25 Essentials of Endocrinology
Diabetes Mellitus*
The incidence of both type 1 and type 2 diabetes mellitus in children is increasing worldwide.1–6 The use of insulin pumps and various multicomponent insulin regimens has increased the complexity of perioperative management of children with diabetes. Anesthesiologists must carefully consider the pathophysiology of the disease, as well as each child’s specific diabetes treatment regimen, glycemic control, intended surgery, and anticipated postoperative course, when devising an appropriate perioperative management plan. Standardized algorithms for perioperative diabetes management improve care7–9 without significantly increasing costs9; several guidelines and studies of perioperative management of children with diabetes are available in the literature and examples are included.10–15
Classification and Epidemiology in Children
Type 1 and type 2 are the most common forms of diabetes.16,17 Type 1 diabetes is characterized by absolute deficiency of insulin, which usually results from immune-mediated destruction of pancreatic beta cells.17 In contrast, type 2 diabetes results from a combination of insulin resistance and a relative deficiency of insulin.16,17 Children with type 2 diabetes typically are overweight and frequently have a first- or second-degree relative with type 2 diabetes.16 However, the increasing prevalence of obesity in children18 has made distinction between type 1 and type 2 diabetes, at times, rather difficult. Children with phenotypic characteristics of type 2 diabetes may have pancreatic autoimmunity,19–21 and approximately 35% of children with diabetes who require exogenous insulin at diagnosis are overweight or obese,22,23 consistent with the prevalence of childhood overweight and obesity in the general U.S. pediatric population.24 Other forms of diabetes may be less commonly encountered (Table 25-1). Additional modifications to the perioperative treatment regimen may be necessary when diabetes is associated with genetic syndromes and/or other endocrinopathies, such as adrenal insufficiency (see later discussion).
TABLE 25-1 Classification of Less Common Forms of Diabetes Mellitus
Modified from Rhodes ET, Ferrari LR, Wolfsdorf JI. Perioperative management of pediatric surgical patients with diabetes mellitus. Anesth Analg 2005;101:986-99.
Although the worldwide incidence of type 1 diabetes is quite variable,25 the incidence is increasing in almost all populations throughout the world.4 In the United States, the SEARCH for Diabetes in Youth Study, which began in 2000, provides the most comprehensive estimates of the prevalence and incidence of type 1 and type 2 diabetes among youth less than 20 years of age in the United States.26–30 Type 1 diabetes remains the most common form of diabetes observed among youth in the United States, with the greatest prevalence (2 per 1000) among non-Hispanic Caucasians.29 The epidemic of obesity has also contributed to a progressive increase in the incidence and prevalence of type 2 diabetes in U.S. children.1 In the SEARCH study, the prevalence of type 2 diabetes in 10- to 19-year-old youth ranged from 0.18 per 1000 among non-Hispanic Caucasian youth to 1.45 per 1000 among Navajo youth, and the incidence ranged from 3.7 per 100,000 per year among non-Hispanic Caucasian youth to 27.7 per 100,000 per year among Navajo youth.29,30 Increases in the incidence and prevalence of type 2 diabetes have been noted in other parts of the world.2,3,5,6,31–34
General Management Principles
Type 1 diabetes always requires treatment with insulin. However, an increasing number of insulin preparations (Table 25-2) and delivery systems are available. The least complex insulin regimens consist of two or three insulin injections per day. They incorporate a combination of an intermediate-acting insulin (e.g., neutral protamine Hagedorn [NPH]) and/or a long-acting insulin (e.g., insulin detemir [Levemir] or insulin glargine [Lantus]) for basal coverage with a short- or rapid-acting insulin (e.g., regular, insulin aspart [NovoLog], insulin lispro [Humalog], or insulin glulisine [Apidra]) to provide prandial glycemic coverage. More intensive insulin regimens are being used with greater frequency and typically consist of insulin glargine, a long-acting insulin, which provides a relatively constant 24-hour basal concentration of circulating insulin without a pronounced peak, to simulate basal insulin secretion,35 in conjunction with rapid-acting insulin administered with food. Some studies have demonstrated superior glycemic control with such regimens as compared with regimens using NPH insulin and regular insulin,36 although the ideal insulin regimen for children with type 1 diabetes remains controversial.37 Another long-acting insulin, insulin detemir, may also be used in intensive insulin regimens and has demonstrated more predictable glucose-reducing effects than both NPH insulin38–40 and insulin glargine.38
Many children with type 1 diabetes are managed with an insulin pump,41,42 a device that administers a continuous subcutaneous infusion of insulin (typically a rapid-acting insulin, see Table 25-2) at a basal rate that is supplemented by bolus doses of insulin given with meals and snacks and to correct hyperglycemia. In appropriately selected children, pump therapy has shown superiority over injection regimens.43–46 Standard insulin preparations are U100, meaning that there are 100 units of insulin per milliliter. However, very young patients with type 1 diabetes may require diluted insulin (e.g., U10) to achieve accurate dosing.47 Parents of toddlers with type 1 diabetes should be specifically questioned about their use of diluted insulin.
Most children with type 2 diabetes are managed with insulin and/or oral metformin, the only oral agent approved for use in children with diabetes in the United States.48–50 Metformin’s primary action is to decrease hepatic glucose production and, secondarily, to increase insulin sensitivity in peripheral tissues. Occasionally, other oral agents, including sulfonylureas, which promote insulin secretion, and thiazolidinediones, which increase insulin sensitivity in muscle and adipose tissue, are used in adolescents.48,51 Nutritional therapy is always included in the management of children with type 2 diabetes. Studies, such as the Treatment Options for Type 2 Diabetes in Adolescents and Youth (TODAY) trial,52,53 are evaluating the optimal treatment for type 2 diabetes. Monotherapy with metformin was associated with durable glycemic control in approximately half of children and adolescents with type 2 diabetes. The addition of rosiglitazone, but not an intensive lifestyle intervention, was superior to metformin alone.
New medications introduced to manage adults with diabetes may eventually become available for use in children. Incretins, including glucose-dependent insulinotropic polypeptide and glucagon-like peptide-1 (GLP-1), are gastrointestinal hormones released after eating that stimulate insulin secretion and are necessary for normal glucose tolerance.54,55 GLP-1 acts through a G protein–coupled receptor to promote glucose-dependent insulin secretion, suppression of glucagon secretion, slowing of gastric emptying, and reduction in food intake.54 Exenatide (Byetta) is a GLP-1 receptor agonist widely used in adults with type 2 diabetes as an adjunctive therapy for those taking metformin and/or a sulfonylurea.56 Inhibitors of dipeptidyl peptidase-IV,57 the enzyme that degrades GLP-1, are also increasingly being used for the treatment of type 2 diabetes in adults, but have not been approved for use in children. Pramlintide acetate (Symlin) is a synthetic amylin receptor agonist that can be used as an adjunct to insulin therapy in adults with type 1 or type 2 diabetes.58–61 Amylin, a 37-amino acid polypeptide islet hormone cosecreted with insulin from beta islet cells,54 has three effects: delays gastric emptying, inhibits glucagon secretion, and modulates satiety.54
Metabolic Response to Surgery
Trauma of any kind, and surgery in particular, causes a complex neuroendocrine stress response, including suppression of insulin secretion and increased production of counter-regulatory hormones (frequently called “stress hormones” in the anesthesiology literature), particularly cortisol and catecholamines.62,63 Insulin is the primary anabolic hormone that promotes glucose uptake in muscle and adipose tissue while suppressing glucose production (glycogenolysis and gluconeogenesis) by the liver.64 The counter-regulatory hormones, which include epinephrine, glucagon, cortisol, and growth hormone, exert the opposite effects, resulting in resistance to insulin action65–67 and an increase in blood glucose concentration by: (1) stimulating glycogenolysis and gluconeogenesis in the liver, (2) increasing lipolysis and ketogenesis, and (3) inhibiting glucose uptake and utilization in muscle and fat. Glucagon, secreted by alpha cells in the pancreatic islets, suppresses insulin secretion while stimulating hepatic glycogenolysis, gluconeogenesis, and ketogenesis.64,65 Epinephrine, which acts via β2– and α2-adrenergic receptors, stimulates glucagon production, increases glycogenolysis and gluconeogenesis, stimulates lipolysis, decreases insulin secretion, and decreases glucose utilization in insulin-sensitive tissues.64 Cortisol stimulates gluconeogenesis, proteolysis, and lipolysis, and decreases glucose utilization.65,68 Growth hormone augments glucose production, decreases glucose utilization, and accelerates lipolysis.69 Pro-inflammatory cytokines may further stimulate secretion of counter-regulatory hormones and alter insulin receptor signaling.67 These changes increase catabolism, as evidenced by increased hepatic glucose production and breakdown of protein and fat. In the diabetic patient with absolute or relative insulin deficiency, the enhanced catabolism resulting from surgical trauma can lead to marked hyperglycemia and even diabetic ketoacidosis.70 These metabolic effects may be exacerbated by a prolonged fast before and during surgery.
Metabolic Response to Anesthesia
Although adequate analgesia is essential to minimize the neuroendocrine stress response to surgery, some anesthetics may also independently contribute to perioperative hyperglycemia.10,71 Inhalation anesthetics, such as isoflurane, may cause hyperglycemia by inhibiting insulin secretion72,73; the hyperglycemia results from both impaired glucose uptake and increased glucose production.71 In contrast, epidural analgesia with local anesthetics prevents this hyperglycemic effect74,75 through an inhibitory effect on endogenous glucose production.71 Similarly, intravenous (IV) anesthesia with opioids mitigates the hyperglycemic response of surgery71,76,77 through its apparent neutral effect on endogenous glucose production but decrease in glucose clearance.71 Although these differences are important to consider, the metabolic effects of anesthesia are relatively minor compared with the direct effects of surgery.10
Adverse Consequences of Hyperglycemia
Hyperglycemia can impair wound healing by hindering collagen production, which may decrease the tensile strength of the surgical wound.78 Hyperglycemia may also have adverse effects on neutrophil function, including decreased chemotaxis, phagocytosis, and bactericidal killing.79–82 Evidence from controlled experimental studies in rabbits demonstrated that these effects may be reversed, in part, by glycemia-independent effects of insulin.83 However, the overall benefits of intensive insulin therapy, including a reduction in mortality, were derived mainly from maintenance of normoglycemia, whereas glycemia-independent actions of insulin exert only minor, organ-specific effects.83
Clinical studies in humans have not consistently supported the relationship between perioperative glycemic control and short-term risk of infection or morbidity.84,85 However, several studies in diabetic adults undergoing surgery have shown an association between postoperative hyperglycemia and infectious complications.86,87 A meta-analysis showed that patients in surgical intensive care units (ICUs) appear to benefit from intensive insulin therapy, whereas patients in other ICU settings do not.88 These outcomes have contributed to confusion regarding specific glycemic targets and the means for achieving them in both critically and noncritically ill patients. A recent consensus statement89 recommended that an insulin infusion should be used to control hyperglycemia in the majority of critically ill patients in the ICU setting, with a starting threshold of no greater than 180 mg/dL. Once IV insulin therapy has been initiated, the glucose level should be maintained between 140 and 180 mg/dL. With no prospective, randomized controlled trial data for establishing specific guidelines in noncritically ill patients treated with insulin, pre-meal glucose targets should generally be less than 140 mg/dL and random blood glucose values less than 180 mg/dL, as long as these targets can be safely achieved. To avoid hypoglycemia, one should reassess the insulin regimen if blood glucose levels decline below 100 mg/dL. Modification of the insulin regimen is necessary when blood glucose values are less than 70 mg/dL, unless the event is easily explained by other factors (such as a missed meal).89
The single pediatric trial to date demonstrated shorter length of stay, attenuated inflammatory response, and decreased mortality in patients randomized to targeting of age-adjusted normoglycemia.90 However, the rate of severe hypoglycemia (less than 40 mg/dL) in that study was too frequent, at 25% of the total cohort, making the implementation of this approach ill-advised without further evidence from ongoing multicenter pediatric trials. Other retrospective studies of critically ill children managed in pediatric ICUs have also demonstrated associations of hyperglycemia with morbidity and mortality.91–94 In the 1980s and 1990s the dogma of tight glucose control replaced an era in which the mantra of caring for diabetic surgical patients was “keep them sweet,” but in more recent years glucose control has become controversial and is unresolved, with conflicting evidence; a systematic review of the literature concluded that there are insufficient data regarding the best strategy or regimen to attain target blood glucose levels in ambulatory surgical patients with diabetes mellitus.95 The recommended practice for subspecialties, such as cardiac, neurosurgical, and solid organ transplant surgical patients, requires still more study, and it must be highlighted that few of the published investigations and meta-analyses have focused specifically on the pediatric age group.
Preoperative Assessment
When feasible, children with diabetes should not undergo elective surgery until they are metabolically stable (Fig. 25-1, box 4); that is, there is no ketosis, serum electrolytes are normal, and the glycated hemoglobin (HbA1c) value is close to or within the ideal range for the child’s age. Although there is no consensus regarding the ideal metabolic targets for control of diabetes in children,96 the American Diabetes Association recommends an HbA1c less than or equal to 8.5% for children younger than 6 years of age; less than 8% for children 6 to 12 years of age; and less than 7.5% for 13 years of age or older, with a more stringent target of less than 7% to be considered in this last age group, for children in whom this can be achieved without excessive hypoglycemia,96 as well as for those with type 2 diabetes.16 The preoperative consultation to assess the adequacy of metabolic control should be scheduled at least 10 days before the procedure (see Fig. 25-1, box 3). If metabolic control is poor, surgery should be delayed if possible. Both the endocrinology and anesthesiology services should participate in this assessment. Whenever possible, surgery for children with diabetes should be scheduled as the first case in the morning so that prolonged fasting is avoided and diabetes treatment regimens may be most easily adjusted.
Preoperative Management
The regimen for managing diabetes before, during, and after a surgical or diagnostic procedure that requires the child to fast should aim to maintain near-normoglycemia, that is, blood glucose levels of 100 to 200 mg/dL. In this blood glucose range there will be a reduced risk of osmotic diuresis, dehydration, electrolyte imbalance, metabolic acidosis, infection, and hypoglycemia in the sedated child who may be unaware of hypoglycemia or unable to communicate with staff.97 The child need not be admitted before the day of surgery, but rather early on the same morning as the surgery or procedure. Parents should receive explicit written instructions regarding appropriate modifications of their child’s diabetes regimen before and for the day of surgery. On admission to the hospital, metabolic control should be assessed, including a preoperative determination of glucose concentration. On the morning of surgery, no rapid- or short-acting acting insulin should be administered unless the blood glucose level exceeds 250 mg/dL. However, admission to the hospital before major surgical procedures in children10 and adults has been recommended if the metabolic status needs to be optimized preoperatively.65 If the surgery must be delayed for any reason, frequent blood glucose monitoring is mandatory to prevent perioperative hypoglycemia or hyperglycemia.
If the blood glucose exceeds 250 mg/dL, a conservative dose of rapid-acting insulin (e.g., insulin lispro or insulin aspart) or short-acting insulin (regular) is administered to restore near-normoglycemia. This is achieved using the child’s usual sliding scale or a “correction factor.” The insulin “correction factor” is the decrease in the blood glucose concentration expected after administering 1 unit of rapid- or short-acting insulin. This can be calculated using the “1500 rule”: divide 1500 by the child’s usual total daily dose (TDD) of insulin. For example, if a child typically receives 30 units of insulin daily, this child’s “correction factor” would be 1500 ÷ 30 = 50. Therefore 1 unit of rapid- or short-acting insulin would be expected to decrease the child’s blood glucose concentration by approximately 50 mg/dL. Various correction factors have been described, including a “1500 rule” for short-acting insulin (regular) and an “1800 rule” for rapid-acting insulin, such as insulin lispro.91,98 For simplicity and because of insulin resistance stimulated by surgical stress, the “1500 rule” is appropriate in this setting, even with the use of a rapid-acting insulin. To then calculate an appropriate corrective dose of insulin to restore near-normoglycemia, the anesthesiologist should aim for a target blood glucose concentration of 150 mg/dL.
More detailed preoperative recommendations must be based on the individual child’s usual treatment regimen. For most children with diabetes undergoing minor outpatient surgical procedures, insulin can be provided perioperatively with subcutaneous injections. In adults, especially those with type 2 diabetes, this practice is commonly,97 although not universally, preferred.99 Others routinely recommend insulin infusions even for minor outpatient surgical procedures in children.10 Several reports suggest that better glycemic control can be achieved in the perioperative period with a continuous intravenous infusion rather than subcutaneous insulin administration.12,100,101 However, these studies were conducted before the availability of rapid-acting insulins whose rapid and reproducible effects after subcutaneous administration102 may more closely match the ability to titrate IV-administered short-acting insulin. Subcutaneous insulin lispro has been shown to be as efficacious as regular IV insulin for treatment of uncomplicated diabetic ketoacidosis in children.103 Whatever the management strategy for the diabetes, it is most important that it is coordinated between the anesthesiology and endocrinology services, and the modifications to the child’s diabetic regimen at home needs to be communicated clearly and in a timely manner to the family.
Split-mixed insulin regimens involve two to three injections per day with a combination of NPH or insulin detemir plus a rapid- or short-acting insulin. For children who use such a regimen, 50% of the usual morning dose of NPH or the full usual dose of insulin detemir should be administered on the morning of the procedure (Fig. 25-2). For the child whose basal insulin is once-daily insulin glargine or insulin detemir, no additional insulin is given on the morning of the surgery if the child received a dose in the evening of the previous day (Fig. 25-3). However, if insulin glargine or detemir is typically administered in the morning, the full dose should be administered on the morning of the procedure to prevent ketosis.
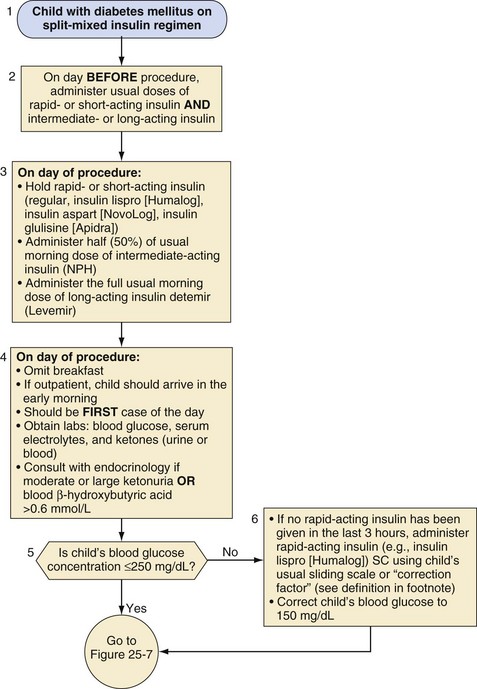
1. Divide 1500 by child’s total daily dose (TDD).
2. If daily dose varies (i.e., use of sliding scales), use the average daily dose in the past week to determine TDD.
3. Example: if TDD = 50 units, then insulin correction factor is 1 unit insulin lispro (Humalog) to reduce blood glucose by 30 mg/dL.
NPH, Neutral protamine Hagedorn; SC, subcutaneous.
(Modified from Rhodes ET, Ferrari LR, Wolfsdorf JI. Perioperative management of pediatric surgical patients with diabetes mellitus. Anesth Analg 2005;101:986-99.)
Management of the child on an insulin pump depends on the duration of the surgical procedure (Fig. 25-4). Those undergoing minor procedures expected to last less than 2 hours can continue to receive their usual basal rate via their insulin pump. However, this approach requires that the anesthesiologist is familiar and comfortable with the use of the pump in the operating room. Other protocols include transitioning patients who use an insulin pump to IV insulin infusion or subcutaneous insulin glargine.65 For procedures longer than 2 hours, children should be transitioned to an IV insulin infusion, as described in the next section.
Major Surgery and Intravenous Insulin Infusions
For children who require major surgery, especially procedures anticipated to last more than 2 hours, an IV insulin infusion is the preferred perioperative diabetes management plan (Fig. 25-5). Studies in children12 and adults7 have demonstrated that glycemic control is superior with infusions of IV insulin compared with subcutaneous injections. These children should receive their usual doses of insulin on the day before the procedure. On the morning of the procedure, an IV infusion of 5% dextrose in half-normal saline should be started at a maintenance rate, and an IV insulin infusion should also be provided to accommodate the dextrose infusion to maintain blood glucose in the target range of 100 to 200 mg/dL (see Fig. 25-5). The maintenance rate for IV fluids in a child depends on body size and can be calculated either based on body weight (4 mL/kg/hr for the first 10 kg body weight, 2 mL/kg/hr for 11 to 20 kg, and then 1 mL/kg/hr for each kg over 20 kg) or body surface area (1.5 L/m2/day). The insulin dose varies with the child’s pubertal status; prepubertal children are relatively more sensitive to insulin than pubertal adolescents.104 In prepubertal children with type 1 diabetes, after the remission (honeymoon) period, the insulin requirement is typically 0.6 to 0.8 unit/kg/day, whereas in adolescents, the requirement is 1 to 1.5 units/kg/day.105,106 Children with type 2 diabetes may require even greater doses of insulin because of their insulin resistance. With IV insulin, a suitable initial ratio of insulin to dextrose may be estimated from the child’s usual or average total daily insulin dose (TDD) using the formula 500 ÷ TDD. For example, if the child typically receives 50 units of insulin daily, then 500 ÷ 50 = 10. Therefore, in this example, 1 unit of insulin would be administered via a “piggyback infusion” for each 10 g of dextrose contained in the maintenance IV infusion to prevent hyperglycemia. Note that a maintenance IV solution of 5% dextrose contains 10 g of dextrose in every 200 mL. Only regular insulin should be used for IV infusions.
Special Considerations for Preoperative Management of Type 2 Diabetes
Children with type 2 diabetes may require insulin or one of several oral antihyperglycemic agents (Fig. 25-6). Metformin should be discontinued 24 hours before a procedure because of its long half-life and the risk of lactic acidosis in the presence of dehydration, hypoxemia, or poor tissue perfusion.107 Other oral agents, such as sulfonylureas and thiazolidinediones, may be discontinued on the morning of the procedure. Figure 25-6 outlines additional recommendations for children with type 2 diabetes who use a split-mixed or basal-bolus insulin regimen. Adjustments for other insulin regimens or oral hypoglycemic agents in type 2 diabetes should be determined in consultation with an endocrinologist or diabetologist.
Intraoperative Management
The insulin and fluid regimen during and after surgery depends on the duration of the procedure. If the procedure is likely to be brief (e.g., ≤1 hour) and one can reasonably anticipate that the child will be able to drink soon after the procedure, it may not be necessary to start a glucose-containing IV infusion. If the duration of fasting is likely to be more prolonged, an IV infusion should be started at a maintenance rate as described earlier (Fig. 25-7). Intraoperative maintenance fluid should then be replaced with a comparable glucose-containing solution. Replacement of insensible losses and intravascular volume owing to blood or other body fluid losses should be with an appropriate isotonic solution (e.g., lactated Ringer’s solution or normal saline).
Although some protocols include potassium chloride in the maintenance IV fluid solution,10,65,97 this practice should generally be avoided because of the danger of inadvertent intraoperative administration of large quantities of potassium during fluid resuscitation. Children undergoing a brief procedure with a baseline normal serum potassium concentration and well-controlled diabetes have a small risk of hypokalemia. Those undergoing more prolonged surgeries or emergent surgeries during which metabolic decompensation is more likely, require intraoperative assessment of electrolytes and appropriate adjustment of the electrolyte composition of their IV solution. In all cases, blood glucose concentrations should be measured hourly and either insulin or dextrose adjusted, as necessary, to maintain blood glucose in the target range of 100 to 200 mg/dL. If the blood glucose exceeds 250 mg/dL, urine or blood ketones should also be measured (see Fig. 25-7). Either an increase in the rate of continuous insulin infusion or subcutaneous administration of a rapid-acting insulin analog is used to correct intraoperative hyperglycemia. Intraoperative IV bolus of regular insulin is not recommended because this causes a rapid supraphysiologic increase in serum insulin concentration, which, owing to insulin’s short half-life (approximately 5 minutes), will have a short-lived effect on blood glucose levels. In contrast, subcutaneously administered rapid-acting insulin analogs have a typical and reproducible pharmacologic profile.
Postoperative Management
As soon as the child is able to resume drinking and eating normally, the usual diabetes regimen, including insulin and/or oral agents, may be reinstituted and the dextrose infusion discontinued, if applicable (Fig. 25-8). One exception to this approach is for children with type 2 diabetes who take metformin; metformin should be held for 48 hours and renal function must be within normal limits before its resumption. For children who are unable to eat or drink, IV dextrose and electrolyte solution should be continued until oral intake is restored. An infusion of IV short-acting insulin (regular) or intermittent subcutaneous rapid-acting insulin should be administered, not more than every 3 hours, to maintain blood glucose in the target range of 100 to 200 mg/dL. Frequent blood glucose monitoring and monitoring blood or urine ketones is essential because of the variable effects of surgical trauma, inactivity, pain, anxiety, nausea and/or vomiting with poor oral intake, medications, and postoperative infection. At the time of discharge from the hospital, children and their parents or care providers should be given appropriate guidelines regarding these issues. Those who are admitted to the hospital overnight after surgery should be managed in consultation with the endocrinology service, if possible, to coordinate appropriate scheduling and subsequent dosing of insulin.
Special Surgical Situations
Children with diabetes who need urgent surgery must have a full clinical and biochemical assessment. Frequently, the problem necessitating surgery may have led to metabolic decompensation that must first be corrected and stabilized, unless the need for surgery is immediate. These children are often dehydrated; in addition to administering insulin, rehydration and electrolyte replacement is critical to address their metabolic derangements and restore normal glomerular filtration rate and renal function. In most cases, these children require emergent surgery that should be managed with an IV infusion of insulin as described earlier (see Fig. 25-5). Children with diabetic ketoacidosis require close collaboration between the anesthesiology and endocrinology services.
Diabetes Insipidus
Diagnosis of Neurosurgical Diabetes Insipidus: The Triple-Phase Response
Of special interest is the triphasic pattern of vasopressin secretion, often, but not always, observed after neurosurgical procedures that interfere with the supraoptic-hypophyseal tract.108 After surgery, an initial phase of transient DI may be observed, lasting between 12 hours and 2 days. This may be explained by local edema that interferes with normal vasopressin secretion. If significant vasopressin-secreting cell damage has occurred, release of stored vasopressin from damaged neurons leads to a second phase that involves water retention. The syndrome of inappropriate antidiuretic hormone secretion (SIADH) may last up to 10 days. Finally, a third phase, permanent neurogenic DI, may follow if more than 90% of vasopressin cells are destroyed. Pronounced SIADH in the second phase generally portends permanent DI in the final phase of the triple response. In children with vasopressin and cortisol deficiency (e.g., in combined anterior and posterior hypopituitarism after neurosurgical treatment of craniopharyngioma), symptoms of DI may be masked because cortisol deficiency impairs renal free water clearance. Institution of glucocorticoid therapy may precipitate polyuria, leading to the diagnosis of DI (Fig. 25-9).
Perioperative Management of Minor Procedures
Children with preexisting DI who are scheduled for a minor procedure, i.e., a procedure without significant blood loss and not followed by a period of further fasting or fluid shifts (e.g., myringotomy, radiologic imaging, peripheral orthopedic procedures) are treated differently from those undergoing a more major procedure associated with blood loss or fluid shifts and delayed postoperative resumption of fluid intake (see Fig. 25-9).
Children undergoing minor procedures under anesthesia should receive their usual morning dose of desmopressin (DDAVP) (Table 25-3). The anesthetic technique is tailored to the procedure (e.g., IV sedation for MRI, general endotracheal anesthesia for tonsillectomy). Arterial and urinary catheters are not required, and recovery takes place in the postanesthesia care unit (PACU). Once the morning dose of desmopressin has been administered, intraoperative and postoperative fluids should be restricted to the rate of 1 L/m2/24 hours, i.e., to match insensible free water losses and obligatory urine output. Oral fluids may be offered once the child is awake. Although modern PACU policies often no longer require successful fluid intake before discharging the ambulatory patient, discharging the child with DI should be delayed until the child is able to freely take oral fluids without vomiting. Subsequent doses of desmopressin should be administered according to the child’s usual preoperative schedule.
TABLE 25-3 Medications for Management of Central Diabetes Insipidus
Desmopressin acetate (100-, 200-µg tablets; nasal tube 10 µg/0.1 mL; nasal spray 10 µg/0.1 mL: delivers 0.1 mL/spray) | |
Oral | Dose: 100-400 µg q12h |
Oral doses are 10-20 times intranasal doses of desmopressin | |
Onset of action: ~15-30 minutes | |
Duration: 8-12 hours | |
May develop tachyphylaxis | |
Intranasal | Dose: ~10-20 µg/dose q12-24h |
Onset of action: 5-15 minutes | |
Duration: 8-12 hours | |
Vasopressin (Pitressin) (20 units/mL; 0.5-mL, 1-mL, and 10-mL vials; conversion: 1 unit = 2.5 µg) | |
Intravenous | Dose: 1.5 milliunits/kg/hr |
Onset of action: minutes | |
Half life: 5-10 minutes |
Perioperative Management of Major Procedures
A major surgical procedure is defined as an operation associated with the potential for significant blood loss; intraoperative or postoperative hemodynamic, neurologic, or respiratory instability; entry into a body cavity (e.g., craniotomy, abdominal surgery, thoracic surgery); craniofacial or airway surgery; major orthopedic surgery (e.g., spine surgery, tumor resection or amputation, major osteotomies); and/or surgery followed by delayed resumption of unrestricted fluid intake. The child with DI should be scheduled as the first case of the day. On the day before the procedure, the child treated with desmopressin should receive the usual morning dose, but only 50% of the usual evening or bedtime dose (see Fig. 25-9). On the morning of the procedure, desmopressin is withheld. The child undergoing a major procedure should receive general anesthesia with conventional monitoring, as well as insertion of arterial and urinary catheters, and after surgery is admitted to the ICU. A central venous catheter for monitoring central venous pressure, although not indicated solely for the management of DI, is useful in the postoperative period.