CHAPTER 100 Failed Disc Replacement
Despite a more than 20-year history of total disc replacements worldwide, detailed analyses of results and complications from comprehensive, prospective randomized studies have only become available through the U.S. investigational device exemption (IDE) trials.1–5 Box 100–1 is a comprehensive list of reported failures to date organized by mode/type of failure. Note that Box 100–1 does not include the myriad of approach-related complications including dysphagia, esophageal perforation, vocal cord paralysis, carotid artery injuries, retrograde ejaculation, ureteral injury, or large vessel injury. These complications are not unique to total disc replacements. They are inherent to anterior spinal surgery in general and have been reviewed elsewhere.6–8 The discussion that follows will review selected failures and focus on the prevention, evaluation, and management of these problems.
Failures of Lumbar Disc Replacement
Prevention of Failures
Benjamin Franklin’s profound words, “an ounce of prevention is worth a pound of cure,” cannot be overstated when discussing failures of the lumbar TDR. With current TDR designs, the lumbar spine is approached anteriorly for numerous reasons. These include (1) minimal morbidity allowing for short recovery times; (2) unobstructed visualization of the disc space, allowing for total discectomy and accurate implant sizing; (3) the absence of the need to enter or retract posterior neural structures in the spinal canal; and (4) the familiarity of territory for many spine and vascular surgeons. Accordingly, the approach-related risks are both predictable and relatively low.1–10 In contrast, revision approaches to the anterior lumbar spine have about a six times higher risk for major bleeding or thromboembolic complications due to adhesion formation, which prevents accurate identification of the great vessels.10 The anterior lumbar spine therefore remains a relatively facile approach as an index procedure but is fraught with potential complications in any revision situation. In our experience, many failures of lumbar disc replacement could have been avoided because they can be traced to surgeon-specific factors (as opposed to patient-specific factors) such as incomplete discectomy, improper device insertion, or inappropriate indications.
Total Discectomy
Rauschning has demonstrated that the bone at the periphery of the vertebral body endplate is strongest and all TDR implants have been designed to be seated along this peripheral rim of bone.11 Performing a complete or radical discectomy therefore becomes paramount for accurate total disc sizing and endplate placement. Implants that are too anterior or do not achieve good “rim fit” are more prone to dislodge or subside. A fundamental impediment to complete discectomy is poor visualization of the disc space. Regan and colleagues12 have demonstrated the importance of the “learning curve” for optimal outcomes for CHARITÉ TDR. Access surgeons and spinal surgeons who are uncomfortable or unfamiliar with the anterior approach to the lumbar spine will be less likely to retract the great vessels far enough to permit complete exposure of the lateral aspect of the disc. In contrast to lumbar interbody fusion, a relatively larger window into the intervertebral disc space is required to properly perform total disc replacement. We routinely use handheld Hohmann retractors, which pierce the vertebral bodies above and below the disc. Large Steinmann pins can serve this purpose as well. This technique of retraction prevents the iliac vessels and vena cava from gradually creeping into the surgical field, thus narrowing the exposure of the intervertebral disc. If one prefers to use fixed table–based retractor systems, then it is wise to continuously assess and reassess the field of exposure to ensure that adequate visibility is maintained to assist complete disc space preparation.
Sizing, Lordosis, and Placement
Maintaining lumbar lordosis is intuitive in terms of spinal balance in the sagittal profile; however, with lumbar disc replacement, especially L5-S1, maintaining and matching the patient’s lordosis is imperative to reduce shear and achieve good endplate contact, thereby reducing the risk of anterior dislodgement or dislocation. If the disc space lordosis measures 15 degrees, for example, but the prosthetic lordosis only reaches a maximum of 10 degrees, one or both of the prosthetic endplates will not be in complete contact with the host vertebral endplate. Assuming the prosthesis can match the 15 degrees of lordosis, it is even more favorable to have a mechanism by which the articulating portion of the prosthesis can assume a more horizontal (parallel to the floor when standing) position. One way to achieve this is to “build up” the caudal prosthetic endplate with a 10-degree or more lordotic endplate. By “horizontalizing” the disc space, the magnitude of the shear forces at the articulating surfaces is reduced but will still be significant at the caudal bone-implant interface. A note of caution might be in order for patients who have an especially steep sacral inclination of the S1 endplate. In conjunction with these concerns, L5-S1 presents with additional and theoretical risks for postoperative spondylolisthesis. The subject of facet orientation introduces the idea that if the facets are more in the sagittal plane rather than the coronal plane, there is a predisposition of future destabilization over time and increase in facet stress in flexion and extension. This has not been directly studied due to the limitation of cadaveric specimens with their respective facet orientation. Additionally, as Rousseau and colleagues showed in a comparison of constrained and semiconstrained disc arthroplasties with intact specimens, facet forces vary during flexion-extension and lateral bending. He found that one implant tended to unload the facets in extension and to a lesser degree in lateral bending, which was attributed to the implant’s instantaneous axis of rotation. The other tended to increase in these areas. Also, the flexion forces on the facets were not statistically different than for the intact specimen with a potted sacral inclination of 60 degrees.13
In the first U.S. IDE to reach completion, McAfee and colleagues3 demonstrated that inaccurate positioning of the lumbar TDR was statistically correlated with reduced patient outcome.
Appropriate patient selection may be the most important factor in reducing the risk of failures. Strict adherence to the inclusion and exclusion criteria from the U.S. IDE trials such as patients with osteoporosis, metabolic bone disease, history of chronic steroid use, or age older than 60 years should reduce the risk of TDR endplate subsidence. Failure to recognize an isthmic spondylolisthesis is another known reason for failure (Fig. 100–1). In the U.S. CHARITÉ trial there were five unrecognized pars defects for fractures that became apparent at the 6-week follow-up visit.10 In four of the five cases the defect was appreciable after retrospective scrutiny of the preoperative flexion and extension images was performed. One such case demonstrated migration of the device requiring posterior pedicle screw instrumentation and fusion. In the fifth case, bilateral pedicle fractures occurred in the postoperative setting and this case was revised to an anterior interbody fusion with posterior pedicle screw-rod instrumentation and fusion. While patients with a degenerative spondylolisthesis of less than 3 mm are not excluded from U.S. IDE trials, we prefer not to perform TDR on patients with more than 2 degrees of intervertebral translation. Computed tomography (CT) is the study of choice to evaluate the presence of an isthmic spondylolisthesis when the diagnosis is in question on preoperative flexion-extension radiographs or magnetic resonance imaging (MRI). CT scans will also reveal the degree of facet arthrosis better than MRI, which might also exclude certain candidates from lumbar TDR.
Failures (see Box 100–1)
Implant failures such as polyethylene core fractures, core dislocations, or implant breakage have been uncommon occurrences thus far. Our knowledge of them derives from case reports or small case series.14–17 Instances of excessive polyethylene wear have been quite rare and have uniformly occurred in patients implanted before 1997. David reported a case of polyethylene failure due to oxidation 9.5 years following implantation.14 Similarly, as reported in the largest series of TDR failures reported to date, there was one case of detectable polyethylene core wear noted 12 years postoperatively.17 Since 1997, an industry-wide enhancement to the sterilization process of polyethylene, whereby irradiation occurs in an inert gas such as nitrogen rather than air, has resulted in dramatically improved wear characteristics due to a reduction in the incidence of oxidation. This corrective preventative action of avoiding polymerization and reducing the UHMWPE wear rate was born out of the total joint implant experience on partially cross-linked UHMWPE. In contrast to the previously mentioned cases, analysis of a retrieved polyethylene core from a revision surgery performed for bone-implant loosening 1.6 years postoperatively in a patient implanted post-1997 demonstrated low levels of oxidation with mechanical properties that were not substantially degraded.18 At the time of this writing, there are only two published cases of anterior dislocation of the polyethylene inlay of a ProDisc artificial disc replacement.16 A case of posterior core dislocation presented to our clinic (Fig. 100–2). There are no reported cases of metal endplate fatigue failures or deformation for modern prosthetic designs.
Bone-implant failures account for the greatest number of failures of lumbar disc replacement, and the mode of failure depends on the type of device. Sagittal vertebral body fractures can occur with keeled devices because the keel slot creates a stress riser in the bone (Fig. 100–3).19 Two-level implantations in which keel slots are introduced into the superior and inferior aspects of the intercalated vertebra are at highest risk for this complication. To reduce this risk, the MAVERICK keel has been reduced from 11 mm to 7 mm.20 In contrast, implants with smaller “anchors” at the bone-implant interface such as the CHARITÉ, in which six 3-mm teeth engage the vertebral endplate, exhibit a greater tendency to migrate or dislodge if improperly placed. In the CHARITÉ U.S. IDE study, there were 15 of 347 implantations that required removal and none of these had been inserted in the “ideal” position.3 Regardless of the design, TDR placement anterior to the center of rotation, especially in a hyperlordotic segment, will tend to migrate or dislocate anteriorly due to excessive shear.21 As previously mentioned, spondylolysis and spondylolisthesis present a biomechanically less stable environment for TDR and explain some of the early failures by migration (Fig. 100–4). Damage to the vertebral endplate during insertion, placement of a TDR in an osteoporotic spine, inaccurate positioning, and insertion of an implant that is too small are all factors that can contribute to TDR subsidence. In van Ooij and colleagues’17 report of 27 complications of the CHARITÉ device, there were 16 cases of subsidence. The cause, prevalence, or incidence of these failures could not be determined because the study was retrospective without mention of the total number of cases. Strict adherence to indications, surgeon education, and modern insertion instrumentation systems should theoretically reduce the incidence of this complication.
Iatrogenic deformity has a greater tendency to occur in multilevel implantations (Fig. 100–5). Preoperative scoliosis is a relative contraindication to TDR; however, subtle coronal and sagittal-plane deformities may not be taken into account. TDR insertions in these scenarios will likely exacerbate rather than reduce any deformity because stabilizing structures such as the anterior and posterior longitudinal ligament, as well as the majority of the annulus, are removed during the implantation. Even in the well-aligned spine, incomplete discectomy and “off axis” TDR placement at one level can create an “off-axis” situation at the next level (Fig. 100–6). Thus when more than one vertebral level is to be implanted, device placement errors will tend to be compounded at sequential levels of insertion, resulting in a “Z deformity.” Kyphosis is not prevalent following TDR unless the implant is inserted backwards (i.e., a lordotic device inserted in kyphosis) or severe subsidence of the implant into the posterior half of the vertebral body.
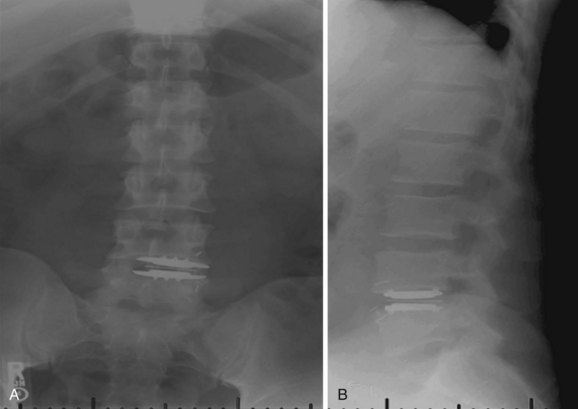
FIGURE 100–6 A and B, CHARITÉ disc replacement placed at an outside facility with off-axis placement.
Host response to particulate debris generated from virtually any articulating biomaterial is characterized by macrophage recruitment and proinflammatory cytokine release. The culmination of this cascade is matrix metalloprotease activation, which leads to bone resorption and osteolysis. Osteolysis is a well-documented complication of total joint replacement22,23; however, most investigators agree that it will not be prevalent following TDR. One reason for the potentially reduced risk in the spine is the fact that the intervertebral disc lacks a surrounding synovial space, the key source for macrophages and other inflammatory cells. The second reason is the relatively reduced range of motion and hence reduced volumetric wear in TDR compared with typical diarthrodial joint replacement. With more than 20 years of global experience and more than 10,000 TDR implantations worldwide, there are only anecdotal reports of osteolysis, and to our knowledge only one has been documented histologically.10 Heterotopic ossification (HO) surrounding lumbar TDR implants has been classified previously by McAfee and colleagues24 in preparation for the CHARITÉ U.S. IDE trial. In this trial, the incidence of HO was 4.3%; however, the presence of HO did not have any impact on flexion-extension range of motion or clinical outcome.25 We know of no other lumbar TDR trial in which the presence of HO has been systematically evaluated; however, isolated cases of periannular ossifications have been reported. To be fair, however, the imaging characteristics of the lumbar devices, which are typically cobalt-chrome or stainless steel alloys, do not allow for identification of all but the larger amounts of periannular bone formation.
Neurologic injury has not been shown to be more prevalent in cases of lumbar TDR when compared with fusion controls in prospective randomized evaluation.26 Most cases of revision surgery in the CHARITÉ U.S. IDE trial involved reoperation with laminectomy and foraminotomy for new neurologic symptoms in the lower extremities. After any anterior discectomy and instrumentation, patients should be asked if they have any “new” pain in their legs that was not present preoperatively and a thorough neurologic examination should be performed in the postanesthesia care unit. If there are any new neurologic complaints or physical examination findings (i.e., motor or sensory loss), a CT scan with myelographic contrast should be performed to assess positioning of the implant and ensure that a hematoma, bone fragment, or disc material is not present in the spinal canal. MRI is not particularly helpful postimplantation due to metal artifact obscuring the regions of greatest interest. By rapidly diagnosing the cause for new neurologic symptoms with appropriate imaging, early revision such as implant repositioning is possible. Neurologic injury without radiographic abnormalities is a complication that typically presents with left-sided leg pain in the L4 or L5 dermatome. First reported by Thalgott and colleagues, this complication has been thought by some to be due to excessive retraction of the lumbar plexus during the retroperitoneal exposure.27 The lumbar plexus is at highest risk when exposing the L4-5 disc space. To mobilize the left common iliac vein to the patient’s right side, the iliolumbar vein(s) needs to be identified and ligated as it courses dorsally between the lumbar nerve roots located under the psoas muscle (Fig. 100–7). Alternatively, such symptoms have been thought to be sympathetic mediated and result from the vascular exposure because similar symptoms may be seen following nonspinal vascular dissections. In either case, whatever the etiology, the dysesthetic pain pattern typically resolves after 6 to 8 weeks; however, corticosteroid selective nerve root blocks may help minimize the symptoms. Notwithstanding, the burden of proof remains to demonstrate that the acquired symptoms are not due to new nerve root compression, which may be remedied with further surgery.