33 Electromagnetic Interference and CIEDs
Electromagnetic Compatibility
International standards have been developed establishing the upper limit of permissible field intensities and protocols established to test CIEDs against possible interference. Regulatory agencies in the United States established recommendations to test for EMI in CIEDs.1 Draft recommendations are also available for guidance in the use of wireless technology.2
Sources of Electromagnetic Interference
Sources of EMI can be classified according to type and spectral frequency of energy emitted, as well as the environment in which the source is encountered (Box 33-1). For clinical purposes, it is useful to recognize radiated and conducted sources of EMI.
Box 33-1
Documented Sources Of Electromagnetic Interference (Emi)
Electromagnetic Fields
Radiated EMI
Radiated EMI can result from energy emitted for communication purposes or as an unintended effect of other electrical activity (e.g., motor operation in an electric razor). Electromagnetic fields have both an electric field, measured in volts per meter (V/m), and a magnetic field. The magnetic flux density is measured in milliteslas (mT). Another common way to characterize an electromagnetic field is by the power density, or power per unit area. Power density can be expressed in milliwatts (mW) or microwatts (µW) per square centimeter (cm2). The unit used to measure how much radiofrequency (RF) energy is actually absorbed by the body is the specific absorption rate (SAR); it is usually expressed in watts per kilogram (W/kg) or milliwatts per gram (mW/g). Electromagnetic sources, for the purpose of examining medical device interactions, may be broadly divided into RF waves, with frequencies between 0 Hz and 450 MHz (e.g., electric power, radio/television transmitters, electrosurgical units, EAS systems), and microwaves, between 450 MHz and 12 GHz (e.g., radar transmitters, cellular telephones, two-way phones, microwave ovens) (Fig. 33-1).
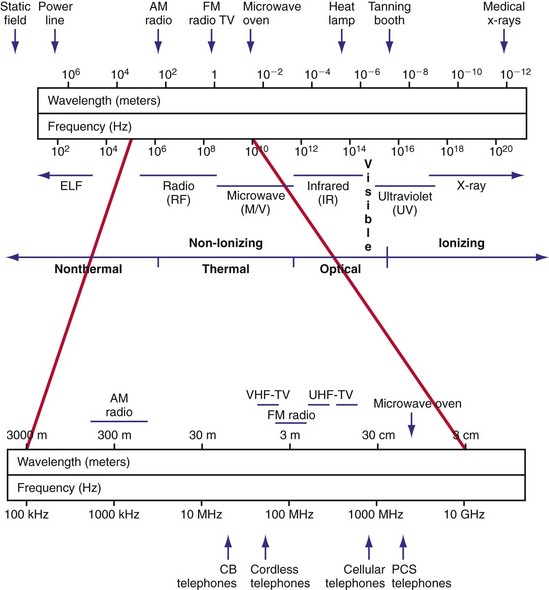
Figure 33-1 Electromagnetic spectrum.
(Modified from Moulder JP: Cellular phone antennas and health. http://www.mcw.edu/gcrc/cop/cell-phone-health-FAQ/toc.html.)
The frequency of the EMI determines the efficiency of energy coupling to the device and the resulting effect. The signal may be modulated in amplitude or frequency, and it may occur in bursts or single, long pulses. An RF carrier with amplitude modulation may induce voltages in the signal processing and detection circuitry of a CIED that can be misinterpreted as intracardiac signals.3 In other words, if the amplitude modulation has frequency components in the device’s physiologic passband, significant interference occurs. Electromagnetic fields may also cause interference with RF telemetry. Programming requires access codes to establish the telemetry link, parity checks of transmitted messages, and often simultaneous magnetic reed-switch closure by a steady magnetic field. Although the chance of modifying programmable parameters in a CIED is unlikely with current systems, integrity of the wirelessly transferred data may be affected.4
Sources of Knowledge Regarding Electromagnetic Interference
Knowledge of EMI effects on implanted devices arises from several sources. Anecdotal reports highlight the possibility of interactions but provide little information regarding overall risk. The interaction may have depended on idiosyncratic programming or device malfunction. In the United States, the Center for Devices and Radiological Health of the Food and Drug Administration (FDA) maintains a database of reported incidents of deleterious interactions (MAUDE) that is searchable online.5 However, reporting is largely voluntary, and documentation is uneven. Case reports published in peer-reviewed journals (especially if they include a re-challenge in a controlled environment) can be most valuable.
Prospective studies can be performed in vitro (i.e., bench testing) or in vivo, using laboratory animals or patient volunteers. In vitro studies are performed with the implantable device submerged in a saline-filled tank (to emulate electrical properties of tissue) and the source of radiated EMI in close proximity. A multichamber heart/trunk simulator allows more realistic in vitro testing of devices.6 Anatomically based electromagnetic models of the human body allow the use of numerical modeling to quantify the relationship between an external electromagnetic field and the voltage induced in the leads of a CIED.7 Such modeling can greatly strengthen the clinical relevance of in vitro simulation studies. These investigations allow expeditious study of interactions among various EMI sources and devices. Multiple iterations of the experiment permit examination of the effects of distance, position, field strength, and device programming on the frequency and severity of the interaction. Although simulation studies predict interference in vivo, they do not match clinical exposures identically. Discrepancies may be related to the inability to replicate the strength and path of induced body fields, differences in body position and movements, modifications from shielding effects, and specific absorption rate of the body. The orientation of the air gap between the source and the saline tank (i.e., perpendicular vs. parallel) significantly influences the distance threshold for interaction.8
In a few high-risk circumstances (e.g., MRI), in vivo testing is first conducted in laboratory animals implanted with a pacemaker system. More frequently, in vivo simulation studies require controlled patient exposure to potential sources of EMI while the cardiac rhythm is monitored. Patient exposure studies clarify the clinical significance of in vitro interactions. However, because of the time and effort involved, the number of assessed permutations is, by necessity, limited. To avoid inadvertent bias, it is important to recruit patients who are representative of the general population with implanted devices. In vivo studies are complicated by many sources of EMI also interfering with real-time or Holter electrocardiogram (ECG) recordings. Bipolar asynchronous pacing pulses that do not elicit a QRS complex are particularly difficult to ascertain. Special recording techniques are often necessary. Furthermore, real-time telemetry between the implanted device and the programmer is often compromised by EMI, even when device function remains otherwise normal. Critical review of the literature suggests that some purported instances of EMI have resulted from this inconsequential phenomenon.9 Furthermore, the programmer wand placed directly over the device can act as an artificial shield. Analysis of annotated stored electrograms (EGMs), if available, is the ideal method to evaluate device behavior during exposure to potential sources of EMI (Fig. 33-2).
Protocols for testing of implantable cardiac devices to interactions with sources of EMI were updated by the American National Standards Institute and the Association for the Advancement of Medical Instrumentation in 2007.1 This voluntary standard addresses electromagnetic compatibility of pacemakers and ICDs and provides guidance for device testing. Increased traveling, the globalization and advancement of technology in addition to a rapid increase of the number of implanted devices also warrant a global, international approach for the management of device interactions. International standards for electromagnetic compliance are issued by the International Electrotechnical Commission (IEC) and by the European Committee for Electrotechnical Standardization (CENELEC).10,11
Pacemaker and ICD Responses to Electromagnetic Interference
Pacing Inhibition
Sustained pacing inhibition can be catastrophic in pacemaker-dependent patients (Fig. 33-3). Depending on the duration of inhibition and the emergence of escape rhythms, lightheadedness, syncope, or death could result. In pacemakers, protective algorithms make prolonged inhibition uncommon. Patients who are dependent on their ICD for bradycardia pacing may be more vulnerable to prolonged pacing inhibition from EMI. In ICDs, automatic adjustment of either the gain or the sensing threshold, according to the amplitude of the intrinsic R wave, ensures sensing of low-amplitude ventricular depolarization signals during ventricular fibrillation (VF) without oversensing of T waves and extracardiac signals. In the absence of sensed complexes, two potentially life-threatening diagnoses must be considered: asystole (requiring pacing) and fine VF (requiring amplifier gain adjustments for proper detection). To ensure the detection of VF, pacing onset triggers an increase in sensitivity in most devices. These very-high-sensitivity levels can promote oversensing of extracardiac signals. Oversensing perpetuates, because the absence of spontaneous large-amplitude escape beats maintains the high operating sensitivity. Asynchronous pacing may not occur in the absence of reliable ICD noise-reversion modes. Therefore, EMI-induced prolonged inhibition and spurious tachyarrhythmia detection become likely (see later discussion). Simulation studies of the interactions between sources of EMI and ICDs require creation of a “worst-case scenario” (inducing maximum sensitivity during continuous pacing).
Triggering of Rapid or Premature Pacing
Oversensing of EMI by the atrial channel of a pacemaker or ICD programmed to a tracking mode (DDD, VDD) can trigger ventricular pacing at or near the upper tracking rate limit. Alternatively, automatic mode switching may occur if this function is enabled (Fig. 33-4). In some pacemakers, detection of noise in the atrial channel can trigger a noise-reversion mode. Preferential detection of EMI does occur, because atrial sensitivity is usually programmed higher (more sensitive) than ventricular sensitivity. It is possible to observe rapid pacing caused by atrial oversensing as a patient approaches an electromagnetic field, followed by a period of ventricular oversensing (inhibition or mode reversion) as the field becomes stronger. If sustained, inappropriate pacemaker acceleration induced by atrial oversensing can cause palpitations, hypotension, or angina.
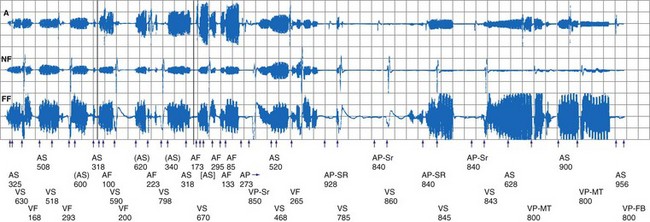
Figure 33-4 Spurious mode switch caused by atrial oversensing of EMI in patient with dual-chamber Guidant ICD.
Less frequently, EMI can induce rapid pacing through other mechanisms. For example, some sources of EMI can trigger rapid pacing (up to the sensor-triggered upper rate limit) by activating the sensor in minute ventilation (MV) pacemakers. The signal emitted by acoustomagnetic EAS systems is at the same frequency as the pulses used by some MV pacemakers to measure transthoracic impedance. MV pacemakers may also erroneously interpret the signals generated by certain monitoring and diagnostic equipment, including cardiac monitors, echocardiography equipment, apnea monitors, and respiration monitors, which also use bioelectric impedance measurements.12–14
Very strong electromagnetic fields could induce enough voltage in the lead or leads to directly capture the myocardium. For example, 58-kHz acoustomagnetic EAS systems are capable of inducing 3.7 V in pacemaker leads.15 Isolated premature paced beats (but no sustained rapid pacing) have been observed in patients. In vitro and in vivo animal studies16 have shown that application of 64-MHz RF power, required to produce MRI scans, can result in rapid pacing at pulsing periods between 200 and 1000 msec. Rapid pacing requires an intact lead connected to a pacemaker. Apparently, energy is coupled to the pacemaker defibrillation protection diodes or to the output circuit, bypassing the runaway protection mechanisms. Very rapid pacing could induce VF. Irregular rapid pacing at a rate of approximately 100 beats per minute (bpm) temporarily related to RF pulses during magnetic resonance imaging (MRI) was observed in a patient with a VVI pacemaker programmed at subthreshold output.17
Spurious Tachyarrhythmia Detection
Electromagnetic interference signals can satisfy ICD tachyarrhythmia detection criteria and lead to spurious ICD discharges (Fig. 33-5). As noted, pacemaker-dependent patients can have concomitant inhibition of pacing. In a follow-up study of 341 ICD patients who received education regarding avoidance of sources of EMI, spurious tachyarrhythmia caused by EMI occurred five times in four patients.18 The incidence was 0.75% per patient-year of follow-up. In a study of 200 chronically implanted Medtronic ICDs with detection of VF, oversensing of EMI was the cause in three patients.19 Intermittent EMI can result in shock delivery if tachycardia detection fails to terminate between the self-limited EMI episodes.
Noise-Reversion Mode
Pacemakers incorporate protective algorithms against prolonged inhibition from spurious signals (Table 33-1). A common response is transient reversion to asynchronous pacing. These algorithms are based on the fact that rapid frequencies are unlikely to represent myocardial activation. In most pacemakers, a noise-sampling or noise-interrogation window (also known as a relative refractory period) occupies the second part of the ventricular refractory period. Pacemakers do not respond to signals during the initial portion of the ventricular refractory period (i.e., ventricular blanking), which may or may not be programmable, and in some devices is adjusted automatically by the generator based on the strength and duration of the ventricular event. Signals recognized during the noise-sampling window cannot reset the lower rate timer, thus preventing inhibition, but they do affect other timing intervals, most importantly the ventricular refractory period. In some models, a noise-sampling period exists in both the atrial and the ventricular channel. Signals sensed in the noise-sampling period results in resetting of the noise-sampling window and extension of the blanking period. Repetitive triggering of the noise-sampling period eventually leads to asynchronous pacing. During simulation studies, a variable but narrow window of inappropriate pacing or inhibition is frequently observed at field or current strengths just below the reversion thresholds, because of intermittent oversensing. This phenomenon has little clinical significance during real-life EMI exposure. Occasional inhibition over a range of external field strengths is possible because EMI-induced body currents can fluctuate widely with changes in posture, respiratory phase, and other natural circumstances.20 Although transient asynchronous pacing is generally safe, it is not completely innocuous. Symptoms secondary to loss of atrioventricular (AV) synchrony and an irregular heartbeat can occur. Competition with the spontaneous rhythm can induce ventricular tachyarrhythmias if the pacing stimulus captures the ventricle during its vulnerable period. This is extremely uncommon in pacemaker patients, as attested to by the routine use of a magnet during clinic or transtelephonic pacemaker checks.
TABLE 33-1 Noise-Reversion and Electrical Reset Responses of Dual-Chamber Pacemakers Programmed DDDR
Implementation of noise-protection algorithms is more difficult in ICDs (Table 33-2). By design, these devices must be able to recognize the rapid rates of VF. Therefore, long refractory periods after sensed events are not feasible. Furthermore, asynchronous pacing is undesirable in patients who are vulnerable to reentrant ventricular arrhythmias.21 Newer Boston Scientific devices (Teligen, Cognis) utilize a Dynamic Noise Algorithm (DNA), which adjusts automatic gain control (AGC) settings in the presence of noise. Sorin devices (Ovatio, Paradym) also decrease sensitivity if noise is detected (signals sensed over 16 Hz). ICDs from Boston Scientific, Sorin, and St. Jude Medical provide programmable noise-reversion modes, but their performance against common sources of EMI is not well documented. Medtronic ICDs lack noise-reversion capabilities.
Electric (Power-On) Reset
Momentarily strong EMI, by inducing very high voltage within device circuits or triggering special microprocessor timers, may reset pacemakers and ICDs to operate in a mode different from the programmed mode. Electrosurgery and external or internal defibrillation are common causes of the reset phenomenon. In the reset mode, the pulse generator functions only with the basic factory-preset instructions (pacing mode and parameters) that are stored in the nonvolatile read-only memory (ROM), because communication between the microprocessor and the random-access memory (RAM), which contains the programmable settings, has been interrupted. Most DDD(R) pacemakers reset to the VVI mode (see Table 33-1). The reset mode does not revert back when EMI is discontinued. Resolution of the problem requires a specific programmer command. In some pulse generators, there is no response to magnet application in the reset mode. In some pacemakers, the pacing mode and rate are similar during electrical reset and elective replacement indicator (ERI). In other pacemakers (e.g., older St. Jude Medical devices), strong EMI can trigger a reset mode or ERI. In those cases, clearing the ERI message with the programmer restores normal function. In Medtronic pacemakers, two levels of electrical reset exist: partial and full. Partial reset tends to occur with less intense interference, preserving the programmed pacing mode and rates. Electrical reset can be differentiated from battery depletion by telemetric assessment of battery voltage and impedance. If reset was caused by EMI, the battery voltage should be normal (~2.8 V), and the battery impedance should be either normal or slightly raised according to battery age.
In ICDs, electrical reset generally results in a “shock box” configuration, with VVI pacing and maximum energy shocks (see Table 33-2). A special type of reset (called “hard reset” by St. Jude Medical, “cold reset” or “safety core mode” by Boston Scientific) can occur if there is damage to the ICD microprocessor or memory (e.g., from radiotherapy). This type of reset is irreversible and cannot be reverted by a programmer command. Prompt generator replacement is usually required.
Closure of the Reed Switch
Most pacemakers and some ICDs contain a magnetic reed switch that is closed by a 7 mT (Boston Scientific) to 1 mT (Medtronic) static magnetic field. This generally results in temporary asynchronous pacing in pacemakers and temporary suspension of tachyarrhythmia detection and therapy in most ICDs. Normal function returns as soon as the magnetic field dissipates. Older Guidant ICDs were deactivated by continuous application of a magnetic field for 30 seconds or longer. Reactivation required reapplication of the magnet for 30 seconds or longer or a programmer command. Guidant ICDs have been inadvertently deactivated by items that generate inconspicuous strong magnetic fields, such as magnetized screws,22 stereo speakers,22 and bingo wands,23 as well as by unadvised magnet application in health care settings.24 In later devices, this function was programmable and nominally disabled, and it is no longer available in the latest generation of Boston Scientific ICDs. In most contemporary ICDs, magnetic reed switch and its function has been largely replaced by other technologies (integrated solid-state detection, Boston Scientific; Hall effect sensor, Medtronic; telemetry coil, Sorin Group; GMR circuit, St. Jude Medical).
Transient suspension of antitachycardia pacing caused by reed-switch closure had been shown to occur frequently with older-generation ICDs. In a study of 46 patients with St. Jude ICDs (which keep a log of magnet reversions), nine unexplained inactivations occurred outside the medical environment (10% per patient-year of follow-up).25
Response to magnet application is also programmable in various devices to trigger specific behaviors, including storage of EGMs and event markers or replay of alert tones. Exposure to a strong magnetic field when these functions are activated can result in eccentric (but clinically inconsequential) device behavior.26 However, inadvertent asystole can occur when magnet application does not result in asynchronous pacing in pacemakers during electrosurgery.
Damage to Generator or Electrode-Myocardium Interface
Current devices incorporate elements (Zener diodes, thyristors) that protect the pacing output circuitry and sensing amplifiers by shunting excess energy away from the device. The Zener diode behaves as a short circuit as soon as the voltage exceeds a certain value, such as 10 to 15 V, that is substantially above the output voltage of the pulse generator. Other circuit designs can also limit the current flowing up the lead, but at the expense of inhibition of pacing output during the reception of large voltages.27
Interactions with Telemetry Function
Communication between the CIED and programmer is achieved by RF transmission via a wand. Once the “handshake” is complete and connection established with the programmer, further communication may be accomplished via wireless communication with certain devices. Wireless communication has also been increasingly used for home monitoring of device function and clinical status.28,29 Communication may be inhibited or greatly degraded if interference from external sources is present. These interactions may occur during device testing in the OR or clinic as well as potentially in the home.
Determinants of Electromagnetic Interference
The effects of EMI on pacemakers and ICDs depend on the intensity of the electromagnetic field, the frequency spectrum of the signal, the distance and positioning (angle) of the device relative to the source, the electrode configuration (unipolar or bipolar), nonprogrammable device characteristics, programmed settings, and patient characteristics (Box 33-2).
Electrical and magnetic fields decrease inversely with the square of the distance from the source. Devices from different manufacturers differ in susceptibility to various sources of EMI, depending on circuitry design. EMI from digital cellular telephones has largely been suppressed by incorporation of simple RF feedthrough filters to the circuitry. Interference is most likely when the antenna is placed over the device header. Neither the sensing electrodes near the distal tips of the leads, nor the coated lead body, is susceptible. A higher-programmed sensitivity level increases device susceptibility to EMI. Unipolar pacemakers are more vulnerable to EMI from sources in the lower range of the frequency spectrum, such as power lines.30 Left-sided unipolar CIEDs are particularly susceptible because of the larger loop for voltage induction between the lead and the generator. Sensing configuration loses importance at longer radiation wavelengths.
Sources of Electromagnetic Interference
Daily Life
Cellular Telephones, Wireless Communication Devices, Personal Media Players
By the end of 2010, subscribers to mobile cellular phone services are expected to reach 5 billion worldwide. Although cell phones continue to be the most popular wireless communications devices, personal digital assistants (PDAs), laptop computers, wireless Internet connections, portable digital media players, and other appliances are being increasingly used for wireless voice, data, music, and video transmission. Assessment of the effects of cell phones on CIEDs has been complicated by the wide variety of technologies in use.31 Almost all current wireless telephones operate with digital technologies, although many can fall back to analog operation if their digital mode is not available. The Global System for Mobile (GSM) communications has become the predominant digital platform worldwide. Other digital technologies in use in the United States and elsewhere include North American Digital Cellular (NADC), also known as Time Division Multiple Access (TDMA-50 Hz); Code Division Multiple Access (CDMA); integrated Dispatch Enhanced Network (iDEN), a cell phone system that also serves as a walkie-talkie platform; and Personal Communication System (PCS). New third- and fourth-generation (3-G, 4-G) networks with increased speed for data transmission are being deployed. Wireless networks operate in the 800- to 960-MHz or the 1.8- to 2.5-GHz band. The power level used by a wireless telephone (and the consequent emitted electromagnetic field) fluctuates throughout the call, according to distance from the base station and the number of devices being used on the system at the same time. The maximal power of handheld phones is limited to 0.6 W in the United States and 2 W in Europe. Vehicle-mounted units can transmit at higher powers (up to 8 W), but they are not in common use by the general public.
Although isolated case reports have suggested the potential for severe interactions,32 most research indicates that deleterious interactions are unlikely to happen with normal cell phone use. Large-scale bench-testing studies of the effects of wireless telephones on pacemakers and ICDs have been conducted at the FDA’s Center for Devices and Radiological Health,33,34 the Medical Devices Bureau of Canada,35 and the University of Oklahoma’s Wireless Electromagnetic Compatibility Center.36,37 These studies encompassed several thousands of runs of telephone-device combinations and provided consistent results. Interference was nonexistent with the now-outdated analog telephones. The pulsed component of the transmission in digital cell phones was detectable by pacemaker sensing circuitry if the field was strong enough. PCS and similar technologies produced interactions in less than 1% of tests, whereas other digital technologies (GSM, TDMA) produced interference in 0% to 25% of tests. In all studies, just a few models were responsible for a disproportionately large number of interactions, and other models were largely immune. An older version of TDMA-11 technology (used only for specialized business applications such as trucking, delivery, and construction in the United States) accounted for most interactions with ICDs. Titanium casing and introduction of feedthrough filters, now common in modern pacemakers, made devices virtually immune to interference. Almost all interactions occurred at distances of less than 10 cm. Devices always reverted to normal operation when the phone was turned off.
Systematic investigations of the effects of cell phones in patients with pacemakers and ICDs have documented that severe interactions are improbable with most technologies during regular phone use. In a comprehensive multicenter study, Hayes et al.38 tested 980 patients with implanted pacemakers for potential interference with five types of telephones (one analog and four digital: NADC, TDMA-11, PCS, and CDMA). Telephones were tested in a simulated worst-case scenario; in addition, NADC telephones were tested during transmission to simulate actual use. Patients were monitored while the phones were held at the ipsilateral ear and in a series of maneuvers directly over the pacemaker. The incidence of any type of interference was 20% in the 5533 tests. Tracking of interference sensed in the atrial channel, asynchronous pacing, and ventricular inhibition were the most common reactions observed (14%, 7%, and 6%, respectively). Interference was least frequent with analog (2.5%) and PCS (1.2%) systems. Clinically significant EMI was observed in 7% of tests and was considered severe in 1.7%. There were no clinically significant EMI episodes when the telephone was placed in the normal position over the ear. The presence of feedthrough filters in the pacemakers almost abolished the risk of EMI (from 29% to 56% down to <1%).
In a study of 39 pacemakers, EMI was more common with portable 8-W GSM telephones than with handheld 2-W models (7% vs. 3% of tests); oversensing was more frequent at maximal than at nominal sensitivity (6% vs. 2% of tests).39 In a recent study of 100 patients with pacemakers from five manufacturers equipped with feedthrough filters testing a GSM cell phone with maximum power of 2 W in the standby, dialing, and operating mode, only two instances of ventricular inhibition were observed with the phone held directly over the pocket and the ventricular sensitivity programmed unipolar at 0.5 mV or less. Reprogramming of the sensitivity to 1 mV abolished the interaction in both cases.40 In another European study, 158 patients with pacemakers from one of seven manufacturers were tested for EMI using a GSM and PCS phone (max power 2 W vs. 1 W, operating frequency 900 MHz vs. 1800 MHz, respectively).41 The phones were placed over the device (as if carried in breast pocket), and EMI was evaluated while the device received a call (highest power emission). Device interaction was very rare and only occurred in four older devices with less advanced filters.
The GSM telephones did not induce inappropriate rapid pacing in patients with MV pacemakers42 or atrial oversensing in single-lead VDD pacemakers programmed at maximum atrial sensitivity.43 In a study of 95 children with a variety of pacemakers programmed to a worst-case scenario, GSM telephones did not induce significant interference.44 Clinical worst-case scenario testing has not disclosed significant interactions between ICDs and wireless digital telephones.44–47 Digital cell phones do not interfere with the detection of induced VF in the electrophysiology laboratory.47 Inconsequential intermittent loss of telemetered EGMs and surface ECGs and inscription of erroneous event markers (i.e., “pseudo-oversensing”) recorded via the programmer are common. Although this may have limited significance during a clinic visit, implications may be different if interaction occurred during the use of a remote home monitoring system. Effect of GSM phones were tested in vitro (three-chamber heart stimulator) as well as in vivo in a study to evaluate possible interactions with Biotronik pacemaker/ICD home monitoring system. A proprietary telemetry is used in these devices transmitting in the 402 to 405–MHz band with maximum power of 25 µW to communicate with the base unit within a 10-foot (3-m) distance. The base unit in turn transmits data via GSM cellular network to the monitoring center. No interactions were observed during in vitro testing, and there was 93% success in transmissions in vivo.48 Delay or loss of transmission was seen as result of EMI. Similar home monitoring systems are also available from several other manufacturers.
Portable media players, especially iPODs (Apple, Cupertino, Calif.) have been increasingly used (>270 million units sold worldwide). Because these units are frequently carried in the shirt pocket and close to the CIED, several studies addressed possible device interactions. While reproducible programmer telemetry interactions were common, these were clinically nonsignificant, and no other serious interaction was seen with modern pacemakers.49–52 Clinically significant device interaction caused by strong magnetic field may occur, however, if portable headphones are held close to a CIED.53 Telemetry interactions may have important implications in the use of home monitoring systems, and these need to be addressed in the future.
Although cell phones can potentially interfere with the function of implanted devices, this interference does not pose a health risk when telephones are placed over the ear. Maintaining an activated cell phone at least 6 inches (15 cm) from the device prevents interactions. The FDA has issued simple recommendations to minimize the risks. Patients should avoid carrying their activated cell phone or iPOD in a breast or shirt pocket overlying a CIED. A wireless telephone in use should be held to the ear opposite the side where the device is implanted. A survey of 1567 Japanese patients with implanted pacemakers revealed that, although 94% were right-handed, 41% used the left hand preferentially to hold a wireless telephone.54 Not-so-obvious reasons for choosing one hand versus the other to hold the phone included hearing loss on one side (10%) and use of the opposite hand for dialing or writing memos (22%). At least in some patients, apparently the hand preferentially used to hold the wireless telephone should be considered when selecting the site for pacemaker implantation.
Limited in vitro and in vivo testing has suggested that 3-W GSM telephones do not interfere with the function of an implantable ECG loop recorder.55 A single case report suggested interference between an ILR and the handheld activator if an iPOD is operating in proximity to the devices.56
In an in vitro study, PDAs connected to a hospital wireless local area network (WLAN) using the 802.11b protocol did not interfere with a variety of pacemakers and ICDs manufactured by Guidant, Medtronic, or St. Jude Medical.57
Electronic Article Surveillance Devices
Also known as antitheft devices or “antishoplifting gates,” EAS devices are ubiquitous in retail stores, libraries, and office buildings. More than 1 million systems have been installed worldwide. The transmitter in these devices emits an electromagnetic field that is designed to interact with a “tag” in an unpurchased item. As a result of the interaction, the tag emits a signal that is detected by the receiver. Customers are exposed to an electromagnetic field as they walk through the gate, which typically consists of a pair of transmitter and receiver pedestals. EAS systems differ greatly in the frequency and strength of their emitted fields. Available technologies include high-frequency systems (operating beyond 900 MHz), swept RF systems (operating at 2 to 10 MHz), low-frequency acoustomagnetic systems (30-132 kHz), and electromagnetic systems (20 Hz to 18 kHz). These technologies serve different retailers’ needs in terms of area covered, cost, detection, and “false alarm” rate, and they are not strictly interchangeable. The general consumer cannot differentiate these systems by their external appearance. Electromagnetic fields from these devices have the potential to induce interference signals in the sensing circuit of implanted cardiac devices. A sentinel report described a patient with complete heart block and a Ventak (CPI/Guidant) AV ICD in an abdominal pocket who developed multiple shocks and near-fatal inhibition of pacing on exposure to an acoustomagnetic EAS system.58 Provocative testing with similar equipment in a controlled environment reproduced the interaction. The maximum distance at which ventricular oversensing occurred was 30 cm. When sensitivity was reprogrammed from “nominal” to “least sensitive,” the interaction occurred only at closer proximity.
Prospective studies have clarified the incidence, severity, and risk factors for EMI from EAS systems. An in vitro study showed that 20 of 21 pacemaker models reacted to the field of an acoustomagnetic EAS system, and 10 reacted to an electromagnetic system.59 Responses included inhibition and noise reversion. Interference occurred when the simulator was within 33 cm of the transmission panel for the acoustomagnetic system, or 18 cm for the electromagnetic system. Mugica et al.60 exposed 204 patients with pacemakers to two different EAS systems (acoustomagnetic at 58 kHz and electromagnetic at 73 Hz) for up to 30 seconds. Interference occurred in 17% of patients and was twice as likely with the acoustomagnetic system. Atrial tracking, asynchronous pacing, and single-beat inhibition were observed. All the interactions were transient and deemed benign.
McIvor et al.15 studied the effects of six EAS devices of three types (magnetic audiofrequency, swept radiofrequency, acoustomagnetic) in 50 patients with pacemakers and 25 patients with ICDs from seven different manufacturers. One exposure protocol mimicked the most common real-life situation, walking at a normal pace midway between the gates. A “worst-case scenario” protocol required the patients to lean against the transmitter gate with the body parallel and then perpendicular to the transmitter. Interactions occurred with 48 pacemakers, almost exclusively with acoustomagnetic systems. No pacemaker reacted to the swept RF systems. Only two patients had transient asynchronous pacing while exposed to an electromagnetic system. The frequency of interactions with the acoustomagnetic system increased with the duration and closeness of the exposure: 16% when walking through the gates and 96% when leaning against the pedestal. Transient asynchronous pacing was the most common response, followed by atrial oversensing with tracking, ventricular oversensing with inhibition, and “voltage-induced” paced beats. Changing the sensing configuration from unipolar to bipolar, or programming a lower sensitivity setting, did not abolish the interactions but limited them to closer distances from the center of the gate. There were no instances of false tachyarrhythmia detection, but the ICDs were not programmed to pace during the testing.
Groh et al.61 studied the interaction between ICDs and two electromagnetic and one acoustomagnetic EAS devices in 169 patients. No spurious detections occurred during a 10- to 15-second walk through the gates. False VF detection occurred in three patients during a 2-minute exposure to the acoustomagnetic system. When the 2-minute exposure was repeated during continuous pacing in 126 patients, oversensing was observed in 19 (15%). Oversensing was severe (complete or prolonged pacing inhibition) in 7 patients (6%), including 3 patients who had had spurious tachyarrhythmia detection at baseline and 4 additional patients with Ventritex ICDs who had oversensing during exposure to an electromagnetic system. All the patients with serious interactions had an abdominal implant; however, by multivariate analysis, diminished R-wave amplitude and a Ventritex ICD were the only predictors of interactions.
In summary, severe interactions between EAS systems and CIEDs are unlikely when patients walk through the gates at a normal pace. On the other hand, dangerous interactions are likely with prolonged, close exposure to acoustomagnetic or electromagnetic systems. Patients should be instructed not to linger in proximity or lean against theft-deterrent gates. Retailers should avoid placing systems where people are required to linger, such as at checkout counters. Merchandise or information (e.g., store floor plans) should not be displayed close to antitheft systems. The FDA recommends that all manufacturers of electronic antitheft systems develop labeling or signage to post on or near all new and currently installed systems, indicating that an electronic antitheft system is in use. The labeling or signage should be positioned so that it is visible before an individual enters the monitored area.62
Metal Detectors
Handheld and walk-through metal detectors are used for security applications. They function by sensing disturbances in electromagnetic fields. Handheld metal detectors typically operate at a frequency of 10 to 100 kHz and produce weak fields (≤4 A/m at a distance of 1 inch). Weapons are detected only within 1 to 4 inches. Walk-through metal detectors have coils on one or both sides of the equipment. They operate in a continuous wave (5-10 kHz) or in pulsed mode (200-400 Hz). Magnetic fields measured at the chest level inside the arch are less than 2 G.63 Typically, a person walking through is exposed for 3 seconds. Kolb et al.64 monitored 200 patients with pacemakers and 148 patients with ICDs from a variety of manufacturers for interactions with a standard airport metal detector gate. Pacemakers were reprogrammed to force ventricular pacing and ICDs to maximum sensitivity. Testing included normal walking through the gate and a worst-case scenario in which the chest was as close as possible to the gate and a 360-degree torsion was performed around the body axis. There were no interactions with any system.
The FDA has received one report of a spurious ICD shock triggered by a handheld metal detector in an airport. In several other instances, older-model Guidant ICDs reverted to “monitor-only” mode after being exposed to metal detectors.5 Current FDA recommendations state that it is safe for patients with implanted cardiac devices to walk through a metal detector gate, although the alarm may be triggered by the generator case. If scanning with a handheld metal detector is needed, patients should ask the security personnel not to hold the detector close to the implanted device longer than is absolutely necessary. A manual personal search can also be requested.65
Electric Power
Detrimental effects from incidental exposure to high-voltage lines are unlikely. Even at a distance of 40 m from a 400-kV line, the electric and magnetic field strength is very low. Numerical studies suggested that the thresholds for EMI from magnetic fields at power line frequencies under the worst-case scenario for unipolar pacemakers are 40 µT in the atrium and 140 µT in the ventricle.66 In vivo studies disclosed that all types of response (inhibition, triggering, noise reversion) could occur, depending on the strength of the field, the generator model, the sensing configuration, and the programmed sensitivity.30 Trigano et al.67 exposed 250 pacemaker patients to a 50-Hz magnetic field with a flux density of 100 µT (the maximum allowed public exposure by European activities). There were no effects in devices programmed to bipolar sensing. Three patients with unipolar devices had noise-reversion to asynchronous mode; in one, symptomatic pacing inhibition followed. The studies suggest that bipolar sensing protects from EMI in all but the most extreme environmental conditions, such as power-generating stations. With unipolar sensing, inappropriate pacemaker behavior can occur during routine daily exposures.
The EMI from household appliances results almost exclusively from improper grounding. Washing machines appear to be a frequent offender.68–70 Anecdotal reports have incriminated slot machines,71 power drills operated in a wet environment,70,72 a current leak from a water boiler (occurring when the hot-water faucet was opened),73 and vibrators.18 A patient with a normally functioning ICD received spurious shocks caused by 60-Hz interference while entering and exiting a public swimming pool; the current leak was otherwise undetectable.74 Household induction ovens are safe in patients with pacemakers75 or ICDs.76
Two case reports describing patients with spurious ICD discharges from low-level alternating-current leak are especially illuminating. In a man with spurious ICD discharges caused by use of an electric razor, provocative testing confirmed oversensing of 50-Hz power with the patient’s razor and a new similar unit. At operative revision, an insulation break was discovered at the ventricular coil of the “integrated” bipolar Endotak (Guidant) lead.77 A boy with a single-chamber Medtronic ICD with an “integrated” bipolar Sprint lead had spurious detection of VF caused by oversensing of 60-Hz current while swimming in a pool and taking a shower powered by an electrical generator. The proximal and distal coils had been inverted in the device header. Because of the hardwiring in the lead and device header, the generator may become part of the sensing circuit.78 Both systems were operating “de facto” in a unipolar sensing mode.
Neuromuscular incapacitation devices have been increasingly used by law enforcement officers and by the general public. The Taser device (Taser International, Scottsdale, Ariz.) shoots darts that are wired to the device and deliver up to 50,000 V over 5 seconds to the victim, causing painful tetany. Oversensing with spurious detection of VF has been described after application of a shot from a Taser device.79 Lakkireddy et al.80 analyzed the effects of Taser shots in animals implanted with an ICD. No permanent device damage was seen, but oversensing was common with Taser application.
Alternative Medicine Devices
A variety of “therapeutic magnets” are commercially available for the treatment of arthritis and other musculoskeletal ailments. Despite manufacturers’ claims of very strong magnetic field strengths (up to 30,000 G), in vitro testing showed that the magnets were able to close the reed switch only when placed at a distance less than 1 inch from the generator.81 Spurious ICD shocks have been reported with unsupervised use of popular battery-operated muscular stimulators for abdominal training.82 Acupuncture entailing delivery of current to needles inserted in the anterior chest has triggered spurious ICD shocks.83 The “Zapper” is a battery-powered alternative medicine device that delivers a square-wave output at a constant frequency of 33.3 kHz.84 These electronic pulse frequencies are applied to both hands. It is marketed to enhance immune function and eliminate chronic illnesses, parasites, and germs. Vendors advise against the use of these devices in patients with pacemakers. Symptomatic pacemaker inhibition from oversensing has been documented in a patient with heart block during use of the Zapper.84
Working Environment
Industrial Equipment
The return of the CIED patient to a work environment suspected of high-level EMI can be challenging. Among the myriad potential EMI sources, arc or spot welders, industrial welding machines, degaussing coils, and electrical motors are frequent causes of concern. Not only do these sources emit energy in the RF spectrum, but their associated magnetic fields could potentially cause magnet response in pacemakers and ICDs. Static magnetic fields strong enough to close the reed switch are unlikely to be present in industrial environments. For example, in a petroleum refinery, peak fields of almost 2 mT were measured close to large compressors and in power distribution centers. However, the fields dropped off to less than 0.1 mT at a distance of 4 feet.85 High levels of electromagnetic radiation exist in the cockpits of general aviation aircraft. However, in vitro testing of five modern pacemakers programmed in a unipolar configuration during flight conditions in single-engine fixed-wing aircraft did not demonstrate EMI.86 There are no current guidelines regarding certification of air pilots with implanted cardiac rhythm management devices.