32 Follow-up Monitoring of Cardiac Implantable Electronic Devices
Cardiovascular implantable electronic devices (CIEDs) include cardiac pacemakers, implantable cardioverter-defibrillators (ICDs), and leadless devices such as implantable loop recorders and cardiovascular monitors. Implantation is indicated for treatment, diagnosis, and monitoring of bradycardia, tachycardia, and most recently, heart failure.1 Since their inception in 1958, the function of CIEDs and monitoring of patients have become more complex. Functional roles may overlap, and capabilities for ventricular tachyarrhythmia therapy, biventricular stimulation, and collection of heart failure monitoring data may be contained in a single device. Positive results from recent trials for prophylactic ICDs and cardiac resynchronization therapy (CRT) devices have driven an expansion of indications and spurred an exponential increase in CIED use. An estimated 1 million devices were implanted in 2007, and this number will increase as their range and versatility increase.2
Purpose of CIED Monitoring
The aims of monitoring are to (1) establish and maintain appropriate CIED function, (2) optimize its programming, (3) identify risks in implanted device (e.g., impending lead failure) or from changes in patient condition (e.g., advent of AF), (4) monitor response to therapy, and (5) provide a communication channel for systematic data review. Current practice generally follows an in-clinic follow-up protocol by physicians and specially trained staff with retrieval of stored diagnostic data. For ICDs and CRT devices, this is performed at short intervals because of safety concerns (e.g., 3 monthly), although optimal frequency has not been tested.2
Significant challenges are increasing volume of patients, complexity of both CIED and patient, maintaining continuous surveillance, capability of prompt problem detection, and management of retrieved data.3,4 These place increasing demands on the health care system.
In-Person Monitoring
In-person monitoring with a physician or allied professional in a clinic or medical institution has been the conventional standard for device follow-up. Face-to-face evaluation permits history taking, physical examination, electrocardiography, and radiography as indicated. Interrogation of the device is performed using a programmer for bidirectional communication with the CIED. This has been traditionally wand-based but more recently, with wireless telemetry using a programmer located within 10 feet (3 m). Device function, including capture and sensing thresholds, may be reviewed (Table 32-1). Response to medical therapy may be disclosed, such as for atrial fibrillation (AF). Programmed settings and functions may be reprogrammed if necessary to optimize device operation and individualize parameters according to patient need (“actionable” encounters). The frequency of this may depend on the indication for the encounter; a routine scheduled check differs from unscheduled encounters to follow a particular condition or specific symptoms (Box 32-1).
In-person evaluation is required after implantation and before hospital discharge, for wound checks within 2 weeks, and then again at 6 to 12 weeks to set chronic parameters for pacemakers, ICDs and CRT devices. This period is significant because system-related problems (e.g., threshold increase, perforation, need for revision) tend to cluster in the early postoperative period.5,6
Subsequent follow-up schedules vary according to facility, physician preference, and available resources. Therefore, guidelines based on consensus opinion were issued by the Heart Rhythm Society (HRS) and European Heart Rhythm Association (EHRA). This consensus advocated a minimal recommended schedule of device follow-up of 3 to 6 monthly clinic checks for ICDs and 6 to 12 monthly checks for pacemakers, but with increased frequency (e.g., monthly) in response to product advisories and recalls.2 Such scheduled checks represent an estimated 4 million encounters in the United States alone. Problem discovery rates with this protocol have been unknown until recently.7 The incidence of unscheduled checks occurring with this schedule—an added burden to already-saturated device clinics—is also undetermined. The occurrence of significant cardiac or arrhythmic symptoms (e.g., shock therapy, palpitations) or detection of a CIED alert (e.g., audible alert) traditionally has required emergent clinic attendance and CIED interrogation. In the mid-2000s, a succession of advisories demanding higher frequency of follow-up overwhelmed clinic follow-up capacity, motivating the development and application of remote monitoring technologies.3
Remote Monitoring
Technologic Advances
Transtelephonic Monitoring Without Interrogation
Historically, remote monitoring of pacemakers has occurred for decades using transtelephonic monitors with modem technology. This system converts electrocardiographic information into sound to communicate over telephone lines to a decoding machine, which changes the sound back into the “rhythm strip.” This was introduced in the early 1970s to monitor the longevity of pacemakers8 and implemented for remote surveillance of sensing and capture as well as lead and device malfunction. It permitted frequent monitoring of pacing rate, determination of the underlying rhythm, and timely detection of battery depletion. Although extensively used, transtelephonic monitoring (TTM) was restricted to pacemakers, depended on active patient participation (and thus vulnerable to adherence issues), and delivered only a “snapshot” of the cardiac rhythm that was likely to miss intermittent problems. Indeed, in a recent trial, only 3 of 190 events were found on TTM, with discovery of the remainder requiring (delayed) inpatient assessment.9
Modern Systems
Remote monitoring systems permit access to the extensive data recorded in diagnostic memory of current-generation CIEDs. Each system is proprietary and works only with compatible devices from the same manufacturer: Home Monitoring (HM; Biotronik, Berlin), CareLink Network (Medtronic, Minneapolis), Latitude Patient Management System (Boston Scientific, St. Paul, Minn.), and HouseCall Plus (St. Jude Medical, Sylmar, Calif.). Their characteristics are summarized in Table 32-2. All technologies from a single manufacturer may not be available in all countries. CareLink may employ wand-operated or wireless transmitters. HM has been most extensively studied,5,10,11 in contrast to CareLink, although this is widely used. Latitude and Merlin (St. Jude Medical) have no published data regarding their technical or clinical utility. Mode of operation and level of patient involvement during transmission vary among monitoring systems. Operating differences pertain to the frequency of data transmission and report generation, type of reports generated, sensor technologies, and degree of patient involvement required for successful transmissions. For example, a high level of patient interaction is required by wand-based systems (e.g., CareLink), but virtually none by automatic systems (e.g., HM). Most remote monitoring systems transmit data via standard telephone lines, whereas HM uses a fully mobile, wandless, cellular-network transmitter that can be worn on the belt or carried in a purse to deliver automatic, daily device interrogations and alert reports (Fig. 32-1).
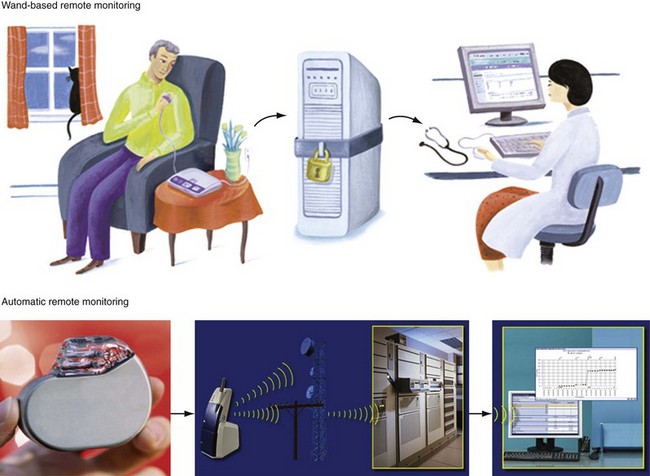
Figure 32-1 Contrasting remote monitoring technologies.
Top, Patient-activated system from home. Bottom, Example of automatic remote home monitoring by Biotronik Home Monitoring (HM). HM transmits data daily at a preset time, and immediately in response to a critical event. Transmission steps are as follows. A very-low-power radiofrequency transmitter circuitry is integrated within the pulse generator. The implanted device wirelessly transmits stored data on a daily basis to a mobile transceiver (typically placed at bedside at night). The transceiver automatically and silently accepts patient data from the implant and transfers this digital information using a cellular short-messaging system. The data are relayed wirelessly or via landline (automatically seeking first path available) to a dedicated service center. The service center generates a customized summary with informative trends, charts, parameters, electrograms, and graphs, available to the physician online via secure Internet access. Thus, daily patient monitoring may occur between scheduled office device interrogations providing trended information (e.g., battery status, lead impedance, sensing function). Service center processing and data upload on the HM webpage is automatic, bypassing potential delays (and errors) associated with manual processing. Data are stored in service center repository and analyzed and disseminated electronically. Critical and clinically relevant status changes data may be transmitted immediately without the need for patient interaction and flagged for attention on the HM webpage (see Fig. 32-2). Automatic alerts occur for silent but potentially dangerous events (e.g., device/lead failure, onset of asymptomatic AF). Event details include transmission of intracardiac electrograms similar to those available during office device interrogations. Physicians may be notified of alert conditions via e-mail or fax, if required. Useful information about the most important device or patient status changes can be delivered to the physician as quickly as a few minutes after the event. HM uses a triple-band and a quad-band exterior wireless transceiver connected to available worldwide cellular telephone networks (>55 countries).
(Modified from Varma N: Rationale and design of a prospective study of the efficacy of a remote monitoring system used in implantable cardioverter defibrillator follow-up: the Lumos-T Reduces Routine Office Device Follow-Up Study (TRUST). Am Heart J 154:1029-1034, 2007.)
An important potential of remote monitoring systems is the ability to perform early detection, especially of asymptomatic events, although systems vary in this ability. Clinically important events may be considerably delayed with systems relying on patient activation but, in contrast, can be notified within minutes by HM.12 However, remote manual testing or reprogramming of devices, although possible from an engineering perspective, is not available because of safety and security concerns. Actionable events, even if straightforward and easily resolved with simple reprogramming, demand in-person assessment.
Patient-Activated Remote Monitoring
These versions require patient-driven communication, whereby wand-derived data are relayed via telephone connections to following facilities (see Fig. 32-1). This requires the patient at home to manipulate a programmer and coordinate with clinic personnel to ensure communication and transmission, typically on a formal, calendar-based schedule. Early data from these systems reported technical feasibility and patient acceptance.13,14 Data transfer was similar to in-person interrogation. Nevertheless, these systems are cumbersome to use (patients reported difficulty in manipulating the wand,14 raising compliance issues) and time-consuming. As a tool, they essentially substituted for scheduled calendar-based in-person encounters, with the lack of monitoring in interim periods risking overlook of interim asymptomatic events. Therefore, although superior to TTM,9 patient-activated systems may not provide an efficient method of follow up.
Automatic Remote Monitoring
Current-generation remote monitoring systems implement an automatic transmission mechanism fully independent of patient or physician interaction. This relies on a CIED-initiated remote transmission using a Medical Implant Communication Service (402-405 MHz) or Industrial, Scientific and Medical (902-928 MHz) radiofrequency band allocated for implanted medical devices. An encrypted telemetric signal is sent from the CIED to a transceiver. The only requirement is that the patient be within a distance of approximately 6 feet (~2 m) from the transceiver. The transceiver (which may be a mobile unit with HM) uses telephone links, which may be landline and/or cellular based (see Fig. 32-1). The system can be programmed to download data at specific times and dates, usually when the patient is sleeping adjacent to the base unit. The transceiver automatically connects the phone link for data transfer. The treating physician can manually access the information and preprogram a customized set of alerts for each patient according to need (Fig. 32-2). If triggered, these alerts are transmitted promptly for physician review (color-coded according to urgency), enabling prompt clinical intervention if necessary. The amount and quality of transmitted information, including real-time intracardiac data, continue to improve and have reached the level of an in-office interrogation.
Automatic remote monitoring was pioneered by Biotronik (Home Monitoring, HM) and has been used in more than 50 countries (U.S. FDA approval in 200215). Other manufacturers are following (Table 32-2). This technology allows for automatic, patient-independent, wireless transmission of diagnostic ICD, CRT, and pacemaker data (see Fig. 32-1). Published data for technical efficacy for automatic remote monitoring are available only for the HM system.12,16 Reliability and early notification ability of this communication system were excellent. The wireless transmission ability is especially useful since almost 20% of U.S. households are currently estimated to have no landline facility.17 Greater than 90% of transmissions were received in less than 5 minutes (Fig. 32-3), and data integrity was 100% preserved.5,10,16,18 HM system operation is not energy costly and not susceptible to electromagnetic interference. The HM transceiver is a fully-mobile cellular unit. The novel quad-band transceiver is compatible with all major Global System for Mobile (GSM) cellular networks worldwide. It permits a unique callback function so that the medical professional can contact the patient worldwide via the transceiver, which is especially useful for travelers. Thus, automatic HM has the ability to maintain surveillance and rapidly bring to attention significant data, enabling clinically appropriate intervention.
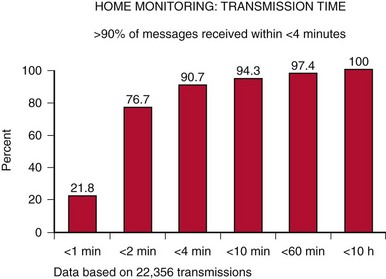
Figure 32-3 Transmission time with automatic remote monitoring.
Current Home Monitoring (HM) units are able to provide transmission speeds of less than 30 seconds.
(From Varma N, Stambler B, Chun S: Detection of atrial fibrillation by implanted devices with wireless data transmission capability. Pacing Clin Electrophysiol 28(suppl 1):133-136, 2005.)
TRUST Trial
The current variety of follow-up practices, with or without the assistance of different remote technologies, contrasts with a paucity of data to support any particular method or schedule. Although guidelines advocate 3 to 6 monthly clinic checks, the efficacy of this schedule with regard to patient safety, adherence, incidence of unscheduled encounters, and rate of problem detection remain untested. The TRUST (Lumos-T Safely Reduces Routine Office Device Follow-up) trial was undertaken to resolve some of these outstanding questions in a heart failure population receiving ICDs.19 TRUST has been the only prospective multicenter clinical study to assess and compare conventional follow-up and remote monitoring. The automatic remote monitoring mechanism tested was Biotronik Home Monitoring (HM).
Representing U.S. implant recipient demographics, 1516 patients enrolled at 105 U.S. sites in a predominantly community-based setting. Patients were randomized to conventional care (HM off) or to remote monitoring (HM on) groups (Fig. 32-4). Eligible patients were enrolled from 0 to 45 days after successful device implantation and then randomly assigned in a 2 : 1 ratio to HM or conventional groups, respectively. All patients had scheduled postimplant follow-ups at 3, 6, 9, 12, and 15 months, with unscheduled (interim) evaluations as needed. Patients assigned to conventional care were evaluated in-clinic only. In the HM arm, patients had HM checks followed by office visits at 3 and 15 months. At 6, 9, and 12 months and for interim visits, cumulated transmission files were remotely retrieved from the secure website and evaluated. Thus, both groups adhered to 3-month follow-up. Investigators were permitted to evaluate patients additionally in-office following HM checks during the study. Between these periodic checks, early notification could automatically occur for compromised system integrity (battery, lead parameters, high-voltage circuitry) or arrhythmia occurrence (e.g., AF, ventricular arrhythmia). These were evaluated online and patients brought in for office visits if deemed necessary by the investigator. Scheduled and unscheduled clinic visits (including responses to HM event notifications) were tracked for each individual in both study arms. Unscheduled interrogations in interim periods resulted from physician or patient initiation. TRUST’s primary objectives were to compare total in-hospital device evaluations in HM compared to conventional care and to assess the safety of the remote follow-up method.
Home monitoring reduced total (scheduled and unscheduled) hospital encounters for device interrogation by 45% (Fig. 32-5).20 The number of in person encounters occurring at scheduled 3-month intervals was reduced by 60%. This was not a 75% reduction, expected if all 6-, 9-, and 12-month checks were performed online, because the protocol permitted reviewing physicians to follow online check with in-person evaluations if deemed necessary. However, the small number of such cases indicated physicians’ confidence in the extent and quality of transmitted data, even at a stage of gaining familiarity with a new technology. Thus, more than 85% of all HM-group 6-, 9-, and 12-month follow-ups were performed using HM only, indicating that HM provided sufficient assessment in these patients. HM maintained better continuity of follow-up compared with traditional methods. A small proportion of the total number of in-person evaluations also resulted from unscheduled encounters. This is an important observation: a concern exists in the implanting community that an automatic remote monitoring system that self-screens daily would result in an increased presentation of patients to the hospital. However, this did not occur. Extension of face-to-face encounters to yearly in remote monitoring was not accompanied by loss of safety (see Fig. 32-5). The incidence of death and stroke trended toward improvement with use of HM.
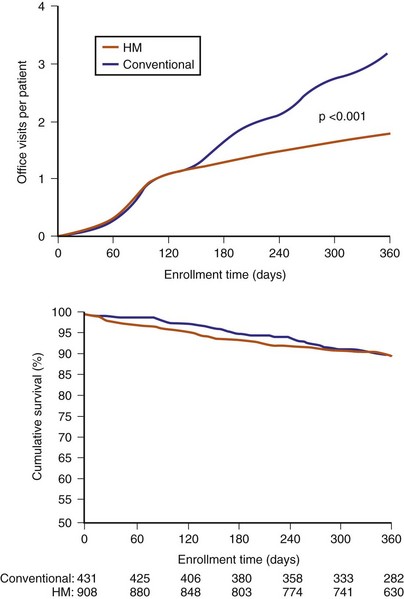
Figure 32-5 TRUST results.
(Compiled from Varma N, Epstein A, Irimpen A, et al: Efficacy and safety of automatic remote monitoring for ICD follow-up: the TRUST Trial. Circulation 122:325-332, 2010.)
TRUST’s secondary endpoint assessed the early-detection capability of HM, defined by time elapsed from event onset to physician evaluation. Evaluation in HM occurred on receipt of event notifications in response to detection of preprogrammed events or in-office interrogation (scheduled or unscheduled). In conventional care, event detection occurred at in-office interrogation (scheduled or unscheduled). HM enhanced problem discovery (even if asymptomatic) despite less frequent hospital evaluations. Detection was advanced by more than 30 days compared to conventional care20 (Fig. 32-6). (With 6 monthly scheduled conventional visits, HM would provide a correspondingly greater advance notification of at least 3-4 months.) Notably, this was not a test of transmission time (a technologic feature) but of “time to physician evaluation” (i.e., of clinical practice).
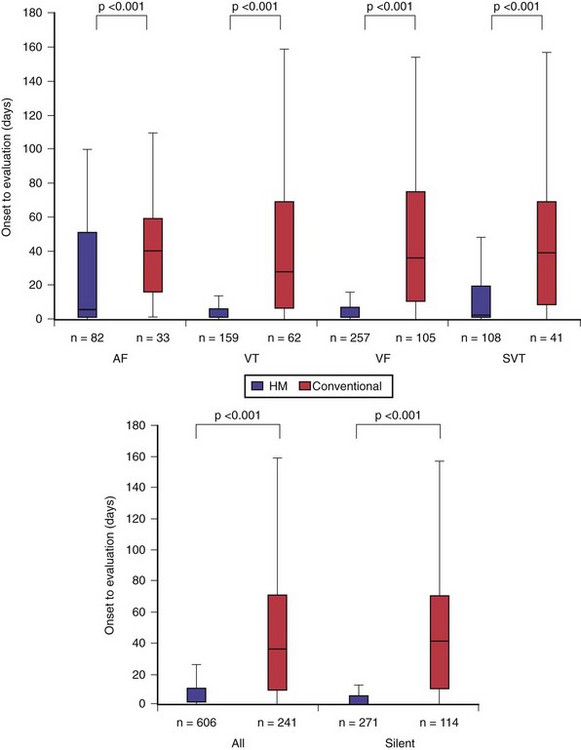
Figure 32-6 Early detection with home monitoring (TRUST secondary endpoints).
HM secured earlier physician evaluation of arrhythmias (top) and of silent events (bottom).
(Compiled from Varma N, Epstein A, Irimpen A, et al: Efficacy and safety of automatic remote monitoring for ICD follow-up: the TRUST Trial. Circulation 122:325-332, 2010.)
Another study, Clinical Evaluation of Remote Notification to Reduce Time to Clinical Decision (CONNECT), applied a separate automatic technology from a different manufacturer to a similar patient group.21 It reported broadly similar effects on total in-person evaluations, endorsing the results of TRUST. A significant shortening of hospital length of stay was observed as well, possibly because of a positive impact on patients’ health. Overall hospital costs were reduced by use of remote technology. However, only a minority of attempted alerts were transmitted successfully, severely limiting the utility of this proprietary technology for early detection.
In summary, TRUST demonstrated significant limitations of previously untested “conventional care” while, in contrast, showing the safety, efficacy, and early-warning capability of automatic remote monitoring. Automatic HM ensured follow-up continuity of a large patient volume, avoiding unnecessary in-hospital patient evaluation (thus reducing clinic load by almost 50%), but maintained near-continuous surveillance to rapidly identify problems. The trial results promote a paradigm shift in current clinical practice to exception-based hospital care.22