22 Essentials of Neurology and Neuromuscular Disorders
General Considerations
The term neurologic disorder encompasses a wide variety of conditions that may have extremely mild or very serious effects (Table 22-1).1–5 These disorders are likely to be associated with a degree of physical, cognitive, or a combined disability. Many children with severe physical disabilities have normal intelligence and are competent to make decisions about treatment options. Those who are mildly cognitively impaired may wish to be consulted about treatment choices; adolescents in particular must be involved in decision making.6 This approach is especially important in managing individuals with chronic disorders who are accustomed to thinking about health issues and who often have strong and well-defined opinions about how they wish to be treated.7
Condition | Prevalence | Reference |
---|---|---|
Cerebral palsy: all types | 2.2 per 1000 live births | 1 |
Epilepsy | 5-10 per 1000 all ages | 2 |
Central nervous system tumors | 1-5 per 1000 all ages | 3 |
Neuromuscular disorders (all ages) | 1 per 2900 total population | 4 |
Congenital myopathy | 1 per 28,600 | |
Duchenne muscular dystrophy | 1 per 3500 | |
Myotonic dystrophy | 1 per 12,000 | |
Limb girdle dystrophy | 1 per 90,000 | |
Spinal muscular atrophy | 1 per 74,000 | |
Mitochondrial disorders | 11.5 per 100,000 all ages | 5 |
When planning anesthesia for these individuals, physicians must become knowledgeable about them and their conditions. Assumptions should not be made about their level of comprehension or about how they and their parents view the choices available.8 It was formerly acceptable practice for children with chronic neurologic disorders to be excluded from the full range of therapeutic options, but it is now essential that all options are included.9
Parents of children with chronic disorders of all types are usually accustomed to dealing with health care situations and often have thought carefully about the implications of various treatments. They know the child best and are usually most qualified to make decisions by proxy. Widespread use of the Internet has assisted many parents in becoming knowledgeable about their child’s condition and about potential interventions.10 Their aims are usually entirely appropriate, and this knowledge may assist physicians in their collaboration with children and parents in determining optimal management. Occasionally, the information has been obtained from an unreliable source and may be inaccurate or inappropriate for a particular child.11 Misinformation can precipitate difficult situations for professionals, especially when there is a perceived disparity between the desires of the parents and those of the child or what the clinician considers to be in the child’s best interest. The surgical team members usually are most involved in the process of obtaining informed consent, but the anesthesiologist must participate in the dialogue because anesthesia may be the part of treatment that carries the greatest risk.
Children with neurologic disorders usually have a regular physician overseeing their care, and this person should participate in the decision-making process. For elective procedures, the surgeon should establish contact with the child’s regular pediatric specialist and anesthesiologist as soon as surgery is contemplated, inform them about the proposed operation, and seek their opinions to optimize perioperative management. Cognitive, communication, and behavioral problems; coexisting diseases; and drug therapy that may influence management of the anesthesia for these patients should be evaluated at an early stage.12 Some children may be receiving long-term respiratory support, including cough assist devices and home ventilation, and all current therapies should be considered.
Oral communication may be difficult for some children with neurologic disorders. Use of age-appropriate assisted communication devices may help to ensure open communication between physician and patient.12 Parents’ opinions and attempts to protect their children should be respected and understood as a consequence of previous experiences, stress, frustration, anger, and probably guilt.13 Each procedure has to be assessed in terms of morbidity, mortality, and probability of improving quality of life. All aspects must be clearly and objectively discussed with parents or guardians and informed consent forms signed.
Static Neurologic Disorders
Cerebral Palsy
Cerebral palsy is a disorder of movement, muscle tone, or posture that is caused by injury or abnormal development of the immature brain. It has a prevalence of 1 case per 500 live births.14 Eighty percent of cases are acquired prenatally and have no obvious cause, although they are associated with prematurity low birth weight, intrauterine growth retardation, intracranial hemorrhage, and trauma. Birth complications, including asphyxia, are estimated to account for another 6% of cases. Postnatal causes of cerebral palsy (10%) usually arise from infectious causes (e.g., bacterial meningitis, viral encephalitis), trauma (e.g., motor vehicle collisions, falls, child abuse), or metabolic disturbance (e.g., hyperbilirubinemia).15
Classification depends on the severity, distribution, and thenature of the motor deficit. With the advent of modern imaging techniques, including magnetic resonance imaging (MRI), understanding the pathogenesis of cerebral palsy has advanced dramatically.16–18 Clinical features are classified most commonly according to the type of motor deficit, its distribution, and the severity of the deficit (Table 22-2). Involvement of a single limb is referred to as monoparesis, of both limbs on one side of the body as hemiparesis, of both lower limbs as diparesis, of three limbs as triparesis, and of all four limbs as tetraparesis (i.e., quadriparesis). The motor deficit may manifest as hypotonia, spasticity, or extrapyramidal features such as choreoathetoid/dystonic movements or ataxia. A descriptive classification includes neurologic deficit and distribution (e.g., spastic diparesis, dystonic hemiparesis).
Children with cerebral palsy require neuroimaging to confirm the diagnosis and underlying cause of the condition. Although some centers use oral sedation (i.e., midazolam or chloral hydrate) for neuroimaging, many children require general anesthesia. They may require multiple anesthesias throughout their lifetimes because of the associated comorbidities (i.e. respiratory, gastrointestinal, neuromuscular, and orthopedic), common surgical conditions, and problems unique to cerebral palsy that require treatment.19
Multisystem Comorbidities
Most children with cerebral palsy have clinically significant oromotor dysfunction, and when associated with gastroesophageal reflux, it may lead to recurrent aspiration, decreased respiratory reserve, esophageal stenosis, and malnutrition.20 Frequently employed procedures include fundoplication, gastrostomy, and esophageal dilation treatment.12 Immobility, underhydration, and poor diet predispose patients to bowel stasis and constipation, which may become severe, with fecal impaction occurring occasionally. Malnutrition may depress immune responses, and electrolyte imbalance and anemia are common. Preoperative assessment of these parameters is essential.
Pulmonary complications are common causes of death in cerebral palsy. Aspiration associated with gastroesophageal reflux is the leading cause, and it may be exacerbated by excessive oral secretions, bulbar dysfunction, recurrent respiratory infection, and chronic lung disease.21,22 Scoliosis may also restrict pulmonary function, with cardiopulmonary involvement depending on the curve pattern and the severity of the curve (see Chapter 30).12
Orthopedic operations are the most frequently performed procedures in children with cerebral palsy.12 Procedures include tendon releases to ease contractures, femoral osteotomy, and hip adductor and iliopsoas releases.23 The trend in orthopedic surgery is to perform multiple procedures involving tenotomies or osteotomies at different levels of all extremities during a single general anesthesia, rather than staging them during multiple operations.12,19 Scoliosis often requires surgery to prevent further deterioration in lung function and to stabilize the spine to facilitate ambulation and sitting. Spinal fusion is considered in all children with progressive curves greater than 40 to 50 degrees.24
Approximately 30% of children with cerebral palsy have epilepsy. It is more common in spastic hemiplegia and less common in the ataxic and choreoathetotic forms. Generalized and focal seizures frequently occur. Anticonvulsants should be maintained until the surgery date (given the morning of surgery) and restarted as soon as possible in the postoperative period.12,25
Anesthesia Considerations for Cerebral Palsy
The many multisystem comorbidities and therapies specific to children with cerebral palsy must be understood to minimize perioperative complications. Risk factors include an inability to walk, severe neurologic deficit, major cognitive dysfunction, severe scoliosis, malnutrition, and the presence of a gastrostomy or tracheostomy.19 Severely compromised children can be optimally managed postoperatively with admission to the pediatric intensive care unit to provide analgesia with comprehensive monitoring, maximum support, and aggressive respiratory care, and after they are stabilized, they can be transferred to a setting with less intense monitoring.
Most of these children have above-average intelligence. They have the same emotional and cognitive concerns as others about undergoing anesthesia, including preoperative anxiety that may require premedication. Children with contractures, especially in the upper extremities, may present a challenge for establishing intravenous access. If gastroesophageal reflux is not controlled, consideration should be given to rapid-sequence induction. Although these children have a neuromuscular disorder, intravenous succinylcholine yields only a normal release of potassium,26 despite evidence of proliferation of extrajunctional acetylcholine receptors.27 Maintenance of and emergence from anesthesia requires special considerations, including the possibility of a reduced minimal alveolar concentration (MAC),28 resistance to neuromuscular blocking agents,29 and reduced bispectral index (BIS) measurements.30 If vomiting is likely to occur, the airway must be protected.
These children have normal responses to pain, which should be managed as if they were unaffected by cerebral palsy. Caudal or epidural analgesia may be a reasonable approach for perioperative pain management if the child does not have a ventricular-peritoneal shunt. Management and assessment of perioperative pain in children with neurocognitive impairment is addressed in Chapter 43.
Malformations of the Nervous System
Malformations are common in pediatric neurologic practice and a frequent cause of early mortality. The appearance of the neural plate shows that the central nervous system (CNS) develops very rapidly in the 2-week embryo and continues until several years after birth. The cause of CNS malformations is largely uncertain, but timing appears to be more important than the nature of the insult in producing the specific type of malformation. Causative agents include maternal drugs such as sodium valproate, which is associated with neural tube defects (NTDs); infections such as cytomegalovirus, which can cause various cerebral lesions, depending on the time in gestation of the infection; toxins such as alcohol; vitamin deficiency (e.g., folic acid), and genetic disorders. Historically, because diagnostic investigation was limited, postmortem examination was required to demonstrate the neuropathologic changes causing the clinical disorder. MRI now can provide adequate images to enable a diagnosis in many instances (e.g., cortical dysplasia).31
Neural Tube Defects: Cranial and Spinal Dysraphism
The cause of NTDs is multifactorial, with genetic and environmental factors being the most important. Approximately 10% of NTDs are caused by chromosomal abnormalities such as trisomies (i.e., 18, 13, and 21), triploidy, and Turner syndrome. Preconceptual folic acid supplementation has reduced the prevalence of NTDs by 30% to 50%.32 Along with antenatal ultrasound examination, screening is done for increased maternal serum levels of α-fetoprotein, reduced human chorionic gonadotropin levels, and reduced unconjugated estriol levels; termination of pregnancy in cases that test positive has further reduced the prevalence of NTDs.33
Anencephaly is a lethal disorder that results from failure of the neural tube to form. This leads to disorganization of neural elements and the absence of skull formation.31 Some deep cerebral structures may remain intact, and the brainstem may develop normally. With the latter, normal respiration and cardiovascular functions may develop, enabling the infant to survive for hours or days after birth. Other structures in the head and brain, including the eyes, face, and pituitary gland, may not develop normally.
Encephalocele is a herniation of neural tissue and meninges out of the skull through deficient skin and bone (see Fig. 24-11, B). They are frequently associated with other cerebral malformations, such as agenesis of the corpus callosum. Encephaloceles found anteriorly are associated with underlying brain, orbital structures, or pituitary gland anomaly. Posteriorly, encephaloceles are associated with cerebral or cerebellar tissue that herniates through a bony defect in the posterior cranium. Intranasal encephaloceles may be difficult to detect. These defects carry a poor prognosis for long-term survival. Most infants die, and in survivors, severe neurodevelopmental disability is common. Most of these children have hydrocephalus.34
Spina bifida refers to a group of conditions in which there is abnormal or incomplete formation of the midline structures over the back (see Fig. 24-11, A, in Chapter 24).31 Skin, bony, and neural elements may be involved singly or in combination. Congenital malformations of the spinal cord may exist in isolation or in association with brain anomalies. These defects may present at birth, as in the case of the more severe and open lesions (i.e., spina bifida) or be identified later in childhood if the skin overlying the spinal defect is intact (e.g., spina bifida occulta). Those who develop a Chiari malformation may present with cervical cord or bulbar deficits, placing them at risk for respiratory embarrassment (see Figs. 24-12 and 24-13). Children with spinal cord lesions are at increased risk for sensory deficits, making meticulous skin care and positioning essential to prevent pressure sores and damage to neuropathic joints.
When the defect is identified at birth, it is optimally managed in a specialist center by a multidisciplinary team (i.e., pediatrician, neurologist, neurosurgeon, orthopedic surgeon, and others) who can anticipate, prevent, and treat complications and assist in the child’s long-term care. Children with dysraphism often develop postoperative hydrocephalus because of disrupted CSF flow and require a ventricular-peritoneal shunt. Long-term complications, including paraparesis, neurogenic bladder and bowel, renal insufficiency, trophic limb changes, pressure sores, joint contractures, and scoliosis, may require surgical repair and future intervention. These children are also at risk for latex allergy. From the outset, all children with NTDs should be considered latex sensitive and undergo management in a latex-safe environment. The anesthesia considerations for NTDs are presented in Chapter 24.
Chiari Malformation
Chiari malformations of the nervous system may coexist with other anomalies and manifest in the neonatal period or later in the early decades of life (Table 22-3).
Syringomyelia
Syringomyelia results from a glial cell–lined cavitation within the spinal cord. Diagnosis has been greatly simplified by the use of MRI, which provides images of the spinal cord and the tubular fluid-filled space within.31 The pathogenesis of syringomyelia remains unclear. It complicates several conditions, such as rare familial cases, congenital malformations, trauma, and meningeal infection. It has been reported as a coincidental finding in normal individuals.
Hydrocephalus
Hydrocephalus results from overproduction or impaired drainage of CSF from the brain.34 In practice, overproduction is an uncommon source of hydrocephalus; these cases most often result from tumors of the choroid plexus. Obstructed CSF drainage is the far more common basis. Causes of hydrocephalus include intraventricular hemorrhage, Arnold-Chiari malformation, brain tumor, congenital obstruction, and myelomeningocele.
Surgical treatment for hydrocephalus involves insertion of a drainage system to shunt CSF from the brain to another site in the body (see Fig. 24-10). The anesthesia considerations for treating hydrocephalus are discussed in Chapter 24.
Disorders of Ventral Induction
Holoprosencephaly is a cephalic disorder in which the forebrain of the embryo fails to develop into discrete hemispheres with normal connections.31 There are three types:
1. Lobar: There is almost complete separation of the hemispheres, and the corpus callosum is almost absent.
2. Semilobar: The two hemispheres are divided posteriorly, with interhemispheric connections present anteriorly. The corpus callosum is absent anteriorly, and the thalami are fused in the midline.
3. Alobar: An undivided and small forebrain with a dorsal sac may contain some cortex. Severe facial defects may include cyclopia (i.e., single orbit with fused globes), cebocephaly (i.e., single nostril), and a midline cleft lip.
Disorders of Cortical Development
Malformations of the cerebral cortex are many and varied. Although uncommon, development of lissencephaly or agyria (i.e., smooth cortex), pachygyria (i.e., thickened cortex), and polymicrogyria (i.e., multiple, small gyri) depends on the stage of embryogenesis affected.31 MRI has advanced the identification of these features and their classification.
Progressive Neurologic Disorders
Primary Brain Tumors
The incidence of primary brain tumors is 2.6 per 100,000 children, and they account for more than 20% of all childhood malignancies. One third of these tumors occur before 5 years of age, and 75% occur before 10 years of age.35 Two thirds are located infratentorially and one third supratentorially (Table 22-4).36
Pathologic classification, which is based on the cell of origin and degree of malignancy, extends from grade I (benign) to grade 4 (malignant).37 The patient’s presentation depends on the site of the tumor. Infants typically display irritability, failure to thrive, and macrocephaly, whereas older children develop headache, nausea, vomiting, seizures, gait disturbances, and visual deficits. If the lesion is rapidly expanding and is accompanied by significant cerebral edema or obstructs CSF drainage, the intracranial pressure (ICP) will increase. Occasionally, hemorrhage may occur into the tumor, causing a dramatic progression of signs and symptoms, mandating emergency treatment. Ultimately, brainstem decompensation and death ensue if the lesion is not treated.
The 5-year survival rate of greater than 60% for primary brain tumors largely reflects improved imaging, aggressive surgery, and evidence-based therapy.38 Unfortunately, children who survive CNS tumors frequently have permanent neurologic deficits, including epilepsy, learning disabilities, visual or hearing impairment, and growth and endocrine disorders. Short-term and long-term follow-up evaluations by specialist teams are required, along with careful emotional and social support for children and their families. Some children have genetic predispositions for CNS tumors, such as neurofibromatosis (i.e., schwannomas of the spinal cord, peripheral nerve tumors, skeletal deformities, carcinoid syndrome, and multiple endocrine neoplasia, including pheochromocytoma) and tuberous sclerosis (i.e., brain tumors, cardiac rhabdomyomas, renal abnormalities, and hepatoma), and they require genetic analysis and long-term follow-up.
Tumors of the spinal cord are rare in childhood. They may be benign or malignant and sited within the cord (intramedullary) or outside (extramedullary). Symptoms and signs may initially be nonspecific and vague, especially in young children. This may delay the diagnosis and increase the risk of spinal cord compression. Delayed relief of compression may cause vascular compromise, which may lead to total and irreversible paralysis of the limbs, bladder, and bowel and permanent, severe disability. Diagnosis is best made by MRI of the cord, which provides details of the lesion and adjacent structures without the risk of further decompensation, a problem raised by the use of myelography in the past.36
Metabolic Disease
These diseases may cause a static encephalopathy but more often produce a progressive course with loss of physical and intellectual skills. Epilepsy, especially myoclonus, is common, as is loss of vision, neuropathy, deafness, or involvement of other organ systems (particularly cardiac). Some neurometabolic diseases are associated with intellectual deficits and some with physical deficits; systemic features may be prominent, and neurologic signs are common (Table 22-5).
TABLE 22-5 Neurometabolic Disorders
There are three main groups of neurometabolic diseases39:
1. Those with a known enzymatic defect, including disorders of amino acid metabolism (e.g., phenylketonuria), peroxisomal disorders (e.g., adrenoleukodystrophy), and lysosomal storage disorders (e.g., Tay-Sachs disease)
2. Those with abnormal storage accumulation in CNS cells, including lysosomal storage disorders and mucopolysaccharidoses
3. Those with no identified biochemical defect (e.g., Cockayne syndrome), a heterogeneous group that is shrinking as research identifies the biochemical defects
Disorders that cause lactic acidosis are the most common of these diseases, although many individuals who harbor the genetic substrate for one of these disorders may not express it or may come to diagnosis very late in life.40 The features of these diseases are extremely varied, and although the neuropathologic, biochemical, and imaging abnormalities are well recognized, a precise diagnosis may remain elusive.
Neuromuscular Disorders
Neuromuscular disorders are caused by an abnormality of any component of the lower motor neuron system: anterior horn cell in the spinal cord, axon, neuromuscular junction, or muscle fiber (Fig. 22-1).41 The cardinal features are weakness of skeletal muscles that is proximal, distal, or generalized in distribution, hypotonia, and reduced deep tendon reflexes. True fatigability suggests a defect of the neuromuscular junction. Neuropathy is characterized by distal weakness and sensory deficit. Joint contractures, scoliosis, and respiratory and cardiac involvement are common complications, and some conditions are associated with cognitive deficits.
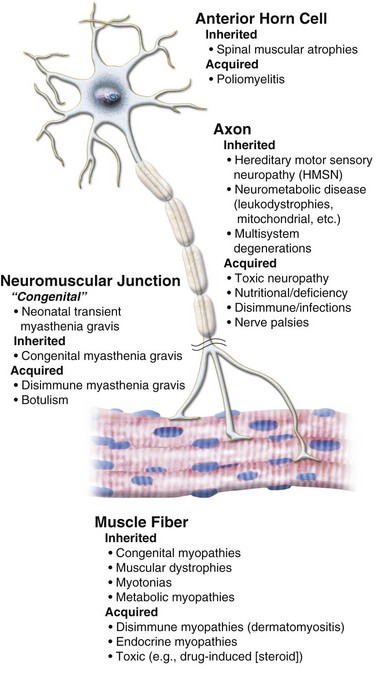
FIGURE 22-1 Diagram of a lower motor neuron.
(Modified from Dubowitz V. Muscle disorders in childhood. Philadelphia: WB Saunders; 1978. Courtesy A. Moosa, MD.)
Disorders of the Anterior Horn Cell
Spinal Muscular Atrophies
The spinal muscular atrophies (SMAs) are a group of disorders in which there is progressive degeneration of the anterior horns of the spinal cord and death of motor neurons. They are inherited as an autosomal recessive trait. The type of SMA (I, II, or III) is determined by the age of onset and the severity of symptoms.41 The diagnosis is a clinical one, but confirmation by molecular testing for the survival of motor neuron gene (SMN) in a blood sample is now possible, and electrophysiology and muscle biopsy are no longer necessary.42 Curative treatment is not available, but much can be done to improve duration and quality of life, especially for mildly affected children.
Type I SMA (i.e., Werdnig-Hoffmann disease) manifests at or soon after birth in most cases. The infant may appear neurologically normal at first, but the typical picture soon emerges. Parents may first notice an abnormal breathing pattern as the intercostal muscles are affected, and the respiratory pattern becomes diaphragmatic, with a bell-shaped chest observed clinically and radiographically. The infant is usually very alert and interactive but severely weak and floppy. There is good facial expression and normal eye movements, but the tongue fasciculates, and the tendon reflexes are absent. There is no cardiac involvement. Weakness, hypotonia, and bulbar involvement lead to progressive respiratory insufficiency and swallowing dysfunction, which are frequently complicated by episodes of aspiration. Most children die within the first 2 years of life, mainly due to respiratory complications. Management is essentially palliative, with gentle physiotherapy to keep the limbs flexible and special care with feeding. Noninvasive nocturnal ventilation is increasingly used, but invasive ventilation through a tracheostomy is not considered appropriate in most medical centers. This issue has provoked considerable debate in the literature.43
Type II SMA is an intermediate form, and it is the most prevalent. Because the weakness is milder, many children survive for years with meticulous multidisciplinary therapy, orthopedic and respiratory management, and care with nutrition. Onset occurs at 6 to 18 months of age. The clinical signs are similar to those of type I SMA, and the children are bright, intelligent, and particularly verbal. Type II patients usually achieve independent sitting at some stage, but these children never bear weight or walk. Respiratory infections are a particular problem, and noninvasive nocturnal respiratory support (i.e., biphasic or continuous positive airway pressure) with a facemask and portable ventilator is well tolerated by children and parents. This improves well-being and enables remarkably full activity despite weakness.44,45
Some children with type II SMA develop feeding difficulties because of weakness of bulbar musculature or as a complication of chronic nocturnal hypoventilation. Good nutrition is essential for health, and supplementation and gastrostomy feeding may be required.46
Type III SMA (i.e., Kugelberg-Welander disease) is a mild variant with similar signs of flaccid and areflexic weakness of lower more so than upper limbs and with proximal predominance. These children attain independent walking, although it may be later than normal and tenuous. There is often deterioration around the time of puberty, when the growth spurt causes the previously precariously balanced muscle groups to become dysfunctional. Obesity and joint contracture also may affect the situation. Aggressive measures to keep the child walking are often effective and delay or prevent scoliosis and lower limb contracture.47 Respiratory support may be necessary for nocturnal hypoventilation. Prognosis for long-term survival is good.
Other SMA variants exist in childhood. A rare type (SMA with respiratory disease) has severe diaphragmatic involvement and respiratory failure in infancy.48