II* |
---|---|---|
Mode of transmission | Autosomal recessive (?) | Autosomal recessive (?) |
Clinical features | ||
Age of onset | Infancy and young childhood | Infancy |
Gender | Both | Predominantly female |
Symptoms | Constipation, distention ± chronic pseudo-obstruction | Obstipation, intestinal pseudo-obstruction |
Extragastrointestinal features | Megacystis and megaureters | Megacystis and megaureters |
Gross lesions | Dilatation of entire GI tract | Short, malrotated small intestine and malfixation of microcolon |
Histologic changes | Degeneration and fibrosis of GI and urinary smooth muscle cells | Vacuolar degeneration of GI and urinary smooth muscle cells |
Treatment, prognosis | No effective Rx; prognosis poor | No effective Rx; prognosis poor |
GI, gastrointestinal; Rx, treatment.
* Megacystis-microcolon-intestinal hypoperistalsis.
Camilleri M. Acute and chronic pseudo-obstruction. In: Feldman M, Friedman LS, Brandt LJ, editors. Sleisenger and Fordtran’s Gastrointestinal and liver disease: pathophysiology, Diagnosis, Management. 8th ed. Philadelphia: Saunders Elsevier; 2006. pp 2679-2702.
SECONDARY CAUSES
Systemic Sclerosis (Scleroderma)
Systemic sclerosis (SSc) is a generalized disorder of the microvasculature and connective tissue that results in increased deposition of collagen and other matrix elements, leading to thickening and fibrosis of the skin, with involvement of synovia and fibrosis of intestinal organs. The highest incidence of SSc occurs between 45 and 55 years of age. The gastrointestinal tract is the second most common target organ after the skin and the esophagus is the most commonly affected gastrointestinal organ, followed by the small intestine.150 Small bowel dysmotility leads to bacterial overgrowth resulting in steatorrhea, malabsorption, and weight loss.
Intestinal and gastric involvement can be identified radiologically in up to 40% of patients. Contrast studies can reveal dilatation of the duodenum and jejunum, along with fixed, narrow, tightly packed valvulae conniventes, despite bowel dilatation, producing an accordion appearance (Fig. 120-7) or a “hide-bound” appearance.151 Wide-necked diverticula are seen more often in the colon than in the small intestine.
Disturbances of small bowel motility152,153 result in delayed gastric or small bowel transit154 and include absence or hypomotility of the interdigestive MMC, low-amplitude clusters of propagated and nonpropagated contractions, a prolonged MMC cycle, diminished activity of phase III,153 and antral hypomotility (characterized by low-amplitude contractions, typically less than 40 mm Hg). Intestinal involvement usually causes fasting and postprandial contractile amplitude to fall below 10 mm Hg.155 Gastric emptying may be delayed by resistance to flow in the hypomotile small intestine, even if the stomach itself is unaffected. SSc patients without gastrointestinal involvement demonstrate normal small bowel manometry155 and normal intestinal transit time.
Dermatomyositis and Polymyositis
The gastrointestinal tract is involved in half of the cases of dermatomyositis and polymyositis. Dysphagia is often the presenting symptom, associated with weakness and atrophy of skeletal muscles of the esophagus. Megaduodenum and delayed intestinal transit are prominent features of chronic pseudo-obstruction. Histopathology demonstrates atrophy and fibrosis of intestinal smooth muscle, most consistent with a visceral myopathy.155
Systemic Lupus Erythematosus
Abdominal pain is the most common gastrointestinal complaint, which occurs in up to 20% of patients. Autopsy studies reveal a 65% prevalence of peritoneal inflammation, although documented cases of serositis as a cause of abdominal pain are rare. Smooth muscle dysfunction related to ischemia can lead to dilatation of the small intestine. Diarrhea occurs in 10% of lupus patients. Chronic intestinal pseudo-obstruction can occur as the presenting symptom and is potentially reversible with early treatment.156
Diabetes Mellitus
Patients with diabetes mellitus often have diarrhea, which may be due to bacterial overgrowth, pancreatic exocrine insufficiency, bile salt malabsorption, impaired absorption or secretion, loss of adrenergic sympathetic innervation, or disturbed motility. In patients presenting with symptoms of gastroparesis, the small intestine also may be affected, and such involvement can be documented by prolonged transit,154 by manometry,157 or sometimes by evidence of a dilated intestine.
Motility studies of the small intestine in patients with diabetes have varied significantly. Normal MMCs are found in many patients with documented gastroparesis, but absence of intestinal phase III has been demonstrated in some. Other abnormalities include MMCs that originate in the distal duodenum or jejunum and uncoordinated bursts of nonpropagated contractions (Fig. 120-8).158 The clinical relevance of these abnormal findings is uncertain. Demyelination of the proximal vagus nerve and sympathetic nerves supplying the bowel occurs in patients with long-standing diabetes. The intrinsic nervous system of the intestine does not appear to be affected because no morphologic abnormalities of the myenteric or submucosal plexuses have been observed; however, animal models and a single case report of a patient requiring transplantation of the pancreas and kidneys was associated with degeneration of the ICC.157 Although thickening of the small bowel muscle layers and eosinophilic hyaline bodies in smooth muscle cells have been observed,159 most authorities believe that myopathy is not a cause of gastrointestinal dysmotility in diabetic patients.
Parkinson’s Disease
Patients with Parkinson’s disease often have symptoms of gastrointestinal dysfunction. Proximal dysphagia, bloating, constipation, and difficulty with evacuation of stool occur commonly.160 Dilatation of the small bowel may be seen radiologically.161 Small intestinal dysmotility does occur, but its frequency is not known, and the contribution of these abnormalities to symptoms is not clear. Compared with controls, manometric studies in patients with Parkinson’s disease reveal infrequent or absent MMCs, hypomotility in the fed state, and an increased incidence of retrograde and tonic contractions.162
The pathogenesis of small intestinal dysmotility in Parkinson’s disease has not been determined. Lewy bodies are neurons containing cytoplasmic hyaline inclusions that originally were identified in the brain of parkinsonian patients. They have not yet been reported in the small intestine but have been found in the myenteric plexus of the esophagus and colon.163 Dopaminergic neurons are reported to be deficient in colons from patients with Parkinson’s disease and constipation.164
Spinal Cord Injury
Spinal cord injury usually produces only mild and probably insignificant dysmotility in the small intestine. The only documented changes in manometric studies performed in a group of patients with spinal cord injury were a greater number of phase III contractions that began in the duodenum rather than in the antrum in patients with high spinal cord lesions.165 Patients with injury to the lower spinal cord demonstrated no abnormalities, a finding consistent with the preservation of innervation to the intestine from the vagus and third thoracic sympathetic levels. One report described a woman with cervical spinal stenosis and paraplegia who displayed normal MMCs but a two-fold to three-fold prolonged interval between MMCs.
Colonic dysmotility is well recognized and is responsible for the common problems of constipation and difficulty of evacuation in patients with spinal cord injury.166 Spinal cord injury decreases colonic motility; though the postprandial colonic response is present, it is suboptimal and confined to the descending colon.167 In these patients, rectal compliance and resting anal sphincter pressures are lower than normal values, and ramp rectal inflation demonstrates patterns of sphincter activity similar to those recorded in the patients’ cystometrograms. There is no definite relationship of bowel function to the findings on anorectal manometry in patients with spinal cord injury.
Rehabilitation goals include continence of stool, simple voluntary independent defecation, and prevention of gastrointestinal complications. Individualized person-centered bowel care includes diet, laxatives, enemas, suppositories, and scheduling of bowel care to initiate defecation and accomplish fecal evacuation. Digital-rectal stimulation is a technique used during bowel care of patients with spinal cord injury to open the anal sphincter and facilitate reflex peristalsis.168
Neurofibromatosis (von Recklinghausen’s Disease)
Gastrointestinal involvement in neurofibromatosis is estimated to occur in 11% to 25% of patients.169 Small bowel dysmotility and intestinal pseudo-obstruction due to neurofibromatosis, however, are rare. Involvement of the intestine occurs in three principal forms: hyperplasia of the submucosal and myenteric nerve plexuses and mucosal ganglioneuromatosis, which can lead to disordered intestinal motility and chronic pseudo-obstruction; GIST tumors that show varying degrees of neural or smooth muscle differentiation and typically manifest with bleeding; and a glandular, somatostatin-rich carcinoid of the periampullary region of the duodenum, which also has been associated with pheochromocytoma.170
Paraneoplastic Visceral Neuropathies
Paraneoplastic neurologic syndrome is a remote effect of cancer that results in (visceral) neuropathy. It is not caused by the tumor or metastases, and it is not caused by infection, ischemia, or metabolic disruptions.171 Paraneoplastic neurologic syndrome is caused by autoimmune processes triggered by the cancer and directed against antigens common to both the cancer and the nervous system, designated as onconeural antigens. Paraneoplastic neurologic syndrome is rare, occurring in less than 0.01% of patients with cancer172; the Lambert-Eaton myasthenic syndrome is more common, occurring in nearly 1% of patients with small-cell lung cancer.173 Less than 50% of patients have detectable antibodies and up to 10% have an atypical antibody that is not well characterized.171 Paraneoplastic neurologic syndrome is considered to be mediated by the immune system, so suppression of the immune response represents another potential treatment approach.171 Associated neoplasms include small cell lung cancer (SCLC), thymoma, gynecologic and breast tumors, Hodgkin’s lymphoma, multiple myeloma, and colon cancer.
The most commonly associated tumor is SCLC, and the most common antibodies are Hu-Ab or CV2-Ab.171 Experimental evidence suggests that anti-Hu antibodies can exert a direct pathogenic role or can contribute, in association with the lymphocytic infiltrate, to ENS dysfunction in patients with chronic intestinal pseudo-obstruction related to an inflammatory neuropathy.113 Some patients with subacute parasympathetic and sympathetic autonomic failure and prominent gastrointestinal dysfunction also have antibodies directed against the neuronal autonomic ganglion type of acetylcholine receptors (nAchR antibodies). In these cases, the main associated tumors are thymoma and SCLC.
Patients with paraneoplastic neurologic syndrome can experience weight loss, persistent bloating, and abdominal distention from damage to the neurons of the enteric plexuses.171 Some patients present with dysphagia, nausea, and vomiting due to esophageal dysmotility or gastroparesis, or, more commonly, they present with symptoms of severe constipation. Radiologic studies can show dilatation of the small bowel, colon, or stomach.171 Esophageal manometry can reveal spasm or achalasia.
These inflammatory neuropathies are characterized by a dense inflammatory infiltrate with CD3-positive CD4 and CD8 lymphocytes that almost invariably are confined to the myenteric plexus.115,116,130
Myotonic Dystrophy
Myotonic muscular dystrophy is a slowly progressive disease characterized by myotonia, or difficulty in muscle relaxation. Diarrhea and abdominal cramping occur in up to one third of affected persons, malabsorption and steatorrhea have been reported in a few cases, and constipation is frequent and can alternate with diarrhea. The small intestine can demonstrate abnormal but nonspecific radiologic changes, including dilatation, diminished motility, and delayed transit.174
Dysmotility of the small intestine can play a significant role in the production of intestinal symptoms. Manometric findings included low-amplitude contractions in fasting and fed states, retrograde propagation of phase III waves, interruption of phase III, and increased incidence of tonic contractions.175 Spontaneous pneumoperitoneum, megacolon, and low-amplitude small intestinal contractions have been documented.
Histologically, smooth muscle cells in the small intestine show changes similar to those found in dystrophic skeletal muscle: They are swollen, partially destroyed, decreased in size, and replaced by fat. Using silver stain, degenerative changes of the myenteric plexus of the colon were found in a patient with megacolon,176 indicating that intestinal dysmotility may be caused by smooth muscle as well as enteric nerve dysfunction. In most patients, the predominant cause of dysmotility appears to be smooth muscle damage.
Duchenne’s Muscular Dystrophy
Duchenne’s muscular dystrophy is the most common of the inherited muscular dystrophies. A sex-linked disease, it affects one in 3500 boys; without respiratory support, death can occur before age 25 years. It is caused by mutations in the gene for dystrophin, a protein that helps stabilizes muscle cell membrane.177 In some cases, the mutations lead to an aberrant messenger RNA and result in a highly truncated dystrophin or no protein at all. Without functional dystrophin, muscle cell membranes leak, followed by muscle fiber necrosis and regeneration, and progressive replacement of muscle by fibrosis and fat. The muscles steadily waste away, and paralysis eventually occurs.177,178
Gastrointestinal symptoms usually are related to esophageal and gastric dysmotility, which are more severely affected than is the small intestine. Dysphagia is the predominant gastrointestinal symptom (36% in one series), followed by vomiting, diarrhea, and constipation.179 Gastric emptying delay and acute gastrointestinal dilatation characterize the clinical course.180 Orocecal transit time can be normal in asymptomatic subjects, although severe bowel dysmotility can occur.
Amyloidosis
Amyloidosis is a mixed group of disorders characterized by extracellular deposits of abnormal protein fibrils with a β-sheet fibrillar structure. The abnormal structure can be identified by x-ray diffraction studies and visualized with electron microscopy, but clinically, amyloid is identified by viewing the intestine with polarized light after staining it with Congo red.181
In patients with amyloidosis, diarrhea and constipation often are present for years and are followed or accompanied by a myriad of disparate problems including gastrointestinal bleeding, steatorrhea, protein loss, perforation, obstruction, intussusception, ischemia, pneumatosis, and pseudo-obstruction.182 A variety of mechanisms might explain the diarrhea: delayed motility and small bowel bacterial overgrowth,183 bile salt diarrhea,184 a sprue-like condition with amyloid deposition in the tips in the villi,185 and rapid orocecal transit.186
The severity of gastrointestinal dysmotility is correlated to the quantity and distribution of deposited amyloid. Amyloid is slowly deposited between muscle fibers, causing pressure atrophy of adjacent fibers so that eventually, the whole muscle layer is replaced by amyloid.182 Neuromuscular infiltration initially affects the intrinsic nervous system and results in a neuropathic process187 characterized by contractions that have normal amplitude but are uncoordinated.182,188 At a later stage, tissue wall infiltration results in a myopathic process with low-amplitude contractions that typically are associated with prolonged transit as in other systemic disorders, such as scleroderma. The vasculature often is involved, with amyloid deposition in the subintima or adventitia, and often involving the submucosa. When the vessel wall thickens, the lumen narrows and eventually obstructs, resulting in ischemia, infarction, and perforation.189 Mucosal architecture remains normal until massive deposits of amyloid destroy the mucosal structures.
There are several forms of amyloidosis: primary (AL), secondary (AA), hemodialysis-related (Aβ2MG), hereditary (ATTR), senile, and localized. AL is most common and has the most significant gastrointestinal involvement. AL amyloid reflects a generalized deposition of excess light chains associated with plasma cell dyscrasia, and 15% of patients with AL have multiple myeloma.190 Amyloid protein is deposited in the small intestine in all forms of amyloidosis.191
Secondary (AA) amyloidosis with acute-phase reactant serum amyloid A protein (A) is associated with infectious, inflammatory, or, rarely, neoplastic disorders.182,192 The incidence of amyloidosis in patients with rheumatoid arthritis ranges from 7% to 21%, the highest prevalence derived from an autopsy study.182 Other disorders associated with AA are Crohn’s disease, ankylosing spondylitis, Reiter’s syndrome, psoriasis, progressive systemic sclerosis, primary biliary cirrhosis, and systemic lupus erythematosus.
Inherited forms of amyloidogenic proteins are rare. The most common of this type is with variant transthyretin (TTR), which is produced by the liver,182,183 and the resulting amyloidosis (ATTR) is called familial amyloidotic polyneuropathy. Besides familial amyloidotic polyneuropathy, there are hereditary non-neuropathic systemic amyloidoses associated with mutations in genes for apolipoprotein AI, lysozyme, and fibrinogen α-chain.
Senile amyloidosis is found in 10% to 36% of patients older than 80 years and mainly involves the heart, but it also can be seen throughout the gastrointestinal tract. Amyloid has been observed in the subserosal veins of the large and small intestine in 41% to 44% of elderly patients.193
Chagas’ Disease
In nearly one third of patients, the late phase of infection with Trypanosoma cruzi leads to destruction of the submucosal and myenteric plexuses along the length of the gastrointestinal tract resulting in dilatation (see Chapter 109). Megacolon and megaesophagus are the most common presentations, although megaduodenum and megajejunum also can occur. Swallowing difficulties may be the first symptom of digestive disturbances and can lead to malnutrition. Some patients are entirely asymptomatic despite significant destruction of neurons. The early phase of gastric emptying of liquids is accelerated in patients who have Chagas’ disease and megaduodenum, suggesting that increased duodenal receptivity has a significant effect on the gastroduodenal transfer of liquids.194
Thyroid Disease
The earliest manifestation of a thyroid disorder might be gastrointestinal dysfunction. Hypothyroid patients often complain of constipation, and their gastric emptying time may be significantly delayed.195 Intestinal dysmotility (associated with change in the frequency of the slow wave oscillations of smooth muscle electrical potential) results from the altered thyroid state and has been considered to be the cause of symptoms. In hypothyroidism, small intestinal transit is significantly slowed.196 Many hypothyroid patients develop constipation, which may be the result of colonic dysmotility. In one patient, manometry revealed decreased amplitude of small intestinal contractions and an overall decreased motility index.197 With severe hypothyroidism (myxedema), paralytic ileus and intestinal pseudo-obstruction can occur. These abnormalities return to normal after the thyroid disorder is corrected.
Medications
Many drugs profoundly affect gastrointestinal motility.198 Although the colon is considered the principal target organ for drug-induced dysmotility, the small intestine often is similarly affected. Tricyclic antidepressants are noted for causing ileus. Phenothiazines and some antiparkinsonian drugs decrease colonic and small intestinal motility and can cause constipation, colonic pseudo-obstruction, and adynamic ileus. The anticholinergic agents atropine and scopolamine and related belladonna alkaloids decrease intestinal tone along with the amplitude and frequency of peristaltic contractions.
Opiate analgesics act on µ-opiate receptors throughout the intestinal tract to suppress motility; this effect is most pronounced in the colon. Loperamide, a predominantly peripheral opioid antagonist, causes chronic pseudo-obstruction by this antikinetic mechanism.198 Morphine enhances the amplitude of nonpropulsive small intestinal contractions and markedly decreases propulsive contractions. The duodenum and jejunum are more prone to these effects than is the ileum, and the overall effect is a delay in transit through the small intestine.
Calcium channel antagonists, particularly verapamil, by their direct stimulation of smooth muscle relaxation, cause constipation in up to 20% of patients and can also cause chronic pseudo-obstruction.199 Small intestinal transit time in subjects taking verapamil was unchanged from pretreatment values, although transit through the colon was slowed and this effect likely accounts for the constipation seen with this drug.
Celiac Disease
Intestinal pseudo-obstruction has been documented in association with celiac disease,200 but the mechanism is unclear. Dilated loops of small intestine with delayed transport of barium can be observed radiologically. In one patient who underwent exploratory laparotomy with full-thickness jejunal biopsy, the nerves and muscle cells appeared normal on both light and electron microscopy.200
Jejunal Diverticulosis
Diverticula can occur anywhere in the small intestine, but diverticulosis is most common in the jejunum. Like their counterparts in the colon, diverticula represent herniations through the mesenteric side of the bowel and usually are acquired. Jejunal diverticulosis is associated with many diseases, including scleroderma, celiac disease, MNGIE cytopathy, and Cronkhite-Canada syndrome.201 Patients can present with symptoms of small intestinal bacterial overgrowth, including diarrhea, steatorrhea, weight loss, and megaloblastic anemia. Local complications of diverticula also can occur, such as diverticulitis, bleeding, and perforation.
Acute and Chronic Irradiation
Ionizing radiation results in damage to all structures of the small intestine, including the mucosa, blood vessels, connective tissue, enteric nerves, and smooth muscle (see Chapter 39). Radiation damage to the intestine can be separated into acute and chronic injury.
Acute radiation injury is associated with symptoms of nausea, vomiting, abdominal pain, and diarrhea, which subside soon after exposure is discontinued. Small intestinal dysmotility can play a significant role in acute radiation enteropathy. Changes in small bowel absorption are considered the main cause of diarrhea. Delayed intestinal fasting and fed motility patterns can persist up to four weeks following the last exposure.202,203 One study has shown accelerated small intestinal transit in a group of patients undergoing abdominopelvic irradiation compared with their pretreatment values.204 More than 75% of the patients exhibited diarrhea during treatment.
Chronic radiation injury can cause gastrointestinal complications decades after the initial exposure. The neuropathic and myopathic injury results in intestinal dysmotility that leads to bacterial overgrowth, diarrhea, and malabsorption. Contrast studies often show dilated, thickened loops of bowel with air-fluid levels. Atrophy and fibrosis of smooth muscle fibers is a characteristic histologic finding, and proliferation of submucosal neurons of the myenteric plexus with extension into the circular muscle coat has been observed.205
Diffuse Lymphoid Infiltration
Diffuse lymphoid infiltration is a rare cause of pseudo-obstruction characterized by a diffuse infiltration of small lymphocytes mixed with mature polyclonal plasma cells that reflects a pseudolymphoma rather than a true neoplasm.179 It affects all the layers of the intestinal wall206 and is associated with extensive damage to the submucosal and myenteric nerve plexus.179
Idiopathic Myenteric Ganglionitis
Ganglionitis refers to an inflammatory neuropathy characterized by a dense lymphoplasmacytic infiltrate of the myenteric plexus134,205 and a marked reduction in the number of ganglia. Other cases have shown an eosinophil-predominant infiltrate, and neurons in the myenteric ganglia expressed the potent eosinophil chemoattractant IL-5.121,179 Ganglionitis is typically associated with paraneoplastic, infectious, or neurologic disorders, although some cases remain idiopathic. The diagnosis of enteric ganglionitis is supported by detecting circulating antineuronal antibodies against certain targets, such as Hu and Yo proteins, neurotransmitter receptors, and ion channels.134 A brief course of glucocorticoids or other immunosuppressive agent has been shown to be beneficial in some cases, thus reinforcing the value of establishing a correct diagnosis in the early stages of the disease process.134,207
Anorexia Nervosa and Bulimia
Delayed gastric emptying of solids and prolonged orocecal transit are well-established abnormalities in patients with anorexia or bulimia.208 Electrolyte abnormalities, such as hypokalemia, may be responsible in some cases and be related to nutritional deficiencies or concomitant diuretic abuse. Typically, the motility disorder is less significant than the underlying psychological and nutritional manifestations of the disease.
CLINICAL FEATURES
Dilatation of the small intestine or of isolated gastrointestinal segments is not a consistent finding in chronic pseudo-obstruction.124 During acute exacerbation, abdominal plain films can show air-fluid levels and dilatation of the small intestine. A chronically dilated, atonic intestine and bacterial overgrowth are more common in chronic pseudo-obstruction of intestinal myopathy and less likely in cases of neuropathic origin. Patients with intestinal dilatation often have malabsorption and steatorrhea, which causes additional weight loss. The prevalence and severity of recurrent obstructive episodes varies from patient to patient and from episode to episode in the same patient.
Extraintestinal features can occur, depending on the underlying disease. Megaduodenum, megacystis, and megaureter are commonly seen in type I FVM; these patients can have urinary retention and frequent infections. Type II FVM (MNGIE), discussed earlier, is defined clinically by two or more of the following extraintestinal conditions: progressive external ophthalmoplegia, ptosis, polyneuropathy, leukoencephalopathy, or a family history with no other known cause of pseudo-obstruction.209 MNGIE also is associated with hearing loss. Type III FVM is notable for marked dilatation of the entire gastrointestinal tract without extra-gastrointestinal features.
COMPLICATIONS
Total Parenteral Nutrition–Related Disorders
Patients with disabling gastrointestinal symptoms and irreversible intestinal failure often require total parenteral nutrition (TPN) (see Chapter 5). These patients are at risk for developing complications such as catheter-related sepsis, venous thrombosis, loss of venous access, and liver failure. Long-term TPN may be complicated by progressive cholestatic liver disease that can become irreversible The onset of hepatic dysfunction in a patient who has intestinal failure and is on chronic TPN is an indication for transplantation of intestine, in isolation or combined with a liver graft.210
Small Intestinal Bacterial Overgrowth
Common in chronic pseudo-obstruction, small bowel bacterial overgrowth syndrome is a condition associated with the proliferation of colon-type bacteria within the small intestine (see Chapter 102). The syndrome is characterized by steatorrhea, flatulence, abdominal discomfort, and bloating, with symptoms of macrocytic anemia, malabsorption of nutrients and vitamins, and weight loss; some patients have no symptoms.211 Direct quantitative cultures of jejunal contents is the diagnostic gold standard,212 although in the clinical setting noninvasive hydrogen breath tests are much more commonly used.211
Pneumatosis Cystoides Intestinalis
Pneumatosis cystoides intestinalis is a rare condition characterized by multiple gas-filled cysts in the submucosa or subserosa of the colon and, less often, the small intestine.213 The mechanism is thought to involve increased gas production by intestinal bacteria, thereby altering the partial pressure of nitrogen in the intestinal wall.214 Pneumatosis cystoides intestinalis is more common in patients with chronic obstructive pulmonary disease, but it occurs in cases of systemic sclerosis and has been described in patients with polymyositis, diabetes, duodenal and gastric ulceration, regional enteritis, and gastrointestinal malignancy. Intestinal peristaltic hypofunction associated with chronic pseudo-obstruction leads to raised intraluminal pressure, allowing the gas-producing bacteria to invade the intestinal mucosa through breaks in the mucosal integrity, forming pneumocysts. This condition can be diagnosed radiologically and endoscopically (Fig. 120-9). Subserous pneumocysts, in particular, are liable to rupture, releasing free gas into the peritoneal cavity (pneumoperitoneum), making it important to distinguish this condition from bowel perforation. Pneumoperitoneum from rupture of pneumatosis cysts does not per se mandate operation, and is not complicated by peritonitis.
NATURAL HISTORY
Chronic intestinal pseudo-obstruction is a severely debilitating and progressive disorder. Familial cases have their onset at birth or typically in the first few years of life.215 Childhood onset is characterized by a particularly severe course with high mortality rates.215,216 The first occlusive episode in adults is often preceded by years of nonspecific progressive digestive complaints. The diagnosis is made a median of 8.8 years after the initial onset of symptoms, and 88% of adults undergo a mean of 2.96 unnecessary operations217 before the diagnosis is established.113 The principal causes of death relate to complications of surgery, parenteral nutrition, transplantation, and septic shock of gastrointestinal origin. Intestinal myopathy and intestinal hypomotility predict poor outcome. MNGIE has a particularly poor prognosis, with death occurring at about age 40 years.113
DIAGNOSIS
Radiologic Studies
Plain abdominal films are very useful in patients who complain of abdominal distention and bloating because they might show gaseous distention of the areas affected by severe dysmotility; dilatation is greater in cases of pseudo-obstruction related to myopathy than when the cause is neuropathic dysmotility. Enteroclysis can be useful for detecting lesions in the small intestine and ruling out mechanical obstruction. Entero-CT scanning allows simultaneous internal and external views of the intestinal wall. Abdominal CT and MR scans are important in investigating possible causes of bowel compression, and MR angiography can noninvasively identify congenital or acquired vascular abnormalities. Excretory urograms should be performed in patients with urinary symptoms.113
Radiopaque polyethylene markers that move with the colonic contents are robust measures of intestinal transit and are used to evaluate whole-gut transit. Segmental colonic transit can be measured with daily abdominal plain films after a single dose of radiopaque markers. Metcalf and colleagues218 simplified the method so that only one film with high kV technique is necessary and radiation exposure is minimized. By this method, radiopaque markers are taken in fixed numbers (24 per day), at the same time (arbitrarily, 9:00 a.m.) each day for three days. On the fourth day, again at the same time, plain film is taken. The method works on the assumption that a 24-hour sampling interval approximates continuous observation. Rapid transit can cause all the markers to be lost in the feces before filming on the fourth day; conversely, in slow transit, all 72 markers may be present on the single plain film; a film on day seven then gives more information.
Laboratory Tests
A complete blood count can reveal anemia and macrocytosis as a result of malnutrition and bacterial overgrowth (see Chapters 101 and 102). Blood chemistries also reflect malnutrition and malabsorption. Diabetic patients have hyperglycemia, and hypoparathyroid patients can have hypocalcemia. Patients with connective tissue disease can have a positive antinuclear antibody or SCL-70. Patients with thyroid disease have changes in serum T3, T4, and TSH levels. Muscular dystrophy or mitochondrial cytopathy patients can have elevated creatine phosphokinase (CPK) and isoenzymes. Hemagglutination and complement fixation for Chagas’ disease may be positive in patients with a history of living in Central or South America.
Surgery
Full-thickness biopsies should be obtained from dilated and nondilated areas of the intestinal tract in all patients with suspected chronic pseudo-obstruction who undergo surgery for unexplained occlusive episodes; biopsy should be considered in patients with acute symptoms. Biopsies should be processed by traditional staining and by specialized immunohistochemistry techniques in dedicated laboratories with a specific interest in this area.125
Manometry
Myopathic Pattern
In patients with myopathy, manometry demonstrates low-amplitude contractions in the affected segment; this pattern generally is found during both fasting and fed periods. During fasting, the MMC usually is present but is diminished in amplitude. The fed pattern also is associated with reduced frequency of contractions. The poorly developed intestinal fed pattern is in part a result of reduced gastric emptying. Weston and associates showed that in myopathic disorders, antral amplitudes are usually less than 40 mm Hg and duodenal amplitudes are less than 10 mm Hg.152
Neuropathic Pattern
Neurologic disorders tend to produce uncoordinated bursts of intense contractions of variable duration but normal amplitude. The MMC often is absent or abnormal in these patients. An abnormal rate of migration, and retrograde propagation of the activity front (phase III), also may be noted. Activity fronts can appear to be normal proximally and then arrest or disappear in the more distal segments of the small intestine. In neuropathic disorders, the normal fed pattern might not replace the fasted pattern, MMC-like activity persists postprandially (normally, the MMC activity should be abolished for one hour per 200 kcal ingested), and the frequency of antral contractions in the first hour is typically less than one per minute, in contrast to the average two (range one to three) per minute in healthy controls.155 These abnormalities reflect dysregulation by the enteric or extrinsic nervous system.
Mechanical Obstruction
The manometric pattern of mechanical obstruction (as opposed to pseudo-obstruction) is characterized by giant propagated or nonpropagated contractions lasting at least 10 seconds or clustered contractions lasting one to five seconds followed by more than one minute of absent motor activity.124 Manometry is not the usual way to establish the diagnosis of mechanical obstruction, but it should alert the physician to the possibility of mechanical obstruction and mandate careful small bowel barium examination, such as by enteroclysis.
TREATMENT
Pain
Long-term narcotic use should be discouraged because patients can become addicted to narcotics, and narcotics can further disturb gastrointestinal motility. Tramadol, gabapentin, and pregabalin sometimes are prescribed for such pain, but these too can retard intestinal transit. Unfortunately, there are conflicting data on transit effects of these medications in otherwise healthy people and virtually no such data in patients with intestinal pseudo-obstruction.219–221
Nutrition Management
In patients with gastrointestinal dysmotility, abdominal pain, bloating, nausea, and vomiting often are related to eating. Symptoms usually can be minimized by manipulating the amount, constituents, and frequency of meals. Patients need sufficient calories without overloading the inefficient bowel: a useful general rule is 25 cal/kg of the patient’s ideal body weight per day taken in small, equal-size feedings four to six times daily. Multivitamins and salt supplementation generally is recommended. Because a liquid meal empties faster from the stomach and probably progresses more readily through the small intestine than a solid meal does, the liquid meal or perhaps an emulsified solid meal may be better tolerated than a solid meal. Supplemental formulas contain the daily vitamin and nutrient requirements, and many are lactose free. There is no advantage to using elemental formulas. Hypercaloric solutions are available, although generally formulas that have a lower caloric content (1 kcal/mL) are better tolerated than those with greater caloric density. Parenteral nutrition may be required to meet caloric and nutritional needs, but oral intake should continue as tolerated. TPN-related complications including catheter-related sepsis or thrombosis, liver insufficiency, pancreatitis, and glomerulonephritis113 significantly increase the morbidity associated with chronic intestinal pseudo-obstruction.
Pharmacotherapy
The aim of treatment is to reduce digestive symptoms and lower the risk of complications. Disordered motility of the small intestine leads to bacterial overgrowth and malnutrition. Poorly absorbed antibiotics, such as rifaximin, are the preferred treatment for bacterial overgrowth. Cycled treatments using metronidazole, ciprofloxacin, and doxycycline can limit resistance. Antiemetics and antispasmodic agents should be tried, although their benefit may be short-lived. Improving intestinal motor function is another means of reducing symptoms. Two controlled trials showed cisapride can improve symptoms and enhance emptying in patients with chronic intestinal dysmotility.222–224 The positive effect of cisapride is not as apparent in patients with vagal dysfunction, such as in diabetes and following vagotomy.224
Other prokinetic agents, including erythromycin, metoclopramide, domperidone, neostigmine, and bethanechol, have been used to treat chronic pseudo-obstruction, but results are anecdotal and high quality studies are lacking. Domperidone is not FDA approved and is unavailable in the United States. In a small, short-term study, the somatostatin analog octreotide stimulated intestinal motility, possibly reduced bacterial overgrowth, and improved abdominal symptoms in patients with scleroderma.152 Other open-labeled studies confirmed the long-term effectiveness of octreotide with erythromycin in the treatment of chronic pseudo-obstruction, but some data show that octreotide retards gastric225 and small bowel transit in health, and many use the drug (50 µg dose) to induce MMC-like contractions at least two hours after the last meal of the day to sweep residue out of the small intestine toward the colon and prevent bacterial overgrowth.226 Oral pyridostigmine (60 mg/5 mL, to 2 teaspoons one to three times daily with gradual titration as needed 10-15 minutes before meals) may be effective in reducing symptoms of bloating and constipation, but clinical trials with this agent are needed.
Treatment of inflammatory neuropathies is centered on the use of immunosuppressive therapies. The diagnosis should be suspected because of the presence of circulating antibodies and proved through specialized tissue analysis, if available.124 When a diagnosis of an enteric ganglionitis or inflammatory neuropathy has been established, glucocorticoids, such as prednisolone (60-100 mg/day), methylprednisolone (up to 600 mg/day), or beclomethasone (200 µg three times daily), alone or in combination with other immunosuppressive treatments, such as azathioprine or cyclophosphamide, have been associated with significant clinical improvement.134
Surgical Therapy
In carefully selected patients, such as those with megaduodenum of the small intestine, resection or bypass of dysfunctional segments can have a good prognosis.227 Venting gastrostomy and enterostomy may be used to reduce abdominal distention and vomiting, which can have a positive effect on conveying intestinal contents through the gastrointestinal tract.113 Because chronic intestinal pseudo-obstruction tends to be a progressive disorder, surgery might provide only temporary benefit. Unnecessary surgery should be avoided because it can create adhesions and future additional difficulties.
Small intestine and multiorgan transplantation are gaining recognition as standard treatment for patients with irreversible intestinal failure in whom TPN fails or those who have high morbidity and poor disease-related quality of life despite optimal parenteral nutrition.228 Isolated intestinal transplant is technically less complex than transplantation of combined or multiorgan grafts, and it is preferred in cases of liver disease associated with intestinal failure because the liver failure often reverses if transplantation is successful.229
Immunosuppressive management, such as tacrolimus, represents a landmark in the treatment of patients undergoing transplantation for intestinal failure. Advances in immunosuppressive protocols, including induction therapy with monoclonal antibodies (daclizumab, alemtuzumab, or thymoglobulin), has lowered immunosuppressive requirements and reduced the risk of renal failure and sepsis. Advances in surgical techniques and graft monitoring via graft ileoendoscopy and intestinal biopsy have led to improved graft and patient survival rates.229 In one series of isolated intestinal transplants in adult patients, the survival rates were similar to those for other solid organ transplantations.229 Three year patient survival rates of 70% for isolated intestinal transplantations and 41% for multivisceral transplantations are reported, with mortality rates of 32.5% from sepsis (63%) or rejection.229
MEGACOLON AND MEGARECTUM
In congenital megacolon (Hirschsprung’s disease), a congenital absence of the intramural neural plexus that mediates relaxation (aganglionosis) causes narrowing (most often of the rectum) of a variable distance proximally and results in functional obstruction (see Chapter 96). Short segment involvement (rectum or rectosigmoid) is most common, although an ultrashort segment of aganglionosis involving only the internal anal sphincter or long segment involvement (less than 20% of patients) can occur. Physiologic testing may be necessary to define ultrashort segment as morphologic, and radiologic confirmation of the diagnosis may be difficult. Hirschsprung’s disease is detected more commonly in children than in adults, but ultrashort segment disease is usually not diagnosed until adulthood.230
Antonucci A, Fronzoni L, Cogliandro L, et al. Chronic intestinal pseudo-obstruction. World J Gastroenterol. 2008;14(19):2953-61. (Ref 113.)
Apfel CC, Korttila K, Abdalla M, et al. A factorial trial of six interventions for the prevention of postoperative nausea and vomiting. N Engl J Med. 2004;350:2441-51. (Ref 77.)
Bauer AJ, Boeckxstaens GE. Mechanisms of postoperative ileus. Neurogastroenterol Motil. 2004;16(Suppl 2):54-60. (Ref 17.)
Bauer TM, Schwacha H, Steinbruckner B, et al. Diagnosis of small intestinal bacterial overgrowth in patients with cirrhosis of the liver: poor performance of the glucose breath hydrogen test. J Hepatol. 2000;33:382-6. (Ref 212.)
De Giorgio R, Guerrini S, Barbara G, et al. Inflammatory neuropathies of the enteric nervous system. Gastroenterology. 2004;126:1872-83. (Ref 134.)
Ebert EC, Nagar M. Gastrointestinal manifestations of amyloidosis. Am J Gastroenterol. 2008;103:776-87. (Ref 182.)
Kreiss C, Toegel S, Bauer AJ. α2-Adrenergic regulation of NO production alters postoperative intestinal smooth muscle dysfunction in rodents. Am J Physiol Gastrointest Liver Physiol. 2004;287:G658-66. (Ref 40.)
Luckey A, Livingston E, Tache Y. Mechanisms and treatment of postoperative ileus. Arch Surg. 2003;138:206-14. (Ref 19.)
Pironi L, Spinucci G, Paganelli F, et al. Italian guidelines for intestinal transplantation: potential candidates among the adult patients managed by a medical referral center for chronic intestinal failure. Transplant Proc. 2004;36:659-61. (Ref 228.)
Vanek VW, Al-Salti M. Acute pseudo-obstruction of the colon (Ogilvie’s syndrome). An analysis of 400 cases. Dis Colon Rectum. 1986;29:203-10. (Ref 3.)
1. Prasad M, Matthews JB. Deflating postoperative ileus. Gastroenterology. 1999;117(2):489-92.
2. Moons V, Coremans G, Tack J. An update on acute colonic pseudo-obstruction (Ogilvie’s syndrome). Acta Gastroenterol Belg. 2003;66(2):150-3.
3. Vanek VW, Al-Salti M. Acute pseudo-obstruction of the colon (Ogilvie’s syndrome). An analysis of 400 cases. Dis Colon Rectum. 1986;29(3):203-10.
4. Kadesky K, Purdue GF, Hunt JL. Acute pseudo-obstruction in critically ill patients with burns. J Burn Care Rehab. 1995;16(2 Pt 1):132-5.
5. Saunders MD. Acute colonic pseudo-obstruction. Gastrointest Endos Clin North Am. 2007;17(2):341-60.
6. Lyford G, Foxx-Orenstein AE. Chronic intestinal pseudoobstruction. Curr Treat Gastroenterol. 2004;7(4):317-25.
7. Kashyap P, Farrugia G. Enteric autoantibodies and gut motility disorders. Gastroenterol Clin North Am. 2008;37(2):397-410.
8. Newton WT. Radical enterectomy for hereditary megaduodenum. Arch Surg. 1968;96(4):549-53.
9. Byrne WJ, Cipel L, Euler AR, et al. Chronic idiopathic intestinal pseudo-obstruction syndrome in children—clinical characteristics and prognosis. J Pediatr. 1977;90(4):585-9.
10. Wood JD, Grundy D. Little brain—big brain V. Neurogastroenterol Motil. 1998;10(5):377-85.
11. Thuneberg L. Interstitial cells of Cajal: intestinal pacemaker cells? Adv Anat Embryol Cell Biol. 1982;71:1-130.
12. Furness JB, Kunze WA, Clerc N. Nutrient tasting and signaling mechanisms in the gut. II. The intestine as a sensory organ: neural, endocrine, and immune responses. Am J Physiol. 1999;277(5 Pt 1):G922-8.
13. Grider JR, Foxx-Orenstein AE, Jin JG. 5-Hydroxytryptamine4 receptor agonists initiate the peristaltic reflex in human, rat, and guinea pig intestine. Gastroenterology. 1998;115(2):370-80.
14. Pomeranz HD, Rothman TP, Gershon MD. Colonization of the post-umbilical bowel by cells derived from the sacral neural crest: direct tracing of cell migration using an intercalating probe and a replication-deficient retrovirus. Development. 1991;111(3):647-55.
15. Fu CG, Muto T, Masaki T, Nagawa H. Zonal adult Hirschsprung’s disease. Gut. 1996;39(5):765-7.
16. Miedema BW, Johnson JO. Methods for decreasing postoperative gut dysmotility. Lancet Oncol. 2003;4(6):365-72.
17. Bauer AJ, Boeckxstaens GE. Mechanisms of postoperative ileus. Neurogastroenterol Motil. 2004;16(Suppl 2):54-60.
18. Mattei P, Rombeau JL. Review of the pathophysiology and management of postoperative ileus. World J Surg. 2006;30(8):1382-91.
19. Luckey A, Livingston E, Tache Y. Mechanisms and treatment of postoperative ileus. Arch Surg. 2003;138(2):206-14.
20. Livingston EH, Passaro EPJr. Postoperative ileus. Dig Dis Sci. 1990;35(1):121-32.
21. Holte K, Kehlet H. Postoperative ileus: a preventable event. Br J Surg. 2000;87(11):1480-93.
22. Holte K, Kehlet H. Postoperative ileus: progress towards effective management. Drugs. 2002;62(18):2603-15.
23. Artinyan A, Nunoo-Mensah JW, Balasubramaniam S, et al. Prolonged postoperative ileus-definition, risk factors, and predictors after surgery. World J Surg. 2008;32(7):1495-500.
24. Holzer P, Lippe IT, Amann R. Participation of capsaicin-sensitive afferent neurons in gastric motor inhibition caused by laparotomy and intraperitoneal acid. Neuroscience. 1992;48(3):715-22.
25. Espat NJ, Cheng G, Kelley MC, et al. Vasoactive intestinal peptide and substance P receptor antagonists improve postoperative ileus. J Surg Res. 1995;58(6):719-23.
26. De Winter BY, Robberecht P, Boeckxstaens GE, et al. Role of VIP1/PACAP receptors in postoperative ileus in rats. Br J Pharmacol. 1998;124(6):1181-6.
27. Bonaz B, Tache Y. Corticotropin-releasing factor and systemic capsaicin-sensitive afferents are involved in abdominal surgery-induced Fos expression in the paraventricular nucleus of the hypothalamus. Brain Res. 1997;748(1-2):12-20.
28. Aikawa S, Ishii M, Yanagisawa M, et al. Effect of neuropeptide B on feeding behavior is influenced by endogenous corticotropin-releasing factor activities. Regul Pept. 2008;151(1-3):147-52.
29. Tache Y, Martinez V, Million M, Wang L. Stress and the gastrointestinal tract III. Stress-related alterations of gut motor function: role of brain corticotropin-releasing factor receptors. Am J Physiol Gastrointest Liver Physiol. 2001;280(2):G173-7.
30. Tache Y, Monnikes H, Bonaz B, Rivier J. Role of CRF in stress-related alterations of gastric and colonic motor function. Ann N Y Acad Sci. 1993;697:233-43.
31. Greenwood-Van Meerveld B. Emerging drugs for postoperative ileus. Expert Opin Emerg Drugs. 2007;12(4):619-26.
32. Evangelista S. Role of sensory neurons in restitution and healing of gastric ulcers. Curr Pharma Des. 2006;12(23):2977-84.
33. Kalff JC, Turler A, Schwarz NT, et al. Intra-abdominal activation of a local inflammatory response within the human muscularis externa during laparotomy. Ann Surg. 2003;237(3):301-15.
34. Schwarz NT, Beer-Stolz D, Simmons RL, Bauer AJ. Pathogenesis of paralytic ileus: intestinal manipulation opens a transient pathway between the intestinal lumen and the leukocytic infiltrate of the jejunal muscularis. Ann Surg. 2002;235(1):31-40.
35. De Jonge WJ, The FO, van der Zanden EP, et al. Inflammation and gut motility: neural control of intestinal immune cell activation. J Pediatr Gastroenterol Nutri. 2005;41(Suppl 1):S10-1.
36. The FO, de Jonge WJ, Bennink RJ, et al. The ICAM-1 antisense oligonucleotide ISIS-3082 prevents the development of postoperative ileus in mice. Br J Pharmacol. 2005;146(2):252-8.
37. Turler A, Moore BA, Pezzone MA, et al. Colonic postoperative inflammatory ileus in the rat. Ann Surg. 2002;236(1):56-66.
38. Blandizzi C, Fornai M, Colucci R, et al. Altered prejunctional modulation of intestinal cholinergic and noradrenergic pathways by alpha2-adrenoceptors in the presence of experimental colitis. Br J Pharmacol. 2003;139(2):309-20.
39. Blandizzi C. Enteric alpha-2 adrenoceptors: pathophysiological implications in functional and inflammatory bowel disorders. Neurochem Int. 2007;51(5):282-8.
40. Kreiss C, Toegel S, Bauer AJ. α2-Adrenergic regulation of NO production alters postoperative intestinal smooth muscle dysfunction in rodents. Am J Physiol Gastrointest Liver Physiol. 2004;287(3):G658-66.
41. Lang K, Boldt J, Suttner S, Haisch G. Colloids versus crystalloids and tissue oxygen tension in patients undergoing major abdominal surgery. Anesth Analg. 2001;93(2):405-9.
42. Lobo DN, Bostock KA, Neal KR, et al. Effect of salt and water balance on recovery of gastrointestinal function after elective colonic resection: a randomised controlled trial. Lancet. 2002;359(9320):1812-18.
43. Bundgaard-Nielsen M, Holte K, Secher NH, Kehlet H. Monitoring of peri-operative fluid administration by individualized goal-directed therapy. Acta Anaesthesiol Scand. 2007;51(3):331-40.
44. Ogilvy AJ, Smith G. The gastrointestinal tract after anaesthesia. Eur J Anaesthesiol Suppl. 1995;10:35-42.
45. Holte K, Kehlet H. Epidural anaesthesia and analgesia—effects on surgical stress responses and implications for postoperative nutrition. Clin Nutr. 2002;21(3):199-206.
46. Liu S, Carpenter RL, Neal JM. Epidural anesthesia and analgesia. Their role in postoperative outcome. Anesthesiology. 1995;82(6):1474-506.
47. Steinbrook RA. Epidural anesthesia and gastrointestinal motility. Anesth Analg. 1998;86(4):837-44.
48. Neudecker J, Schwenk W, Junghans T, et al. Randomized controlled trial to examine the influence of thoracic epidural analgesia on postoperative ileus after laparoscopic sigmoid resection. Br J Surg. 1999;86(10):1292-5.
49. Delaney CP. Clinical perspective on postoperative ileus and the effect of opiates. Neurogastroenterol Motil. 2004;16(Suppl 2):61-6.
50. Yoshida S, Ohta J, Yamasaki K, et al. Effect of surgical stress on endogenous morphine and cytokine levels in the plasma after laparoscopic or open cholecystectomy. Surg Endosc. 2000;14(2):137-40.
51. Kurz A, Sessler DI. Opioid-induced bowel dysfunction: pathophysiology and potential new therapies. Drugs. 2003;63(7):649-71.
52. Manara L, Bianchetti A. The central and peripheral influences of opioids on gastrointestinal propulsion. Ann Rev Pharmacol Toxicol. 1985;25:249-73.
53. Foxx-Orenstein AE, Jin JG, Grider JR. 5-HT4 receptor agonists and delta-opioid receptor antagonists act synergistically to stimulate colonic propulsion. Am J Physiol. 1998;275(5 Pt 1):G979-83.
54. Frantzides CT, Condon RE, Schulte WJ, Cowles V. Effects of morphine on colonic myoelectric and motor activity in subhuman primates. Am J Physiol. 1990;258(2 Pt 1):G247-52.
55. Borody TJ, Quigley EM, Phillips SF, et al. Effects of morphine and atropine on motility and transit in the human ileum. Gastroenterology. 1985;89(3):562-70.
56. Mangesi L, Hofmeyr G. Early compared with delayed oral fluids and food after caesarean section. Cochrane Database Syst Rev 2002(3):CD003516.
57. Johnson Casto C, Krammer J, Drake J. Postoperative feeding: a clinical review. Obstet Gynecol Surv. 2000;55(9):571-3.
58. Cheatham ML, Chapman WC, Key SP, Sawyers JL. A meta-analysis of selective versus routine nasogastric decompression after elective laparotomy. Ann Surg. 1995;221(5):469-76.
59. Nelson R, Tse B, Edwards S. Systematic review of prophylactic nasogastric decompression after abdominal operations. Br J Surg. 2005;92(6):673-80.
60. Waldhausen JH, Schirmer BD. The effect of ambulation on recovery from postoperative ileus. Ann Surg. 1990;212(6):671-7.
61. Lacy AM, Garcia-Valdecasas JC, Delgado S, et al. Postoperative complications of laparoscopic-assisted colectomy. Surg Endosc. 1997;11(2):119-22.
62. Leung KL, Lai PB, Ho RL, et al. Systemic cytokine response after laparoscopic-assisted resection of rectosigmoid carcinoma: A prospective randomized trial. Ann Surg. 2000;231(4):506-11.
63. Bohm B, Milsom JW, Fazio VW. Postoperative intestinal motility following conventional and laparoscopic intestinal surgery. Arch Surg. 1995;130(4):415-19.
64. Davies W, Kollmorgen CF, Tu QM, et al. Laparoscopic colectomy shortens postoperative ileus in a canine model. Surgery. 1997;121(5):550-5.
65. Brodner G, van Aken H, Kehlet H. Influence of thoracic epidural anaesthesia on the metabolic stress response. Best Pract Res Clin Anaesth. 1999;13(1):23-29.
66. Bredtmann RD, Herden HN, Teichmann W, et al. Epidural analgesia in colonic surgery: results of a randomized prospective study. Br J Surg. 1990;77(6):638-42.
67. Asantila R, Eklund P, Rosenberg PH. Continuous epidural infusion of bupivacaine and morphine for postoperative analgesia after hysterectomy. Acta Anaesth Scand. 1991;35(6):513-17.
68. Jorgensen H, Fomsgaard JS, Dirks J, et al. Effect of peri- and postoperative epidural anaesthesia on pain and gastrointestinal function after abdominal hysterectomy. Br J Anaesth. 2001;87(4):577-83.
69. Kehlet H. Fast-track colorectal surgery. Lancet. 2008;371(9615):791-3.
70. Werawatganon T, Charuluxanun S. Patient controlled intravenous opioid analgesia versus continuous epidural analgesia for pain after intra-abdominal surgery. Cochrane Database Syst Rev 2005(1):CD004088.
71. Kehlet H, Dahl JB. Anaesthesia, surgery, and challenges in postoperative recovery. Lancet. 2003;362(9399):1921-8.
72. White PF, Kehlet H, Neal JM, et al. The role of the anesthesiologist in fast-track surgery: from multimodal analgesia to perioperative medical care. Anesth Analg. 2007;104(6):1380-96.
73. Holte K, Kehlet H. Fluid therapy and surgical outcomes in elective surgery: a need for reassessment in fast-track surgery. J Am Coll Surg. 2006;202(6):971-89.
74. Jans O, Tollund C, Bundgaard-Nielsen M, et al. Goal-directed fluid therapy: stroke volume optimisation and cardiac dimensions in supine healthy humans. Acta Anaesth Scand. 2008;52(4):536-40.
75. Gan TJ, Meyer TA, Apfel CC, et al. Society for Ambulatory Anesthesia guidelines for the management of postoperative nausea and vomiting. Anesth Analg. 2007;105(6):1615-28.
76. Sinclair DR, Chung F, Mezei G. Can postoperative nausea and vomiting be predicted? Anesthesiology. 1999;91(1):109-18.
77. Apfel CC, Korttila K, Abdalla M, et al. A factorial trial of six interventions for the prevention of postoperative nausea and vomiting. N Engl J Med. 2004;350(24):2441-51.
78. Chan MK, Law WL. Use of chewing gum in reducing postoperative ileus after elective colorectal resection: a systematic review. Dis Colon Rectum. 2007;50(12):2149-57.
79. Vasquez W, Hernandez A, Garcia-Sabrido J. Is gum chewing useful for ileus after elective colorectal surgery? A systematic review and meta-analysis of randomized clinical trials. J Gastrointest Surg. 2009;13(4):649-56.
80. Kehlet H. Postoperative ileus—an update on preventive techniques. Nat Clin Pract Gastroenterol Hepatol. 2008;5(10):552-558.
81. Lewis S, Andersen H, Thomas S. Early enteral nutrition within 24 h of intestinal surgery versus later commencement of feeding: a systematic review and meta-analysis. J Gastrointest Surg J Gastrointest Surg. 2009;13(3):569-75.
82. Carr CS, Ling KD, Boulos P, Singer M. Randomised trial of safety and efficacy of immediate postoperative enteral feeding in patients undergoing gastrointestinal resection. BMJ. 1996;312(7035):869-71.
83. Andersen HK, Lewis SJ, Thomas S. Early enteral nutrition within 24h of colorectal surgery versus later commencement of feeding for postoperative complications. Cochrane Database Syst Rev 2006(4):CD004080.
84. Spindler JS, Mehlisch D, Brown CR. Intramuscular ketorolac and morphine in the treatment of moderate to severe pain after major surgery. Pharmacotherapy. 1990;10(6(Pt 2):51S-8S.
85. Schlachta CM, Burpee SE, Fernandez C, et al. Optimizing recovery after laparoscopic colon surgery (ORAL-CS): effect of intravenous ketorolac on length of hospital stay. Surg Endosc. 2007;21(12):2212-9.
86. Kaba A, Laurent SR, Detroz BJ, et al. Intravenous lidocaine infusion facilitates acute rehabilitation after laparoscopic colectomy. Anesthesiology. 2007;106(1):11-18.
87. Kehlet H. Postoperative opioid sparing to hasten recovery: what are the issues? Anesthesiology. 2005;102(6):1083-5.
88. Zutshi M, Delaney CP, Senagore AJ, et al. Randomized controlled trial comparing the controlled rehabilitation with early ambulation and diet pathway versus the controlled rehabilitation with early ambulation and diet with preemptive epidural anesthesia/analgesia after laparotomy and intestinal resection. Am J Surg. 2005;189(3):268-72.
89. Delaney CP, Wolff BG, Viscusi ER, et al. Alvimopan, for postoperative ileus following bowel resection: a pooled analysis of phase III studies. Ann Surg. 2007;245(3):355-63.
90. Wolff BG, Viscusi ER, Delaney CP, et al. Patterns of gastrointestinal recovery after bowel resection and total abdominal hysterectomy: pooled results from the placebo arms of alvimopan phase III North American clinical trials. J Am Coll Surg. 2007;205(1):43-51.
91. Wolff BG, Weese JL, Ludwig KA, et al. Postoperative ileus-related morbidity profile in patients treated with alvimopan after bowel resection. J Am Coll Surg. 2007;204(4):609-16.
92. Traut U, Brugger L, Kunz R, et al. Systemic prokinetic pharmacologic treatment for postoperative adynamic ileus following abdominal surgery in adults. Cochrane Database Syst Rev 2008(1):CD004930.
93. Thomas J, Karver S, Cooney GA, et al. Methylnaltrexone for opioid-induced constipation in advanced illness. N Engl J Med. 2008;358(22):2332-43.
94. Brown TA, McDonald J, Williard W. A prospective, randomized, double-blinded, placebo-controlled trial of cisapride after colorectal surgery. Am J Surg. 1999;177(5):399-401.
95. Wiriyakosol S, Kongdan Y, Euanorasetr C, et al. Randomized controlled trial of bisacodyl suppository versus placebo for postoperative ileus after elective colectomy for colon cancer. Asian J Surg. 2007;30(3):167-72.
96. Fanning J, Yu-Brekke S. Prospective trial of aggressive postoperative bowel stimulation following radical hysterectomy. Gynecol Oncol. 1999;73(3):412-14.
97. Hansen CT, Sorensen M, Moller C, et al. Effect of laxatives on gastrointestinal functional recovery in fast-track hysterectomy: a double-blind, placebo-controlled randomized study. Am J Obstet Gynecol. 2007;196(4):311.e1-7.
98. Zargar-Shoshtari K, Connolly AB, Israel LH, Hill AG. Fast-track surgery may reduce complications following major colonic surgery. Dis Colon Rectum. 2008;51(11):1633-40.
99. Wind J, Polle SW, Fung Kon Jin PHP, et al. Systematic review of enhanced recovery programmes in colonic surgery. Br J Surg. 2006;93(7):800-9.
100. Khoo CK, Vickery CJ, Forsyth N, et al. A prospective randomized controlled trial of multimodal perioperative management protocol in patients undergoing elective colorectal resection for cancer. Ann Surg. 2007;245(6):867-72.
101. Ogilvie W. Large-intestine colic due to sympathetic deprivation. Br Med J. 1948;2:671-673.
102. De Giorgio R, Barbara G, Stanghellini V, et al. Review article: the pharmacological treatment of acute colonic pseudo-obstruction. Aliment Pharmacol Ther. 2001;15(11):1717-27.
103. Saunders MD, Kimmey MB. Systematic review: acute colonic pseudo-obstruction. Aliment Pharmacol Ther. 2005;22(10):917-25.
104. Geller A, Petersen BT, Gostout CJ. Endoscopic decompression for acute colonic pseudo-obstruction. Gastrointest Endosc. 1996;44(2):144-50.
105. Lowman R, Davis L. An evaluation of cecal size in impending perforation of the colon. Surg Gynecol Obstet. 1956;16:711-18.
106. Guenaga KF, Matos D, Castro AA, et al. Mechanical bowel preparation for elective colorectal surgery. [update in Cochrane Database Syst Rev. 2005; (1):CD001544; PMID: 15674882]. Cochrane Database Syst Rev 2003(2):CD001544.
107. Fukatsu K, Zarzaur BL, Johnson CD, et al. Enteral nutrition prevents remote organ injury and death after a gut ischemic insult. Ann Surg. 2001;233(5):660-8.
108. De Giorgio R, Stanghellini V, Barbara G, et al. Prokinetics in the treatment of acute intestinal pseudo-obstruction. Idrugs. 2004;7(2):160-5.
109. Ponec RJ, Saunders MD, Kimmey MB. Neostigmine for the treatment of acute colonic pseudo-obstruction. N Engl J Med. 1999;341(3):137-41.
110. De Giorgio R, Knowles CH. Acute colon pseudo-obstruction. Br J Surg. 2009;96:223-39.
111. Saunders MD. Acute colonic pseudo-obstruction. Best Pract Res Clin Gastroenterol. 2007;21(4):671-87.
112. Ramage JIJr, Baron TH. Percutaneous endoscopic cecostomy: a case series. Gastrointest Endosc. 2003;57(6):752-5.
113. Antonucci A, Fronzoni L, Cogliandro L, et al. Chronic intestinal pseudo-obstruction. World J Gastroenterol. 2008;14(19):2953-61.
114. Krishnamurthy S, Schuffler MD. Pathology of neuromuscular disorders of the small intestine and colon. Gastroenterology. 1987;93(3):610-39.
115. De Giorgio R, Sarnelli G, Corinaldesi R, Stanghellini V. Advances in our understanding of the pathology of chronic intestinal pseudo-obstruction. Gut. 2004;53(11):1549-52.
116. Deglincerti A, De Giorgio R, Cefle K, et al. A novel locus for syndromic chronic idiopathic intestinal pseudo-obstruction maps to chromosome 8q23-q24. Eur J Hum Genet. 2007;15(8):889-97.
117. De Giorgio R, Guerrini S, Barbara G, et al. New insights into human enteric neuropathies. Neurogastroenterol Motil. 2004;16(Suppl 1):143-7.
118. Talamonti L, Li Q, Beyak M, et al. Sensory-motor abnormalities in severe gut dysmotility: role of anti-HuD neuron antibody (A-HuD). Neurogastroenterol Motil. 2006;18(8):669.
119. De Giorgio R, Bovara M, Barbara G, et al. Anti-HuD-induced neuronal apoptosis underlying paraneoplastic gut dysmotility. Gastroenterology. 2003;125(1):70-9.
120. Debinski HS, Kamm MA, Talbot IC, et al. DNA viruses in the pathogenesis of sporadic chronic idiopathic intestinal pseudo-obstruction. Gut. 1997;41(1):100-6.
121. Schappi MG, Smith VV, Milla PJ, Lindley KJ. Eosinophilic myenteric ganglionitis is associated with functional intestinal obstruction. Gut. 2003;52(5):752-5.
122. Accarino A, Colucci R, Barbara G, et al. Mast cell neuromuscular involvement in patients with severe gastrointestinal motility disorders. Gut. 2007;39:A18.
123. Hall KE, Wiley JW. I. New insights into neuronal injury: a cautionary tale. Am J Physiol Gastrointest Liver Physiol. 1998;274(6):G978-83.
124. De Giorgio R, Camilleri M. Human enteric neuropathies: morphology and molecular pathology. Neurogastroenterol Motil. 2004;16(5):515-31.
125. Stanghellini V, Cogliandro RF, de Giorgio R, et al. Chronic intestinal pseudo-obstruction: manifestations, natural history and management. Neurogastroenterol Motil. 2007;19(6):440-52.
126. Hockenbery D, Nunez G, Milliman C, et al. Bcl-2 is an inner mitochondrial membrane protein that blocks programmed cell death. Nature. 1990;348(6299):334-6.
127. De Giorgio R, Barbara G, Stanghellini V, et al. Reduced BCL-2 expression in the enteric nervous system as a marker for neural degeneration in patients with gastrointestinal motor disorders. Gastroenterology. 2000;118(4):A867.
128. Burns AJ. Disorders of interstitial cells of Cajal. J Pediatr Gastroenterol Nutr. 2007;45(Suppl 2):S103-6.
129. Huizinga JD, Thuneberg L, Kluppel M, et al. W/kit gene required for interstitial cells of Cajal and for intestinal pacemaker activity. Nature. 1995;373(6512):347-9.
130. De Giorgio R, Seri M, Cogliandro R, et al. Analysis of candidate genes for intrinsic neuropathy in a family with chronic idiopathic intestinal pseudo-obstruction. Clin Genet. 2001;59(2):131-3.
131. Ward SM, Sanders KM, Hirst GDS. Role of interstitial cells of Cajal in neural control of gastrointestinal smooth muscles. Neurogastroenterol Motil. 2004;16(Suppl 1):112-17.
132. He CL, Burgart L, Wang L, et al. Decreased interstitial cell of Cajal volume in patients with slow-transit constipation. Gastroenterology. 2000;118(1):14-21.
133. Boeckxstaens GE, Rumessen JJ, de Wit L, et al. Abnormal distribution of the interstitial cells of Cajal in an adult patient with pseudo-obstruction and megaduodenum. Am J Gastroenterol. 2002;97(8):2120-6.
134. De Giorgio R, Guerrini S, Barbara G, et al. Inflammatory neuropathies of the enteric nervous system. Gastroenterology. 2004;126(7):1872-83.
135. Knowles CH, Silk DBA, Darzi A, et al. Deranged smooth muscle alpha-actin as a biomarker of intestinal pseudo-obstruction: a controlled multinational case series. Gut. 2004;53(11):1583-9.
136. Gargiulo A, Auricchio R, Barone MV, et al. Filamin A is mutated in X-linked chronic idiopathic intestinal pseudo-obstruction with central nervous system involvement. Am J Hum Genet. 2007;80(4):751-8.
137. Stanghellini V, Camilleri M, Malagelada JR. Chronic idiopathic intestinal pseudo-obstruction: clinical and intestinal manometric findings. Gut. 1987;28(1):5-12.
138. Giordano C, Sebastiani M, De Giorgio R, et al. Gastrointestinal dysmotility in mitochondrial neurogastrointestinal encephalomyopathy is caused by mitochondrial DNA depletion. Am J Pathol. 2008;173(4):1120-8.
139. Nishino I, Spinazzola A, Papadimitriou A, et al. Mitochondrial neurogastrointestinal encephalomyopathy: an autosomal recessive disorder due to thymidine phosphorylase mutations. Ann Neurol. 2000;47(6):792-800.
140. Spinazzola A, Marti R, Nishino I, et al. Altered thymidine metabolism due to defects of thymidine phosphorylase. J Biol Chem. 2002;277(6):4128-33.
141. Giordano C, Sebastiani M, Plazzi G, et al. Mitochondrial neurogastrointestinal encephalomyopathy: evidence of mitochondrial DNA depletion in the small intestine. Gastroenterology. 2006;130(3):893-901.
142. Nishino I, Spinazzola A, Hirano M. Thymidine phosphorylase gene mutations in MNGIE, a human mitochondrial disorder. Science. 1999;283(5402):689-92.
143. Schuffler MD, Pope CE2nd. Studies of idiopathic intestinal pseudoobstruction. II. Hereditary hollow visceral myopathy: family studies. Gastroenterology. 1977;73(2):339-44.
144. Milla PJ. Endothelins, pseudo-obstruction and Hirschsprung’s disease. Gut. 1999;44(2):148-9.
145. Hageman MJ, Delleman JW. Heterogeneity in Waardenburg syndrome. Am J Hum Genet. 1977;29:468-85.
146. Jacobs JM, Wilson J. An unusual demyelinating neuropathy in a patient with Waardenburg’s syndrome. Acta Neuropathol. 1992;83:670-4.
147. Touraine RL, Attié-Bitach T, Manceau E, et al. Neurological phenotype in Waardenburg syndrome type 4 correlates with novel SOX10 truncating mutations and expression in developing brain. Am J Hum Genet. 2000;66:1496-503.
148. Tan F, Tan Y, Lim D. The significance of cystic adrenal lesions in multiple endocrine neoplasia IIB syndrome. Singapore Med J. 2004;45(10):494-6.
149. Bonsib SM, Fallon B, Mitros FA, Anuras S. Urological manifestations of patients with visceral myopathy. J Urol. 1984;132(6):1112-16.
150. Di Ciaula A, Covelli M, Berardino M, et al. Gastrointestinal symptoms and motility disorders in patients with systemic scleroderma. BMC Gastroenterol. 2008;8:7.
151. Levine MS, Rubesin SE, Laufer I. Pattern approach for diseases of mesenteric small bowel on barium studies. Radiology. 2008;249(2):445-60.
152. Soudah HC, Hasler WL, Owyang C. Effect of octreotide on intestinal motility and bacterial overgrowth in scleroderma. N Engl J Med. 1991;325(21):1461-7.
153. Greydanus MP, Camilleri M. Abnormal postcibal antral and small bowel motility due to neuropathy or myopathy in systemic sclerosis. Gastroenterology. 1989;96(1):110-15.
154. Greydanus MP, Camilleri M, Colemont LJ, et al. Ileocolonic transfer of solid chyme in small intestinal neuropathies and myopathies. Gastroenterology. 1990;99(1):158-64.
155. Weston S, Thumshirn M, Wiste J, Camilleri M. Clinical and upper gastrointestinal motility features in systemic sclerosis and related disorders. Am J Gastroenterol. 1998;93(7):1085-9.
156. Narnaez J, Perez-Vega C, Castro-Bohorquez FJ, et al. Intestinal pseudo-obstruction in systemic lupus erythematosus. Scand J Rheumatol. 2003;32:191-5.
157. Camilleri M, Malagelada JR. Abnormal intestinal motility in diabetics with the gastroparesis syndrome. Eur J Clin Invest. 1984;14(6):420-7.
158. Norman DA, Fleischmann RM. Gastrointestinal systemic sclerosis in serologic mixed connective tissue disease. Arthritis Rheum. 1978;21(7):811-19.
159. He CL, Soffer EE, Ferris CD, et al. Loss of interstitial cells of Cajal and inhibitory innervation in insulin-dependent diabetes. Gastroenterology. 2001;121(2):427-34.
160. Edwards LL, Pfeiffer RF, Quigley EM, et al. Gastrointestinal symptoms in Parkinson’s disease. Movement Disord. 1991;6(2):151-6.
161. Lewitan A, Nathanson L, Slade WRJr. Megacolon and dilatation of the small bowel in parkinsonism. Gastroenterology. 1951;17(3):367-74.
162. Bozeman T, Anuras S, Hutton T, et al. Small intestinal manometry in Parkinson’s disease. Gastroenterology. 1990;99(4):1202.
163. Kupsky WJ, Grimes MM, Sweeting J, et al. Parkinson’s disease and megacolon: concentric hyaline inclusions (Lewy bodies) in enteric ganglion cells. Neurology. 1987;37(7):1253-5.
164. Singaram C, Ashraf W, Gaumnitz EA, et al. Dopaminergic defect of enteric nervous system in Parkinson’s disease patients with chronic constipation. Lancet. 1995;346(8979):861-4.
165. Fealey RD, Szurszewski JH, Merritt JL, DiMagno EP. Effect of traumatic spinal cord transection on human upper gastrointestinal motility and gastric emptying. Gastroenterology. 1984;87(1):69-75.
166. Bruninga K, Camilleri M. Colonic motility and tone after spinal cord and cauda equina injury. Am J Gastroenterol. 1997;92(5):891-4.
167. Fajardo NR, Pasiliao RV, Modeste-Duncan R, et al. Decreased colonic motility in persons with chronic spinal cord injury. Am J Gastroenterol. 2003;98(1):128-34.
168. Stiens SA, Bergman SB, Goetz LL. Neurogenic bowel dysfunction after spinal cord injury: clinical evaluation and rehabilitative management. Arch Phys Med Rehabil. 1997;78(Suppl 3):S86-102.
169. Pinsk I, Dukhno O, Ovnat A, Levy I. Gastrointestinal complications of von Recklinghausen’s disease: two case reports and a review of the literature. Scand J Gastroenterol. 2003;38(12):1275-8.
170. Fuller CE, Williams GT. Gastrointestinal manifestations of type 1 neurofibromatosis (von Recklinghausen’s disease). Histopathology. 1991;19(1):1-11.
171. Honnorat J, Antoine J-C. Paraneoplastic neurological syndromes. Orphanet J Rare Dis. 2007;2:22.
172. Darnell RB, Posner JB. Paraneoplastic syndromes involving the nervous system. N Engl J Med. 2003;349(16):1543-4.
173. Sculier JP, Feld R, Evans WK, et al. Neurologic disorders in patients with small cell lung cancer. Cancer. 1987;60(9):2275-83.
174. Simpson A, Khilnani M. Gastrointestinal manifestations of the muscular dystrophies. Am J Roentgenol. 1975;125:948.
175. Nowak TV, Anuras S, Brown BP, et al. Small intestinal motility in myotonic dystrophy patients. Gastroenterology. 1984;86(5 Pt 1):808-13.
176. Yoshida MM, Krishnamurthy S, Wattchow DA, et al. Megacolon in myotonic dystrophy caused by a degenerative neuropathy of the myenteric plexus. Gastroenterology. 1988;95(3):820-7.
177. Pennisi E. Genetics. Hopping to a better protein. Science. 2008;322(5907):1454-5.
178. Wagner KR. Approaching a new age in Duchenne muscular dystrophy treatment. Neurotherapeutics. 2008;5(4):583-91.
179. McDonald GB, Schuffler MD, Kadin ME, Tytgat GN. Intestinal pseudoobstruction caused by diffuse lymphoid infiltration of the small intestine. Gastroenterology. 1985;89(4):882-9.
180. Borrelli O, Salvia G, Mancini G, et al. Evolution of gastric electrical features and gastric emptying in children with Duchenne and Becker muscular dystrophy. Am J Gastroenterol. 2005;100:695-702.
181. Westermark P. Aspects on human amyloid forms and their fibril polypeptides. FEBS J. 2005;272(23):5942-5949.
182. Ebert EC, Nagar M. Gastrointestinal manifestations of amyloidosis. Am J Gastroenterol. 2008;103(3):776-87.
183. Matsumoto T, Iida M, Hirakawa M, et al. Breath hydrogen test using water-diluted lactulose in patients with gastrointestinal amyloidosis. Dig Dis Sci. 1991;36(12):1756-60.
184. Suhr O, Danielsson A, Steen L. Bile acid malabsorption caused by gastrointestinal motility dysfunction? An investigation of gastrointestinal disturbances in familial amyloidosis with polyneuropathy. Scand J Gastroenterol. 1992;27(3):201-7.
185. Schein J. Syndrome of nontropical sprue with hitherto undescribed lesions of the intestine. Gastroenterology. 1947;8:438-60.
186. Guirl MJ, Hogenauer C, Santa Ana CA, et al. Rapid intestinal transit as a primary cause of severe chronic diarrhea in patients with amyloidosis. Am J Gastroenterol. 2003;98(10):2219-25.
187. Battle WM, Rubin MR, Cohen S, Snape WJJr. Gastrointestinal-motility dysfunction in amyloidosis. N Engl J Med. 1979;301(1):24-5.
188. Camilleri M, Malagelada JR, Stanghellini V, et al. Gastrointestinal motility disturbances in patients with orthostatic hypotension. Gastroenterology. 1985;88(6):1852-9.
189. Gilat T, Spiro H. Amyloidosis and the gut. Am J Dig Dis. 1968;13:619-33.
190. Kyle RA, Gertz MA. Primary systemic amyloidosis: clinical and laboratory features in 474 cases. Semin Hematol. 1995;32(1):45-59.
191. Briggs GW. Amyloidosis. Ann Intern Med. 1961;55:943-57.
192. Gertz MA, Kyle RA. Secondary systemic amyloidosis: response and survival in 64 patients. Medicine (Baltimore). 1991;70(4):246-56.
193. Caballeria J, Bruguera M, Sole M, et al. Hepatic familial amyloidosis caused by a new mutation in the apolipoprotein AI gene: clinical and pathological features. Am J Gastroenterol. 2001;96(6):1872-6.
194. Troncon LE, Aprile LR, Oliveira RB, Iazigi N. Abnormally rapid gastric emptying of an isosmotic liquid meal in patients with megaduodenum. Dig Dis Sci. 2000;45(11):2145-50.
195. Kahraman H, Kaya N, Demircali A, et al. Gastric emptying time in patients with primary hypothyroidism. Eur J Gastroenterol Hepatol. 1997;9(9):901-4.
196. Shafer RB, Prentiss RA, Bond JH. Gastrointestinal transit in thyroid disease. Gastroenterology. 1984;86(5 Pt 1):852-5.
197. Duret RL, Bastenie PA. Intestinal disorders in hypothyroidism. Clinical and manometric study. Am J Dig Dis. 1971;16(8):723-7.
198. Cappell MS. Toxicity of administered drugs and chemicals. Am J Gastroenterol. 2004;99:1175-90.
199. Schultz HS, Vernon B. Intestinal pseudo-obstruction related to using verapamil. West J Med. 1989;151:556-8.
200. Dawson DJ, Sciberras CM, Whitwell H. Coeliac disease presenting with intestinal pseudo-obstruction. Gut. 1984;25(9):1003-8.
201. Behm B, Stollman N. Postoperative ileus: etiologies and interventions. Clin Gastroenterol Hepatol. 2003;1(2):71-80.
202. Otterson MF, Sarna SK, Lee MB. Fractionated doses of ionizing radiation alter postprandial small intestinal motor activity. Dig Dis Sci. 1992;37(5):709-15.
203. Summers RW, Glenn CE, Flatt AJ, Elahmady A. Does irradiation produce irreversible changes in canine jejunal myoelectric activity? Dig Dis Sci. 1992;37(5):716-22.
204. Fernandez-Banares F, Villa S, Esteve M, et al. Acute effects of abdominopelvic irradiation on the orocecal transit time: its relation to clinical symptoms, and bile salt and lactose malabsorption. Am J Gastroenterol. 1991;86(12):1771-7.
205. Perino LE, Schuffler MD, Mehta SJ, Everson GT. Radiation-induced intestinal pseudoobstruction. Gastroenterology. 1986;91(4):994-8.
206. Arista-Nasr J, Gonzalez-Romo M, Keirns C, Larriva-Sahd J. Diffuse lymphoplasmacytic infiltration of the small intestine with damage to nerve plexus. A cause of intestinal pseudo-obstruction. Arch Pathol Lab Med. 1993;117(8):812-19.
207. Basilisco G, Gebbia C, Peracchi M, et al. Cerebellar degeneration and hearing loss in a patient with idiopathic myenteric ganglionitis. Eur J Gastroenterol Hepatol. 2005;17(4):449-52.
208. Kamal N, Chami T, Andersen A, et al. Delayed gastrointestinal transit times in anorexia nervosa and bulimia nervosa. Gastroenterology. 1991;101(5):1320-4.
209. Cogliandro RF, De Giorgio R, Barbara G, et al. Chronic intestinal pseudo-obstruction. Best Pract Res Clin Gastroenterol. 2007;21(4):657-69.
210. Iyer KR, Horslen S, Torres C, et al. Functional liver recovery parallels autologous gut salvage in short bowel syndrome. J Pediatr Surg. 2004;39(3):340-4.
211. Kerckhoffs APM, Visser MR, Samsom M, et al. Critical evaluation of diagnosing bacterial overgrowth in the proximal small intestine. J Clin Gastroenterol. 2008;42(10):1095-102.
212. Bauer TM, Schwacha H, Steinbruckner B, et al. Diagnosis of small intestinal bacterial overgrowth in patients with cirrhosis of the liver: poor performance of the glucose breath hydrogen test. J Hepatol. 2000;33(3):382-6.
213. Tsujimoto T, Shioyama E, Moriya K, et al. Pneumatosis cystoides intestinalis following alpha-glucosidase inhibitor treatment: A case report and review of the literature. World J Gastroenterol. 2008;14(39):6087-92.
214. Seket B, Kaczmarek D, Tiffet O. Intestinal pseudo-obstruction and pneumotosis cystoides in a scleroderma patient. J Am Coll Surg. 2007;205:180-1.
215. Mousa H, Hyman PE, Cocjin J, et al. Long-term outcome of congenital intestinal pseudoobstruction. Dig Dis Sci. 2002;47(10):2298-305.
216. Faure CM, Goulet OM, Ategbo SM, et al. Chronic intestinal pseudoobstruction syndrome: clinical analysis, outcome, and prognosis in 105 children. Dig Dis Sci. 1999;44(5):953-9.
217. Stanghellini V, Cogliandro RF, De Giorgio R, et al. Natural history of chronic idiopathic intestinal pseudo-obstruction in adults: a single center study. Clin Gastroenterol Hepatol. 2005;3(5):449-58.
218. Metcalf AM, Phillips SF, Zinsmeister AR, et al. Simplified assessment of segmental colonic transit. Gastroenterology. 1987;92(1):40-7.
219. Freye E, Latasch L. Effects of tramadol and tilidine/naloxone on oral-caecal transit and pupillary light reflex. Arzneimittelforschung. 2000;50(1):24-30.
220. Wilder-Smith CH, Hill L, Spargo K, Kalla A. Treatment of severe pain from osteoarthritis with slow-release tramadol or dihydrocodeine in combination with NSAID’s: a randomised study comparing analgesia, antinociception and gastrointestinal effects. Pain. 2001;91(1-2):23-31.
221. Field MJ, Oles RJ, Lewis AS, et al. Gabapentin (neurontin) and S-(+)-3-isobutylgaba represent a novel class of selective antihyperalgesic agents. Br J Pharmacol. 1997;121(8):1513-22.
222. Camilleri M, Malagelada JR, Abell TL, et al. Effect of six weeks of treatment with cisapride in gastroparesis and intestinal pseudoobstruction. Gastroenterology. 1989;96(3):704-12.
223. Di Lorenzo C, Reddy SN, Villanueva-Meyer J, et al. Cisapride in children with chronic intestinal pseudoobstruction. An acute, double-blind, crossover, placebo-controlled trial. Gastroenterology. 1991;101(6):1564-70.
224. Camilleri M, Balm RK, Zinsmeister AR. Determinants of response to a prokinetic agent in neuropathic chronic intestinal motility disorder. Gastroenterology. 1994;106(4):916-23.
225. Foxx-Orenstein A, Camilleri M, Stephens D, Burton D. Effect of a somatostatin analogue on gastric motor and sensory functions in healthy humans. Gut. 2003;52(11):1555-61.
226. Soudah HC, Hasler WL, Owyang C. Effect of octreotide on intestinal motility and bacterial overgrowth in scleroderma. N Engl J Med. 1991;325:1508-9.
227. Anuras S, Shirazi S, Faulk DL, et al. Surgical treatment in familial visceral myopathy. Ann Surg. 1979;189(3):306-10.
228. Pironi L, Spinucci G, Paganelli F, et al. Italian guidelines for intestinal transplantation: potential candidates among the adult patients managed by a medical referral center for chronic intestinal failure. Transplant Proc. 2004;36(3):659-61.
229. Lauro A, Zanfi C, Ercolani G, et al. Italian experience in adult clinical intestinal and multivisceral transplantation: 6 years later. Transpl Proc. 2007;39(6):1987-91.
230. Barnes PR, Lennard-Jones JE, Hawley PR, Todd IP. Hirschsprung’s disease and idiopathic megacolon in adults and adolescents. Gut. 1986;27(5):534-41.