Chapter 1 Historical Perspective on Three-Dimensional Echocardiography
Earliest Approaches
3DE had its beginnings in the 1970s with primitive equipment and equally primitive images. By the mid-1980s, there was early 3DE performed with standard B-mode ultrasound and tracking devices designed to locate the transducer in space. The initial approach to 3D echo was to obtain multiple 2D images and reconstruct them into a 3D image. This was accomplished by registering the 2D images such that it could be discerned how the individual images fit together to form the 3D image. Such registration of the images was achieved by tracking the transducer in space via mechanical, acoustic, electromagnetic, or optical detection apparatus. In the mechanical approach, the transducer had an actual mechanical arm attached to it and therefore dictated movement in space (Figure 1-1). Dekker and colleagues1 are credited with this first iteration of 3DE using the mechanical arm approach in 1974. Next came an acoustic attempt at location, the “spark gap” technique by Moritz and Shreve2 in 1976. In this technique, the transducer was located by sending pulsed acoustic signals from a device holding the transducer, called a spark gap to a Cartesian locator grid (Figure 1-2). This approach, in theory, allowed free-hand scanning—meaning that the transducer did not have to follow a predetermined pathway and, indeed, was the precursor to free-hand scanning using an electromagnetic locator. Both electrocardiography and respiratory gating were often used, or images were acquired with breath holding. Initially, only end-diastolic and end-systolic images of the left ventricle (LV) were obtained. Over time, complete cardiac cycles could be obtained.3,4 Several groups published articles during this period using a variety of primitive transducer tracking systems and the resultant less than pleasing 3D imaging.5–10
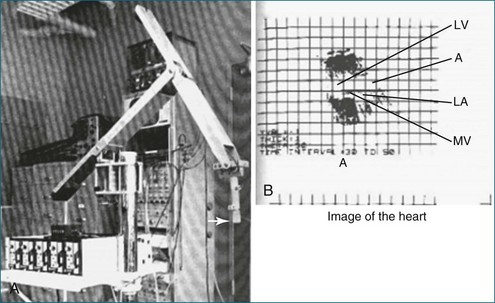
Figure 1-1 A, The mechanical arm used by Dekker and colleagues1 for tracking the ultrasound transducer in space. Note the arrow depicting the actual ultrasound transducer. As might be imagined, this apparatus was not well received by sonographers because moving the transducer around was physically taxing, given the weight and awkwardness of the mechanical arm. B, Image of the heart. Note that the image shows only mild resemblance to the actual heart and heart chambers. A, aorta; LA, left atrium; LV, left ventricle; MV, mitral valve.
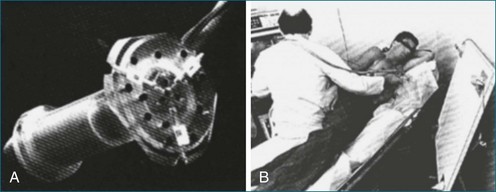
Figure 1-2 An acoustic “spark gap” apparatus used by Moritz and Shreve2 for locating the transducer for three-dimensional echocardiography. A, Actual device housing the transducer, with the audio signal sent from the trifurcating mechanism. B, The device in practice, with the coordinates shown on the board to the right of the user.
In 1977, Raab and colleagues11 developed the magnetic locator, which ultimately led to the most advanced type of free-hand scanning, but this method for transducer location never caught on until the mid-1990s. This method is still used today by some labs, predominantly for research purposes (Figure 1-3; see also Figures 1-13, 1-14).
In between the spark gap and the free-hand magnetic approaches were myriad tactics that used the technique of transducer movement in a preprogrammed, stepwise fashion while attempting to keep the patient and the sonographer as stationary as possible. The mechanical devices then moved the transducer in a linear, fanlike, or rotational direction. An example of a fanlike acquisition is shown in Figure 1-4.
Fanlike Scanning
Fanlike scanning was, in theory, advantageous for evaluating the right ventricle (RV) compared with rotational or linear scanning, since the RV is not easily accessed from the apex and often is best imaged from the RV inflow tract using a more rightward direction of scanning from the standard parasternal view.12
Rotational Scanning
The rotational approach used a cylinder-like mechanical apparatus that those in the industry at that time referred to as the “bazooka” (Figure 1-5). This device had a mechanical stepper motor that moved the transducer sequentially through a semicircular or 180-degree scan with stops every two to three degrees to acquire a 2D image. The acquisition of roughly 60 images was followed by computer software processing, initially using a polar coordinate approach to meld the images into a 3D image. The computer software used significant interpolation between images. The result was frequent “stitch” artifact caused by deficiencies in image line-up from patient movement, transducer movement outside the prespecified position, respiratory or cardiac gating artifact, or all of these factors. Although innovative and potentially very powerful for this era, clear limitations remained. Hence, clinical applicability was very limited.13
Linear or Parallel Scanning
In linear scanning, the transducer was sequentially stepped along a line and obtained images every few millimeters, as opposed to degrees with the rotational and fanlike scans (Figures 1-6 to 1-9). Examples of linear scanning using an intravascular ultrasound, predominantly for coronary imaging, as well as a transthoracic echocardiography (TTE) device are shown in the figures. A device, known as “lobster tail,” for linear pullback using transesophageal echocardiography (TEE) was available as well. In similar fashion as the previously described linear devices, the lobster tail operated by stepwise pullback of the TEE probe in the esophagus, with imaging at each stop. This TEE probe, developed by TomTec, Inc. (Munich, Germany), included both the TEE probe and the “stepper,” that is, the device that moved the probe sequentially (see Figures 1-8 and 1-9). There were several iterations of this technique, including one that involved placing the TEE probe in a water bath within the esophagus to serve as a “standoff” to improve acoustic effects and sharpen the images (see Figure 1-8). The rotational method of scanning with the lobster tail is summarized in Figure 1-9. Further summaries regarding the various types of scanning, early fetal ultrasound, and early computer hardware are shown in Figures 1-10 to 1-12.
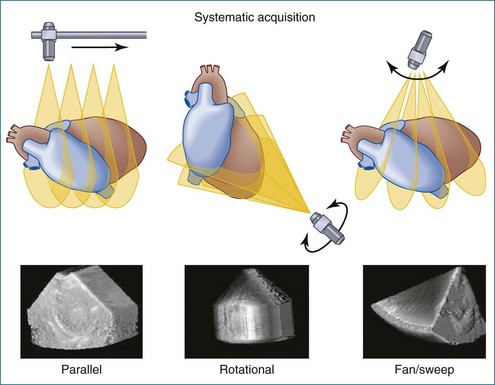
Figure 1-10 Summary of linear (parallel), rotational, and fanlike scanning.
(Courtesy TomTec, Inc., Munich, Germany.)
More Advanced Free-Hand Scanning
Later, using the technology developed by Raab, free-hand scanning became commercially available from TomTec and from 3D Echo Tech (Boulder, CO; later acquired by GE Medical systems). Both systems used an enhanced version of the electromagnetic detector described by Raab in 1977 (Figures 1-13 and 1-14; see also Figure 1-3). A magnet was enclosed in a plastic housing and placed close to the patient. The electromagnetic locator was electrically wired to a sensor that was placed on the ultrasound transducer and connected to a microcomputer and the commercially available ultrasound system. This enhanced version of the electromagnetic detector is still commercially available today through Ascension Technologies (Milton, VT). Before using this device for free-hand scanning, a calibration must be performed, typically using a phantom that had multiple wires passing through a water bath (see Figure 1-14). This process is tedious and time consuming and is a major reason for the lack of clinical acceptance. Currently, companies that use the electromagnetic sensor for free-hand scanning often hire out the calibration process so the user does not need to become involved in the process. An example of quantitative left ventricular imaging using the method the electromagnetic locator is provided by Legget et al.14 As computing power improved in the late 1980s and early 1990s, the data processing algorithms for 2D images became advanced and allowed direct conversion of basic images from a polar coordinate system to the cartesian coordinate system. With the evolution of interpolation, gaps between images could be filled, and with the addition of smoothing algorithms, the images could be more systematically reconstructed to resemble an entire heart—or at least individual ventricles.
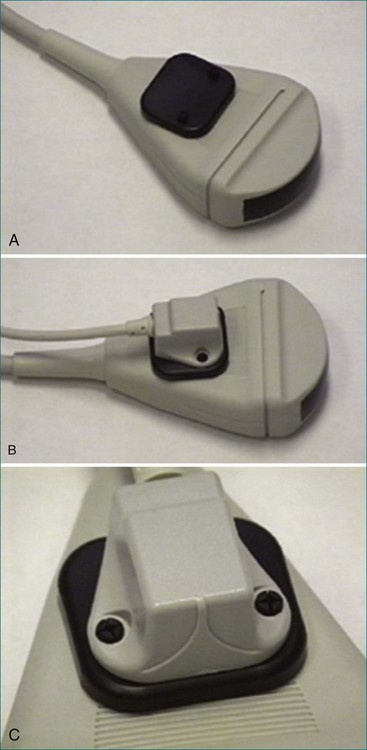
Figure 1-13 A to C, Electromagnetic sensor placed on a general ultrasound transducer.
(Courtesy TomTec, Inc., Munich, Germany.)
On-Board Rotational 3D Transesophageal and Transtracheal Echocardiogrphy
In 1993, TomTec partnered with Hewlett-Packard (HP, Palo Alto, CA) to develop a rotation motor for the existing HP TEE multiple probe (Omniplane I) (Figure 1-15). This allowed rotational scanning using an already available TEE probe. In 1995, the partnership between TomTec and HP progressed even further and led to the development of software that was embedded in the HP 5500 Ultrasound system (Figure 1-16). The technique for this system is summarized in Figure 1-17. Examples of some of the images obtained with the rotational TEE approach are shown in Figures 1-18 to 1-23. In addition, HP developed a transthoracic transducer during the same period (see Figure 1-23). Although a transducer with the rotational mechanism contained within the ultrasound probe was much less cumbersome than holding a conventional probe within the rotational device (shown in Figure 1-5), the image quality was not found to be superior and this transducer was short lived. For the next 7 years or so, 3DE would mostly be performed with the rotational TEE approach. This approach occasionally was quite impressive in the evaluation of the mitral valve but did not offer much for evaluating LV function and size, particularly for quantification.15,16
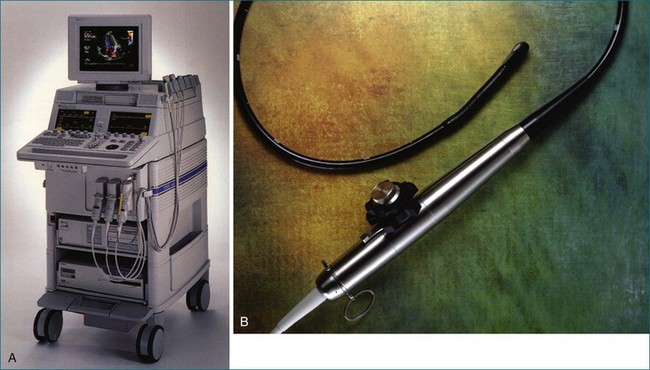
Figure 1-16 Later, Hewlett-Packard and TomTec, Inc. partnered to use a standard ultrasound system (the HP 5500, A) and standard transesophageal echocardiography (TEE) probe (the Omni II, B) and an onboard software program that achieved the same result as shown in Figure 1-15 without needing external hardware to drive the TEE probe.
(Courtesy TomTec, Inc., Munich, Germany.)
Real-Time Three-dimensional Methods
RT3DE was first developed by von Ramm at Duke University during the early 1990s.17,18 With RT3DE, an entire cardiac volume was obtained in one cardiac cycle. Since only one cardiac cycle was used, there was no “stitch” artifact caused by interpolation between multiple 2D images. The good news was that a volume of data had been acquired. The bad news, however, was that the output was in the form of multiple cross-sectional views of the LV that had the look of parasternal short-axis views. This gave rise to the term C scan (Figure 1-24). Each C scan was a cross-sectional cut through the 3D dataset. Note that in Figure 1-24, A and B, the four- and two-chamber orthogonal views are shown, as well as C1 and C2, the sequential short-axis or axial cuts through the heart. This technology was made commercially available in 1997 by Volumetrics (Chapel Hill, NC) (Figure 1-25). However, the ultrasound system was very large and did not have a separate transducer for performing 2D imaging. Hence, only what was supposedly 3D imaging could be performed. This technology used a sparse-array transducer, as shown in Figure 1-26, with not all of the area of the transducer head being connected to individual elements. In addition, to derive true 3D volume rendering, an offline workstation had to be used. Hence, there were no truly “live” 3D echo images. Volumetrics did show RT3DE rendering at the 2000 American Heart Association meetings, but within months after this showing, the company ceased operations. Undoubtedly, this was a business decision based on the knowledge that a similar, yet much more advanced product was already well into the development stage at HP. The development stage for RT3DE began at HP around 1996. As mentioned earlier, HP had already been a force in the industry when the company co-developed rotational 3D TEE scanning with TomTec and released that product to the general market in 1995. By late 1998, HP had a working prototype for transthoracic RT3DE; it was undoubtedly being tested at specialized 3D centers such as those at the thorax center in Rotterdam, The Netherlands; by Dr. Andreas Francke in Hannover, Germany; and at the University of Chicago echocardiography lab in the United States. HP began showing the prototypes to luminary customers by 1999. A major development in the history of RT3DE came in 1999 when HP spun off its medical division and other computer technology divisions into an entity called Agilent Technologies (Andover, MA). The next year, the medical division of Agilent (excluding the measurement and chemical testing divisions) was bought by Philips Medical Systems of The Netherlands, now known as Philips Health Care. Philips had bought another ultrasound company in 1998, Advanced Technology Laboratories (ATL) of Bothell, Washington, in 1998. Hence, after the HP spinoff to Agilent, the purchase of Agilent by Philips and the already existing ATL, the plan was to merge the ultrasound knowledge to further develop 3D technology as well as other echocardiography applications. The U.S. headquarters for Philips Health was established in Andover, Massachusetts, and the sales headquarters in Bothell, Washington. Thereafter, in 2002, at the time of the American Heart Association meetings, Philips released the first generation of its RT3DE, which used a transthoracic transducer. This transducer, which was significantly larger that standard 2D transducers, was a dense-array transducer (Figures 1-27 to 1-29) and had more than 3000 elements, as opposed to the 256 in the sparse-array unit.
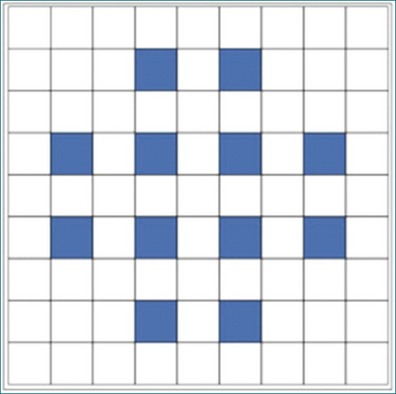
Figure 1-26 The Volumetrics (Chapel Hill, NC) system used sparse-array imaging, which limited image resolution.
The greatest impact on patient care with regard to 3D and RT3DE happened when Philips introduced the RT3DTEE probe in 2007 at the American Heart Association meetings and earlier that year in Europe (Figures 1-30 to 1-34). At the time of this writing, General Electric is releasing an RT3DTEE system, 5 years after the release of the first one by Philips Health Care. RT3DTEE has been a particular advance with regard to patient treatment, particularly for the treatment of structural heart disease in the interventional lab. Treatments involving percutaneous intervention on the mitral valve are now possible using RT3DTEE guidance. The use of RT3DTEE in the interventional lab is outlined in Chapter 15.
Finally, in 2010, Philips Health Care introduced a TTE transducer for both RT2D and RT3DE imaging (Figure 1-35). The X5 transducer is the world’s first miniaturized matrix-type transducer that allows the user to perform both 2D and 3D echocardiographic imaging without switching transducers, with comparable 2D and 3D image quality.
The development of RT3DE in 2002 by Philips Health Care was a step that made the greatest impact toward 3DE being used on a regular basis by clinical cardiologists and not merely in the research arena. With its 2002 introduction, RT3DE has become more commonplace throughout the United States and worldwide. In fact, 3D technology has proliferated more widely and quickly into Europe and Asia than in United States. Further proliferation continues on a near exponential curve as the technology continues to improve, becomes more miniaturized, and develops more user-friendly interfaces with clearer integration into clinical practice without affecting the general workflow. This fact is discussed in more detail in Chapter 3.
Since the initial release of RT3DE by Philips in 2002, the volume of data generated from research in the field is enormous, with more than 3000 articles on 3DE cited on Medline during this period. A position paper and summary paper were published by the American Society of Echocardiography in 2007 to guide clinicians in the use of 3DE.19,20 The European Society of Cardiology has provided a website called 3D Echo Box to guide clinicians through all the articles that describe the use of 3D echocardiography (http://www.escardio.org/communities/EAE/3d-echo-box/Pages/welcome.aspx). The most recent guidelines were published in the January 2012 issue of the Journal of the American Society of Echocardiography.21
1 Dekker DL, Piziali RL, Dong B, Jr. A system for ultrasonically imaging the human heart in three dimensions. Compt Biomed Res. 1974;7:544–553.
2 Mortiz WE, Shreve PL. A microprocessor-based spatial locating system for use with diagnostic ultrasound IEEE. Trans Biomed Eng. 1976;64:966–974.
3 King DL, King DL, Jr., Shao MYC. Three-dimensional spatial registration and interactive display of position and orientation of real-time ultrasound images. J Ultrasound Med. 1990;9:525–532.
4 Gopal AS, King DL, Katz J, et al. Three-dimensional echocardiographic volume computation by polyhedral surface reconstruction: In vitro validation and comparison to magnetic resonance imaging. J Am Soc Echocardiogr. 1992;5:115–124.
5 Geiser EA, Lypkiewics SM, Christi LG, et al. A framework for three-dimensional time varying reconstruction of the human left ventricle: Sources of error and estimation of their magnitude. Compt Biomed Res. 1980;13:225–241.
6 Matsumoto M, Matsuo H, Kitabatake A, et al. Three-dimensional echocardiograms and two-dimensional echocardiographic images at desired planes by a computerized system. Ultrasound Med Biol. 1977;3:163–178.
7 Pearlman AS, Mortiz WE, Medema DK, et al. Three-dimensional reconstruction of the formalin-fixed ventricle using nonparallel two-dimensional ultrasonic scans. Circulation. 1981;64(Suppl IV):65.
8 Ghosh A, Nanda NC, Maurer G. Three-dimensional reconstruction of echocardiographic images using the rotation method. Ultrasound Med Biol. 1982;8:655–661.
9 Maurer G, Nanda NC. Two dimensional echocardiographic evaluation of exercise-induced left and right ventricular asynergy: Correlation with thallium scanning. Am J Cardiol. 1981;48:720–727.
10 Linker DT, Mortiz WE, Pearlman AS. A new three-dimensional echocardiographic method of right ventricular volume measurement: In vitro validation. J Am Coll Cardiol. 1986;8:101–106.
11 Raab FH, Blood EB, Steiner TO, et al. Magnetic position and orientation tracking system. IEEE Trans Aerosp Electron Syst. 1979;AES-15:709–718.
12 Delabays A, Pandian NG, Cao QL, et al. Transthoracic real-time three-dimensional echocardiography using a fan-like scanning approach for data acquisition: Methods, strengths, problems, and initial clinical experience. Echocardiography. 1995;12:49–59.
13 Salustri A, Roelandt JR. Ultrasonic three-dimensional reconstruction of the heart. Ultrasound Med Biol. 1995;21:281–293.
14 Legget ME, Leotta DF, Bolson EL, et al. System for quantitative three-dimensional echocardiography of the left ventricle based on a magnetic-field position and orientation sensing system. IEEE Trans Biomed Engineer. 1998;45:494–504.
15 Nanda N, Pinheiro L, Sanyal R, et al. Multiplane transesophageal echocardiographic imaging and three-dimensional reconstruction. Echocardiography. 1992;9:667–676.
16 Chen Q, Nosir YF, Vletter WB, et al. Accurate assessment of mitral valve area in patients with mitral stenosis by three-dimensional echocardiography. J Am Soc Echocardiogr (Official publication of the American Society of Echocardiography). 1997;10:133–140.
17 von Ramm OT, Smith SW. Real time volumetric ultrasound imaging system. J Digit Imag (Official journal of the Society for Computer Applications in Radiology). 1990;3:261–266.
18 von Ramm OT, Smith SW, Pavy HR. High-speed ultrasound volumetric imaging system. II. Parallel processing and image display. IEEE Transac Ultrason Ferroelectr Freq Control. 1991;38:109–115.
19 Hung Lang R, Flachskampf F, Shernan SK, et al. 3D echocardiography: A review of the current status and future directions. J Am Soc Echocardiogr. 2007;20:213–233.
20 Picard M. The time for 3D. J Am Soc Echocardiogr. 2007;20:A22–A23.
21 Lang RM, Badano LP, Tsang W, et al. Recommendations for image acquisition and display using three-dimensional echocardiography. J Am Soc Echocardiogr. 2012;25:3–46.