Chapter 20 General Principles of Infection
Etiology
Surgeon-Dependent Factors
Skin Preparation
Hand washing is the most important procedure for prevention of nosocomial infections. Studies suggest that hand scrubbing for 2 minutes is as effective as traditional hand scrubbing for 5 minutes. The optimal duration of hand scrubbing has yet to be determined. Hand rubbing with an aqueous alcohol solution that is preceded by a 1-minute nonantiseptic hand washing for the first case of the day was found by Parienti et al. to be just as effective in prevention of surgical site infections as traditional hand scrubbing with antiseptic soap. The effectiveness of common antiseptics is summarized in Table 20-1.
Prophylactic Antibiotic Therapy
Methicillin-Resistant Staphylococcus Aureus
The evolution of S. aureus into a multiple-drug–resistant pathogen (methicillin-resistant S. aureus [MRSA]) has become a major health concern worldwide. Approximately 57% of S. aureus bacteria are methicillin resistant, and now vancomycin-resistant strains are being reported. This is probably one of the most worrisome problems in the fight against bacterial infections. Initially, MRSA was seen only in hospital settings and long-term care facilities; however, it is now becoming increasingly prevalent in young, healthy individuals in the community (Table 20-2; at-risk groups), and it is particularly virulent. The mortality rate associated with invasive MRSA infections is 20%.
TABLE 20-2 At-Risk Groups and Risk Factors for Community-Acquired Methicillin-Resistant Staphylococcus aureus
AT-RISK GROUPS | RISK FACTORS |
---|---|
From Marcotte AL, Trzeciak MA: Community-acquired methicillin-resistant Staphylococcus aureus: an emerging pathogen in orthopaedics, J Am Acad Orthop Surg 16:98, 2008.
Because of the prevalence of community acquired (CA)-MRSA, it is necessary to rapidly identify the organism, determine antibiotic sensitivity, and begin antibiotic therapy (for empirical coverage see Table 22-2). For invasive infections, intravenous vancomycin is recommended or, alternatively, daptomycin, gentamicin, and linezolid can be used. In cases of necrotizing fasciitis, clindamycin, gentamicin, rifampin, trimethoprim-sulfamethoxazole, and vancomycin are effective. Until a sensitivity determination can be made, antimicrobial coverage specifically of CA-MRSA is recommended. For deep subperiosteal abscess or superficial abscess, irrigation and débridement are necessary to reduce bacterial counts. Obtaining an infectious disease consult is highly recommended.
Diagnosis
Laboratory Studies
A complete blood cell count, including differential and erythrocyte sedimentation rate (ESR) and C-reactive protein (CRP), should be obtained during initial evaluation of bone and joint infections. The white blood cell count is an unreliable indicator of infection and often is normal even when infection is present. The differential shows increases in neutrophils during acute infections. The ESR becomes elevated when infection is present, but this does not occur exclusively in the presence of infection. Fractures or other underlying diseases can cause elevation of the ESR. The ESR also is unreliable in neonates, patients with sickle cell disease, patients taking corticosteroids, and patients whose symptoms have been present for less than 48 hours. Peak elevation of the ESR occurs at 3 to 5 days after infection and returns to normal approximately 3 weeks after treatment is begun. CRP, synthesized by the liver in response to infection, is a better way to follow the response of infection to treatment. CRP increases within 6 hours of infection, reaches a peak elevation 2 days after infection, and returns to normal within 1 week after adequate treatment has begun. Other tests, such as the S. aureus surface antigen or antibody test and counterimmunofluorescence studies of the urine, are promising, but their usefulness in clinical situations has not been proved. Material obtained from aspiration of joint fluid can be sent to the laboratory for a cell count and differential to distinguish acute septic arthritis from other causes of arthritis. In septic arthritis, the cell count usually is greater than 80,000/mm3, with more than 75% of the cells being neutrophils (Table 20-3). Jung et al. devised an algorithm to predict the probability of septic arthritis in children (Table 20-4). A Gram stain also should be obtained. Gram stains identify the types of organisms (gram-positive or gram-negative) in about a third of bone and joint aspirates. Intraoperative frozen section also should be obtained in cases in which infection is suspected. A white blood cell count greater than 10 per high-power field is considered indicative of infection, whereas a count less than 5 per high-power field all but excludes infection.
LEUKOCYTES | NEUTROPHILS (%) | |
---|---|---|
Normal | <200 | <25 |
Traumatic | <5,000 | <25 |
Toxic synovitis | 5,000-15,000 | <25 |
Acute rheumatic fever | 10,000-15,000 | 50 |
Juvenile rheumatoid arthritis | 15,000-80,000 | 75 |
Septic arthritis | >80,000 | >75 |
From Morrissy RT: Septic arthritis. In Gustilo RB, Genninger RP, Tsukayama DT, editors: Orthopaedic infection: diagnosis and treatment, Philadelphia, 1989, WB Saunders.
Imaging Studies
Radiographic studies are helpful but are not as useful in the diagnosis of acute bone and joint infections as they are in following responses to treatment. Plain radiographs show soft tissue swelling, joint space narrowing or widening, and bone destruction (Fig. 20-1). Bone destruction is not apparent on radiographs, however, until an infection has been present for 10 to 21 days. In addition, 30% to 50% of the bone matrix must be lost to show a lytic lesion on radiographs (Fig. 20-2). Wheat found that fewer than 5% of plain radiographs were initially abnormal in bone and joint infections, and fewer than 30% were abnormal at 1 week; however, 90% were abnormal at 3 to 4 weeks. If initial radiographs are normal in the evaluation of bone and joint infections, other imaging methods that show soft tissue swelling and loss of normal fat planes around the involved bone or joint should be used.
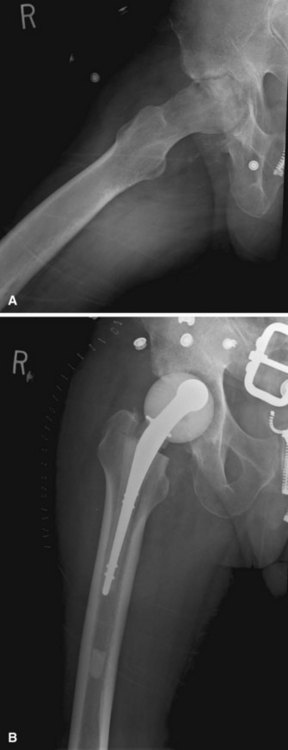
FIGURE 20-1 A, Radiographic evidence of acute bone and joint infection. B, Treatment with antibiotic cement spacer.
(Courtesy of Andrew Crenshaw.)
Conventional tomography can be useful in identifying a sequestrum or subchondral bony plate destruction, although it has largely been replaced with more conventional radiographic methods. Arthrography helps document proper aspiration of a suspected septic joint. Dye should be injected only after fluid is obtained from the joint because the bactericidal effect of iodinated contrast material can cause a false-negative culture result. CT can help determine the extent of medullary involvement. Pus within the medullary cavity replaces the marrow fat, causing an increased density on the CT scan. Adjacent soft tissue abscesses also are seen easily (Fig. 20-3). CT diagnosis of acute osteomyelitis is based on detection of intraosseous gas, osteolysis, soft tissue masses, abscesses, or foreign bodies. Additionally, increased vascularity after administration of a contrast agent also can aid in the diagnosis. Narrowing of the medullary cavity by granulation tissue and new bone is readily shown during the healing phase of osteomyelitis. CT identifies sequestra in chronic osteomyelitis (Fig. 20-4). It also is helpful in identifying alterations in areas poorly seen on plain films, such as the sternoclavicular joint, sacroiliac joint, and spine. Contrast material can be used to delineate abscesses in necrotic tissue that does not enhance from surrounding hyperemic tissue.
A major disadvantage of three-phase 99mTc phosphate bone scintigraphy is that the increased uptake caused by osteomyelitis is difficult to distinguish from that caused by degenerative joint disease or posttraumatic or postsurgical changes. The relative activity in each of the three phases may be helpful in differentiating other causes of increased uptake. Cellulitis causes increased activity during the flow and equilibrium phases and a decreased or normal uptake in the delayed phase. Osteomyelitis causes increased uptake in all three phases (Fig. 20-5). Increased uptake in the delayed phase but not in the flow or equilibrium phase suggests degenerative joint disease (Table 20-5). 99mTc phosphate bone scans are unreliable in neonates (<6 weeks old) and usually are negative in 60% of these patients with bone or joint infections.